Polymerization of 10,16-Dihydroxyhexadecanoic Acid, Main Monomer of Tomato Cuticle, Using the Lewis Acidic Ionic Liquid Choline Chloride·2ZnCl2
- 1Instituto Politécnico Nacional, Centro de Nanociencias y Micro y Nanotecnologías, Mexico City, Mexico
- 2Departamento de Procesos y Tecnología, DCNI, Universidad Autónoma Metropolitana, Unidad Cuajimalpa, Mexico City, Mexico
- 3Departamento de Ingenierías Química y Bioquímica, Instituto Tecnológico de Zacatepec, Zacatepec de Hidalgo, Mexico
10,16-Dihydroxyhexadecanoic acid, main monomer of the tomato cuticle obtained from agroresidual wastes, was polymerized using ionic liquid (choline chloride·2ZnCl2) as catalyst at three different temperatures (80, 90, and 100°C). The resulting polyesters obtained under these conditions were insoluble in most of the organic solvents and showed different physicochemical properties. While at 80°C, polymers were obtained as powder, and at higher temperature, they were found in viscous consistency. According to the cross polarization magic angle spinning nuclear magnetic resonance and Fourier transform infrared spectroscopy attenuated total reflectance analysis, polymers showed a linear structure with an increasing degree of esterification in position C-10. Polyesters were analyzed by means of differential scanning calorimetry, atomic force microscopy, and X-ray diffraction (small- and wide-angle scattering) techniques.
Introduction
Biodegradable polymers belong to one of the most attractive biomaterials because of their wide applications in biomedical fields. Most of the research on renewable and biodegradable materials based on polyesters has been concentrated on polylactide (PLA), polyglycolide (PGA), and their copolymer poly(lactide-co-glycolide) (PLGA), which are the most commonly used and extensively studied biodegradable polymers because of their good biocompatibility, low immunogenicity, and satisfactory mechanical properties (Albertsson and Varma, 2002; Chen and Wu, 2005; Nair and Laurencin, 2007; Philip et al., 2007). Although the application-relevant property profile of any polymeric material depends on numerous properties, one significant disadvantage of these aliphatic polyesters is their lower melting points (Yamamoto et al., 2002). Apart from the very short-chain aliphatic polyesters, melting points of polyesters based on common aliphatic monomers are too low for thermoplastic processing. Linear polyesters do not have strong interacting groups, so, the predominant factor affecting melting temperature is the number of conformations available in the molten state, in other words chain flexibility. Higher flexibility leads to higher entropy of melting and lower melting temperature. For example, Tm of poly(alkylene adipates)s is different for poly(alkylene terephthalate)s, an aliphatic and aromatic polyesters (Tm 50 and 265°C, respectively). It has been demonstrated that the presence of functionalizable groups, such as hydroxyl and carboxylate, in polyesters can efficiently increase their hydrophilicity and degradability, plus modulate their mechanical, thermal, chemical, and biological properties (Lecomte et al., 2006; Parrish and Emrick, 2006; Williams, 2007). Within the new group of degradable polymers, various types of aliphatic polyesters films have been described (Bayer et al., 2004; Benitez et al., 2004; Olsson et al., 2007; Heredia-Guerrero et al., 2009; Quinzler and Mecking, 2010; Stempfle et al., 2014; Benítez et al., 2015). Between them, successful polycondensation reactions have been achieved using titanium alkoxides as catalyst to afford the corresponding long-chain aliphatic polyesters (Quinzler and Mecking, 2010; Stempfle et al., 2014).
Even when long-chain polyhydroxyesters (C16–C20) are present in nature in the form of the plant biopolymer cutin, its monomeric complexity makes difficult to get and use it as natural polyester for commercial source of “green” chemicals (Olsson et al., 2007).
We were able to isolate the main monomer of the tomato cutin, the 10,16-dihydroxyhexadecanoic acid (10,16-DHPA), from agroresidual wastes (Arrieta-Baez et al., 2011). These residuals represent approximately 20% of the crop losses and are a good source of this aliphatic long-chain acid, which could be used to get other monomers. 10,16-DHPA was successfully polymerized with Candida antarctica lipase-B (CAL-B) to get polyesters with Mw = 814 and Mn = 1,206 Da (Gómez-Patiño et al., 2013). The most prominent synthetic aliphatic polyesters are usually prepared by enzymatic ring-opening polymerization (ROP) of the respective cyclic monomers. This method provides sufficient polymerization control, resulting in polymers of the required molar masses and with the desired end groups. However, it has been very difficult to prepare aliphatic long-chain polyesters by direct polycondensation because of their thermal degradation at high temperature and low hydrolytic stability in one step (Akinori et al., 2003).
Most of the polymerization reactions are carried out in organic solvents of which some eventually end up polluting environment by evaporation or leakage. Recently, there have been intense research efforts to replace conventional organic solvents with novel solvent systems, which are recyclable and environmentally compatible and therefore reduce waste and hazard. Ionic liquids (ILs; Seddon, 1997; Welton, 1999) and other non-conventional solvent systems, such as choline chloride/oxalic acids (Abbott et al., 2004) and ZnCl2, have drawn attention to the synthetic organic and polymer chemists worldwide, since they offer a potentially clean method to carry out chemical reactions or processes. They are non-volatile, while liquids over a wide range of temperatures, and offer benign alternatives to traditional organic solvents.
However, it is still a challenging subject to seek for other excellent catalysts in order to achieve in one-step synthesis long-chain aliphatic polyesters under milder conditions (lower temperature and short time). Polycondensations under mild conditions are very important from an environmental technology view point (Shu et al., 1994; Quinzler and Mecking, 2010; Stempfle et al., 2014).
The present work describes the synthesis of aliphatic polyesters from 10,16-hydroxyhexadecanoic acid obtained from agroresidual tomato wastes using an IL, such as choline chloride·2ZnCl2, as a potent catalyst in the synthetic method for the synthesis of long-chain wax esters. These aliphatic polyesters obtained at different temperatures and times showed particular physicochemical characteristics. Although it has been reported that enzymatic lipase-mediated reactions give soluble oligomeric products (Gómez-Patiño et al., 2013), reactions with choline chloride yield insoluble polymers that were characterized by cross polarization magic angle spinning nuclear magnetic resonance (CPMAS 13C NMR), Fourier transform infrared spectroscopy (FT-IR), and differential scanning calorimetry (DCS).
Materials and Methods
Materials
Choline chloride, zinc chloride·toluene, hexane, dimethylformamide (DMF), and 2-methyl-2-butanol (2M2B) of reagent grade were purchased from Merck (Naucalpan de Juarez, Edo. de Mexico, Mexico) and used without further purification.
Isolation of 10,16-Dihydroxyhexadecanoic Acid (10,16-DHPA 1)
10,16-DHPA (1) was obtained by depolymerization of tomato cutin as described elsewhere (Osman et al., 1995, 1999). Identification of the monomer was done by nuclear magnetic resonance (NMR) spectrometry and mass spectrometry (MS) analysis, and the data were compared with those previously reported (Ahmed et al., 2003; Quinzler and Mecking, 2010).
10,16-DHPA (1). Yellow pale powder. 1H-NMR (500 MHz, CH3OD) δ ppm 3.63 (m, 4H) C(OH)H-10 and C(OH)H2-16, 2.27 (t, J = 7.52 Hz, 2H) CH2-2, 1.60–1.22 (m) CH2-3-9 and CH2-11-15. 13C-NMR (CH3OD) δ 177.68 (CO); 72.40 (C-10); 63.00 (C-16). EI-MS (m/z): 289 [M + H]+, 271 [M-H2O]+, 253 [M-2H2O]+, 130 [C8H18O2-OH]+ (Quinzler and Mecking, 2010).
Preparation of Choline Chloride·2ZnCl2
Choline chloride (20 mmol) was mixed with zinc chloride (40 mmol) and heated to 150°C with stirring until a clear colorless liquid was obtained (Sunitha et al., 2007).
General Procedure for Polyesterification Reaction
Polyesterification reactions were carried out under low vacuum reflux with two-necked round-bottom flask equipped with a magnetic stirrer, thermometer and fitted with a Dean Stark adapter and a condenser for reflux. These reactions were carried out at three different temperatures (80, 90, and 100°C) with different reaction times (2, 4, 6, 12, and 24 h) using IL, choline chloride·2ZnCl2 as a catalyst. IL catalyst was used at molar ratio 1:1 to the reactant. Polymers were precipitated with cold methanol, extracted, and characterized by NMR, FT-IR, and DSC.
Methods
NMR Spectroscopy
Soluble products were characterized by 1H- and 13C-NMR (Varian NMR System, 500 MHz; Palo Alto, CA, USA). The NMR spectra were recorded in deuterated chloroform (CDCl3) or methanol (CH3OD).
Insoluble polymers were analyzed using standard CPMAS 13C NMR experiments carried out on a Varian Instruments Unityplus 300 widebore spectrometer (Palo Alto, CA, USA) equipped for solid-state NMR. The resonance frequency was 74.443 MHz, with a customary acquisition time of 30 ms, a delay time of 2 s between successive acquisitions, and a cross polarization (CP) contact time of 1.5 ms. Typically, each 30-mg sample was packed into a 5-mm rotor and supersonic magical angle spinning (MAS) probe from Doty Scientific (Columbia, SC, USA), then spun at 6.00 (0.1 kHz and room temperature for approximately 10 h. No spinning sidebands were observed up- or downfield from the major carbonyl or aliphatic carbon peaks. The resulting data were processed with 50 Hz of exponential line broadening.
ATR FT-IR Spectroscopy
Attenuated total reflectance ATR) FT-IR spectra were recorded with a BOMEM 157 FTIR spectrometer equipped with a deuterated triglycine sulfate (DTGS) detector. The instrument was under continuous dry air purge to eliminate atmospheric water vapor. The spectra were recorded in the region of 4000–400 cm−1.
AFM Analysis
The atomic force microscopy (AFM) image was obtained fixing the samples on a metallic disk with double face tape, using a MultiMode AFM V (Bruker, Santa Barbara, CA, USA) in air with a RTESP cantilever, the size of each image was 5 × 5 μm2 in taping mode. The roughness parameters Rq and Ra were determined using the expressions and , where Rq is the root mean square average of the height deviations, Ra is the arithmetic average of the absolute values of the surface height deviations, Z is the height value, and N is the number of the number of the data, these parameters were obtain using the nanoscope analysis image Software.
X-Ray Diffraction (Small- and Wide-Angle Scattering) Study
The X-ray patterns were measured from 1.5° to 30°. It is possible to do SAXS and WAXS in a single run [small- and wide-angle scattering (SWAXS)] with the equipment used. X Pert PRO MRD Diffractometer from PANalytical in Bragg-Brentano geometry was used with Cu radiation (Kα = 1.54 Å) at 45 kV and 40 mA tube power. The step size was 0.03 and 500 s for each step. X-ray mirror with 1/32 divergence slit and Soller slit (0.04 rad) were used in incident optic for parallel beam and measurements in small angle. PIXcel ultrafast X-ray detector for diffracted optic was used.
DSC Analyses
Differential scanning calorimetry was performed with a TA Instrument 2010 Modulated DSC (New Castle, DE, USA). Sample of about 10 mg was encapsulated in aluminum pan and heated from 30 to 220°C at a heating rate of 5°C min−1 under 50 mL min−1 nitrogen purge.
Results and Discussion
Polymerization Reaction
Polyesters (Scheme 1) derived from 10,16-DHPA (1) were synthesized via IL, using choline chloride·2ZnCl2 as a catalyst. When compared with enzymatic reactions as previously reported (Gómez-Patiño et al., 2013), the obtained polyesters were completely different, they were waxes, with ILs were powders and materials with viscous consistence.
After reaction, polyesters were precipitated with methanol and separated from the IL. Subsequently, they were washed with water, methanol, and chloroform and dried to characterize them by NMR, FT-IR, and DSC techniques. The IL was subject to an extraction with ethyl acetate to recover the unreacted monomer and after that was heated at 150°C to evaporate remained solvents. After this, the IL was used at least four times without any significant loss of activity. The synthesis data are shown in Table 1.
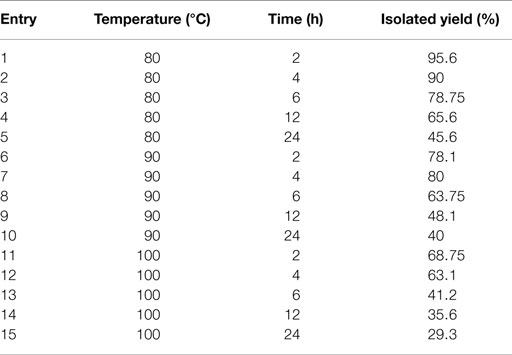
Table 1. The result of synthesis of 10,16-DHPA under different temperatures at different times in choline chloride·2ZnCl2.
Polyesters obtained were insoluble in chloroform, methanol, water, DMSO, and DMF, which made them difficult to analyze by means of MS in order to get their molecular weight.
Characterization of the Polymers
Polymers obtained from IL, choline chloride·2ZnCl2, at different temperatures and different times showed different physical characteristics (Figure 1). Polymers obtained in reactions at 80°C were mainly powder; those obtained at 90°C were solid whiles those obtained at 100°C were essentially viscous, completely different from those obtained from enzymatic reactions, which were like waxes (Gómez-Patiño et al., 2013). However, all of them were insoluble in most of the solvents.
ATR-FT-IR Spectroscopy
We have found that polyesters obtained from enzymatic reactions are mainly linear (Gómez-Patiño et al., 2013). Polyesters obtained from CAL-B with 10,16-DHPA (Mw = 982 and Mn = 860 Da) showed a band at 3365 cm−1 attributed to the presence of residual OH and an intense band at 1738 cm−1 attributed to C = O stretching in ester groups, and the asymmetric stretching vibration of the C–CO–O at 1172 cm−1 which confirm the presence of a linear polyester group (Gómez-Patiño et al., 2013). For the polymers obtained in choline chloride·2ZnCl2, the IR spectra (Figures 2A,B) spectra are very similar, and the majority of the bands correspond to an ester functional group stated by the νC = O around 1735 cm−1 and the (OC–O–C) stretching at 1177 and 1247 cm−1 (Quinzler and Mecking, 2010; Gómez-Patiño et al., 2013) and the weak contribution of the free acid IR peak around 1700 cm−1, that was not present, confirm that polymers are mainly linear. At longer reaction time (24 h), there is a reduction in the intensities of these signals, which maybe due to the polyesterifications with the secondary OH.
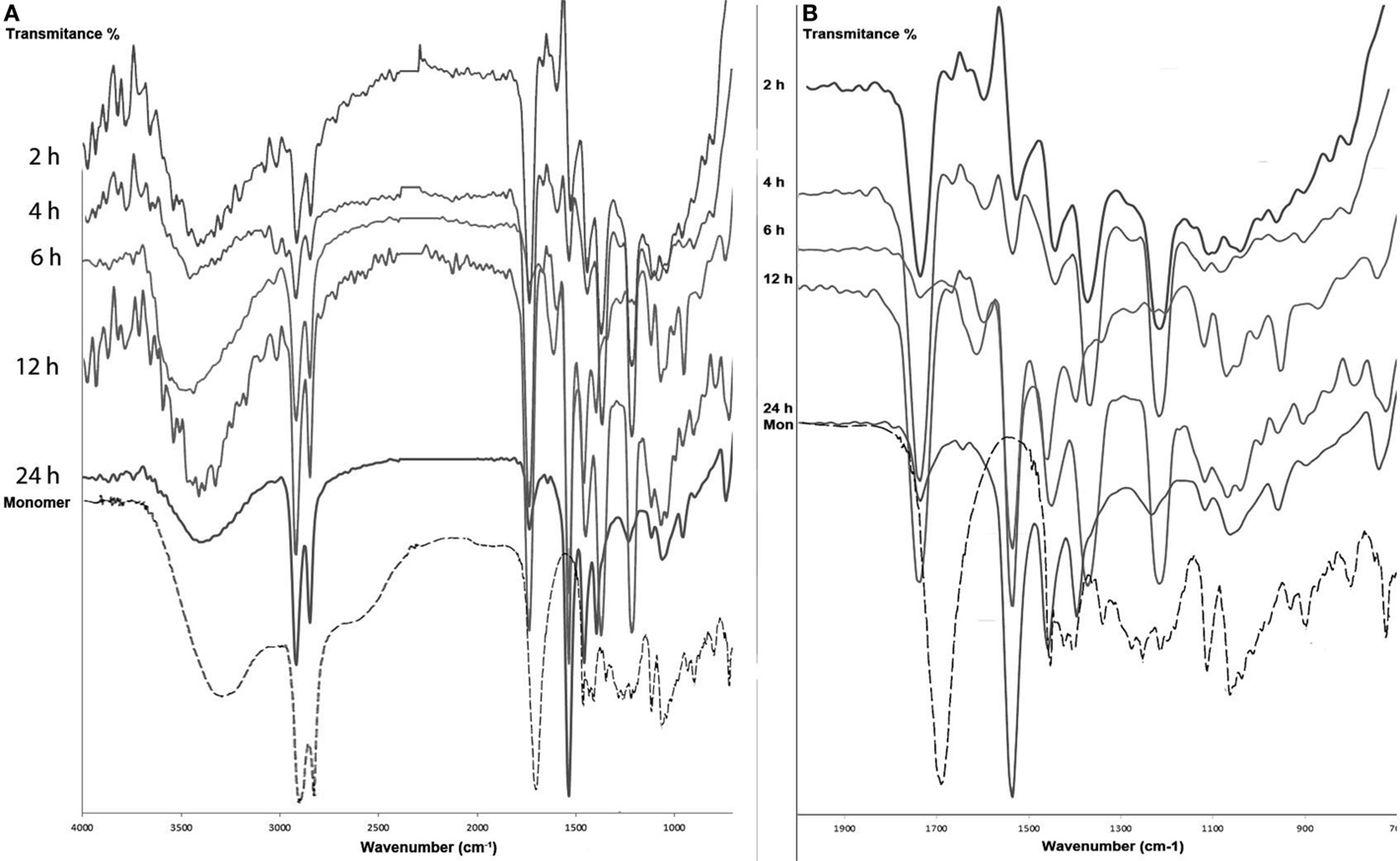
Figure 2. ATR-FT-IR spectra (A) and fingerprint region (B) of the polymer obtained at 90°C at different times.
CPMAS 13C NMR Analysis
To further characterize the polyesters, solid-state CPMAS 13C NMR spectra have been acquired (Figure 3). The main carbonyl peak at 174.2 ppm evidences the formation of the corresponding esters. The ester peak should be accompanied by a decreasing component of free –COOH groups at −177 ppm. However, we could not see the presence of this peak, which indicates the complete consumption of the monomer. As we can see in Figures 1–3, a single peak at 174.2 ppm was observed in the reaction at 80°C. This is in agreement for a linear polymer such as those obtained with enzymatic reactions (Gómez-Patiño et al., 2013) and in agreement with the FT-IR analysis. However, from reaction at 90°C (Figures 2 and 3), a peak at 172 ppm showed up and this signal increased in reaction at 100°C (Figure 3). This could be due to the esterification with the secondary OH’s at position 10.
According to our previous NMR analysis of polyesters obtained from enzymatic reactions, the signals for esterified carbons of primary –OH are at ≈63–64 ppm and carbons of secondary −OH are at ≈72–74 ppm (Gómez-Patiño et al., 2013). The analysis in the C–O region shows that most of the primary hydroxyls are esterified (intense peak at 65.0 ppm, –CH2–CO–O–CH2– versus the weak contribution at 63 ppm, HO–CH2–). Consequently, the esterification of secondary hydroxyls has to be a minority because of the COOH/OH imbalance in the monomer mixtures. The peak at 75.0 ppm (Figures 2 and 3) is then probably due to free secondary hydroxyl groups, and the weak signals around 73 and 72 ppm reveal the minor formation of esters in this position. This confirms that most of the polymers have a linear structure.
AFM Analysis
The topography of bulk polymers was analyzed by AFM. The roughness study showed that polyesters obtained at 90°C (24 h) has the highest values with an Rq 200 nm and an Ra 159 nm, while those obtained at 100°C (24 h) showed values of Rq 196 nm and Ra 157 nm, which resulted very similar. However, the polyester obtained at 80°C (24 h) presented the lowest roughness with an Rq 146 nm and an Ra 113 nm. The roughness analysis did not show a clear difference between the analyzed polyesters.
However, the AFM amplitude error images showed clear differences. In Figure 4B, agglomerates of particles sizing about 100 nm can be observed, while in Figure 4D, it is possible to observe also the agglomeration of particles and some well-defined islands with sizes about 150 nm, and finally in Figure 4F, an amorphous structure conformation is clearly observed and individual particles were not found.
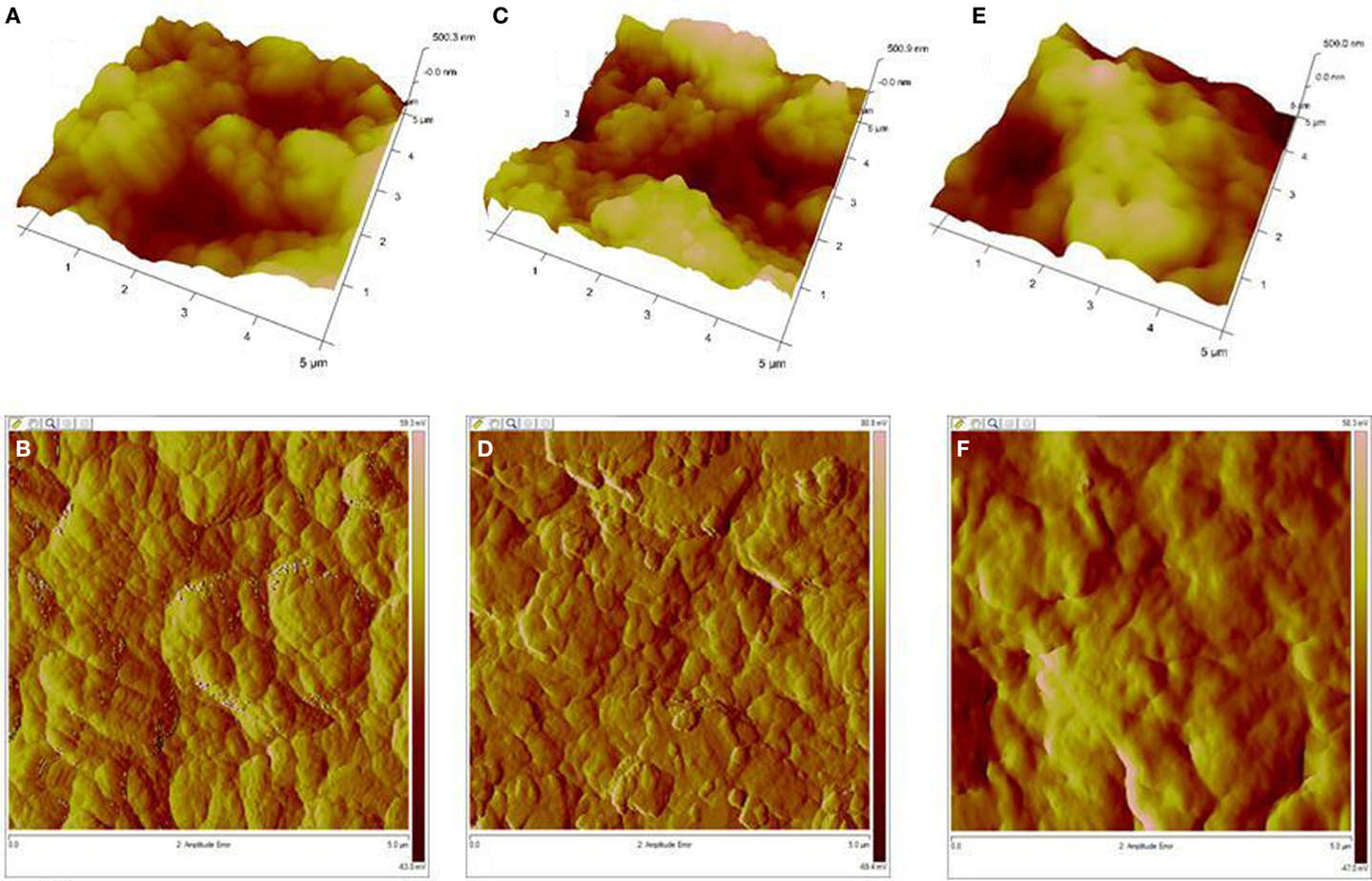
Figure 4. Topographical images obtained using an atomic force microscope from polyesters of 10,16-dihydroxyhexadecanoic acid at (A) 80°C, (B) 90°C, and (C) 100°C and their amplitude error images (B,D,F).
The image analysis may suggest that reactions at 80°C could produce polymeric chains with different molecular weights that could have partial conformation and crystallinity. Most of this sample looks amorphous (Figure 4B). The increasing polyesterification could lead to the presence of isolated particles in Figure 4D (90°C), and finally the topography shown in Figure 4F (100°C) may be related to the higher degree of polyesterification at this temperature.
X-Ray Diffraction Analysis (Small- and Wide-Angle Scattering)
The X-ray patterns from the polyester obtained from 10,16-dihydroxyhexadecanoic at 80, 90, and 100°C at 12 and 24 h were measured with small- and wide-angle X-ray scattering (SWAXS) in a conventional diffractometer with adequate optics as explained in Section “Methods.” The results were surprising since the polyesters looks like the crystalline structure of the potassium palmitate (C16H31KO2) with PDF # 00-007-0730 (black lines in Figure 5). Unfortunately, there are no polymer data to correlate the X-ray patterns with this kind of polyesters, and we are working with the analysis of different long-chain polyesters to understand this behavior. However, semicrystallinity has been reported in polyesters with long-chain hydrocarbon segments derived of oleic and erucic acid (Stempfle et al., 2014).
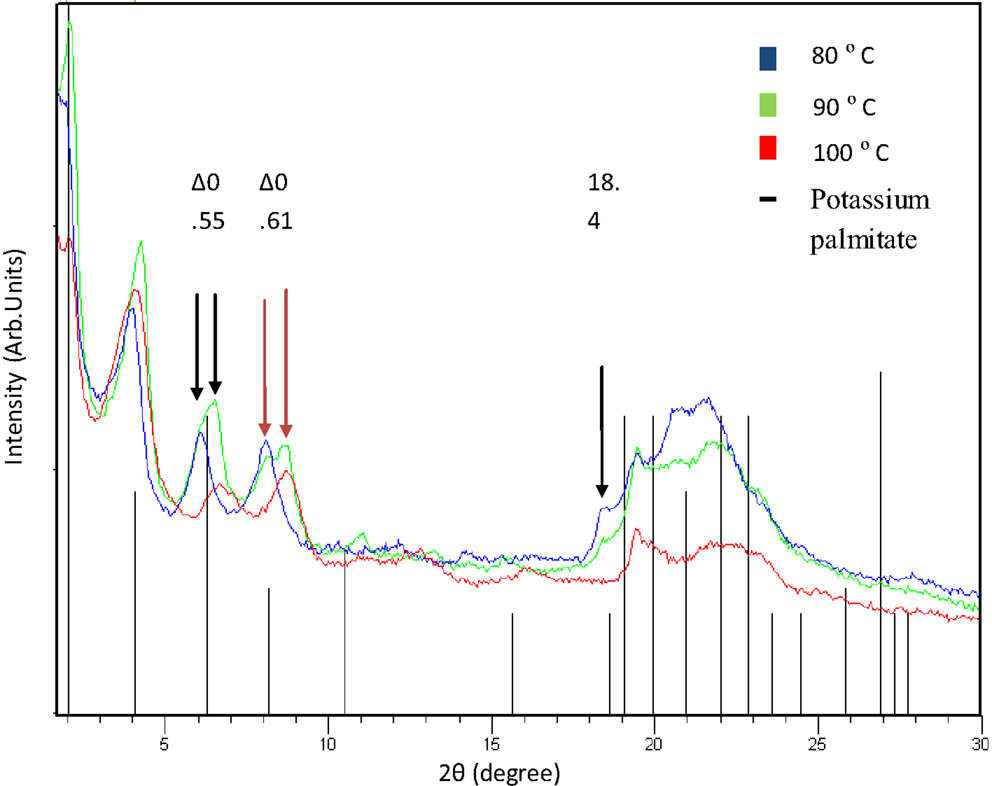
Figure 5. X-ray patterns from polyesters obtained at 80, 90, and 100°C with 12 h of reaction (Cu Kα = 1.54 Å). Black lines correspond to potassium palmitate (C16H31KO2) PDF # 00-007-0730 crystalline structure.
Figure 5 shows the X-ray patterns from polyesters obtained at 80, 90, and 100°C for 12 h of reaction. There is a small glide for the peak in the position 4.0 for sample at 80°C to 4.3 for sample at 90°C and back to 4.15 for sample at 100°C. The effect is due to changes in the interplanar distance at 2.207, 2.053, and 2.127 nm, respectively. Also, it can be observed that the peak at 6.11 moves to 6.66° and the peak at 8.1 changes to 8.71° in the samples at 80 and 100°C, respectively, with both contributions in the sample at 90°C. This observation indicates that the interplanar distance of the polyesters change from 14.48 to 13.51 nm and from 10.9 to 10.1 nm with the change in reaction temperature of 80–100°C, respectively, according to Bragg’s law. However, both structures with the same parameters are present in the polymer obtained at 90°C. Another displacement is observed with the peak at 18.4° that changes when temperature increases. However, it disappears in the polyester obtained at 100°C.
Figure 6 shows the X-ray patterns from polyesters obtained at 80, 90, and 100°C for 24 h of reaction. The changes observed in the peaks are similar to those seen in the samples obtained at 12 h for the different temperatures. The best crystalline structure is observed in the sample at 90°C for 24 h. In this case, the peaks are more defined and they have more intensity. More comprehensive studies are underway to understand and explain the changes in the structure of these new polymers.
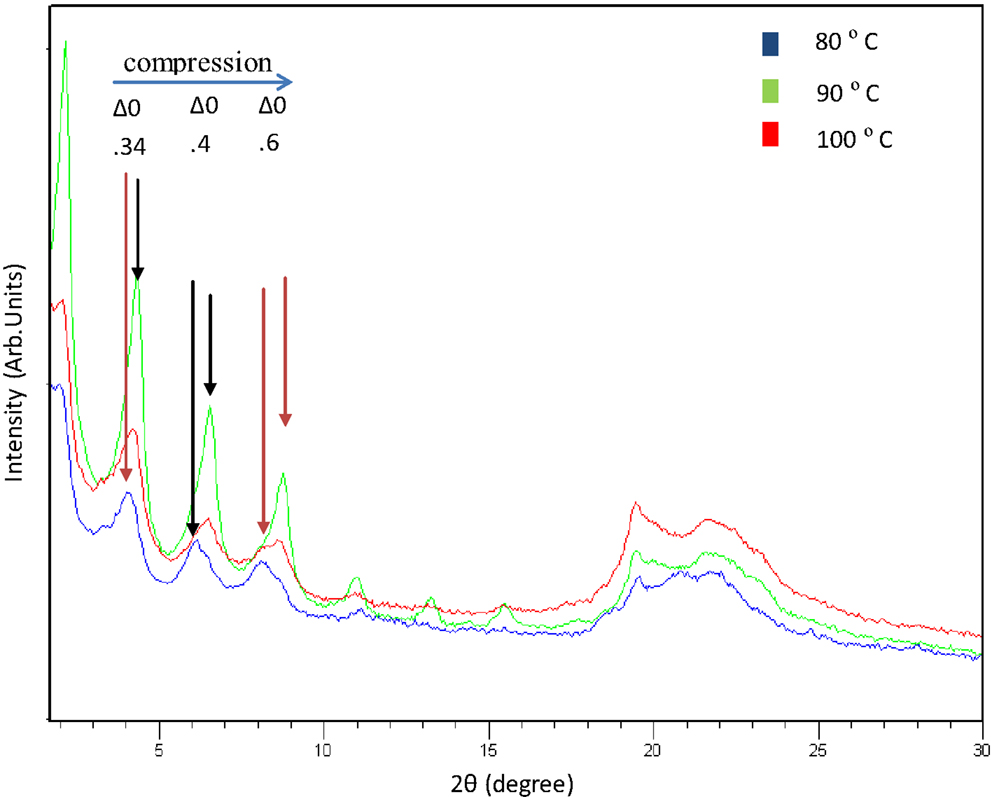
Figure 6. X-ray patterns from polyesters obtained at 80, 90, and 100°C with 24 h of reaction (Cu Kα = 1.54 Å).
Thermal Properties of Polymers
DSC analysis was essential to analyze the polymerization homogeneity in the reactions at different times. While reactions obtained in the first hours and lower temperatures showed more than two endothermic transitions, reactions at longer times and higher temperatures showed only one, indicating that polymers obtained at these times (12 and 24 h) could have more homogenous physicochemical characteristics. The DSC thermograms of polymers obtained at 80, 90, and 100°C at 12 and 24 h of reaction are shown in Figure 7. For the polymers at 12 h of reaction at 80 and 90°C, two endothermic transitions (Tm) can be observed at around 115 and 122°C. Meanwhile for the same reaction time but at 100°C, only one endothermic transition is observed at 105°C. At longer reaction times, 24 h, only one endothermic transition at 109 and 90°C was detected for reaction temperatures of 90 and 100°C, respectively. For the case of the polymers obtained at 80°C for 24 h, two endothermic transitions are still observed at almost the same temperature that the ones observed at 12 h of reaction.
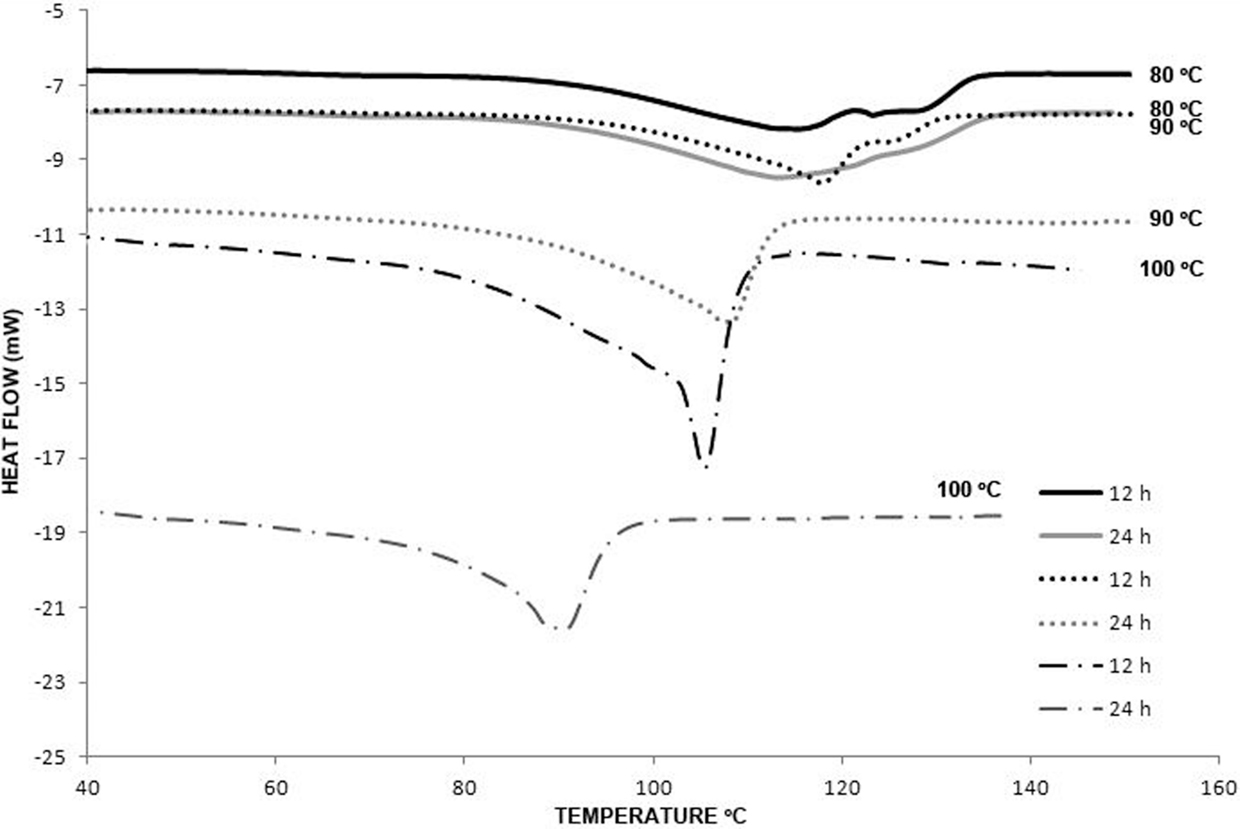
Figure 7. DSC thermograms of the polyesters obtained at 80, 90, and 100°C at 12 and 24 h. Endothermal transitions (Tm) are detected in the polymers (see text for description).
The fact that two endothermic transitions are observed after 12 h of reaction could be attributed to high-molecular-weight dispersion, due to the presence of different chain sizes (larger short-range ordered domains), since it has been described that in polymerization by polycondensation reactions, the chain length is not completely controlled (Okada, 2002; Albertsson and Srivastava, 2008; You et al., 2010). Also SWAXS results demonstrated that all the polymers showed crystallinity that could explain the relatively high temperature of the transitions observed. Above these temperatures, the vibrational energy of the polymer chain is sufficient to overcome secondary bonds between the chains and allow for translational chain motion.
In this sense, the sample obtained at 90°C for 24 h is the one with the highest crystalline structure since the peak and the temperature of transition at its thermogram are the most defined, in agreement with what is observed in SWAXS.
One interesting observation is that the transition temperatures for the samples at 90 and 100°C for 24 h reaction are reduced compared with the ones obtained after 12 h. A possible explanation could be that some polymers present branching that reduces the percentage of crystallinity. In this case, the transition temperatures decrease since the molecules are less densely packed and therefore cannot form Van der Waals interactions or hydrogen bonds as easily.
Conclusion
We have used the IL, choline chloride·2ZnCl2, to polymerize efficiently the main monomer of tomato cuticle. The 10,16-DHPA was obtained from tomato residual wastes and used to produce polymers at different times and temperatures. According to the FT-IR and CPMAS 13C NMR analysis, the reaction produces polymers that are mainly linear. The best experimental conditions to get these polymers were 90°C at 24 h and 100°C at 12 h. However, at longer times and higher temperatures, polymers show branched structures with esterification in the −OH-10. The high polymerization and the branching present in the polymers could be the reason for the insolubility observed in different solvents. The use of choline chloride·2ZnCl2 in the polymerization of 10,16-DHPA could be more efficient than those common process used to esterify long-chain fatty acids, like the DCC/DMAP reaction. Beside, it is cheap, very easy to prepare, and can be reused for at least six cycles without any pretreatment or significant loss of activity. Polymers obtained from these reactions have potential use for a wide range of biomedical applications.
Conflict of Interest Statement
The authors declare that the research was conducted in the absence of any commercial or financial relationships that could be construed as a potential conflict of interest.
Acknowledgments
The study was financed by ICyTDF through project PICSO12-054 and to the National Polytechnic Institute (IPN) for the SIP grants (20140058, 20150482 and 20150236). Thanks to the CONACyT for the Postdoctoral Fellowship of M. Beatriz Gómez-Patiño. Our gratitude to the BIOCATEM NETWORK support.
References
Abbott, A. P., Boothby, D., Capper, G., Davies, D. L., and Rasheed, R. K. (2004). Deep eutectic solvents formed between choline chloride and carboxylic acids: versatile alternatives to ionic liquids. JACS 126, 9142. doi: 10.1021/ja048266j
Ahmed, A., Crawford, T., Gould, S., Ha, Y. S., Hollrah, M., Noor-E-Ain, F., et al. (2003). Synthesis of (R)- and (S)-10,16-dihydroxyhexadecanoic acid: cutin stereochemistry and fungal activation. Phytochemistry 63, 47–52. doi:10.1016/S0031-9422(03)00003-7
Akinori, T., Yoshika, O., Yoshitaka, I., Yoshihito, I., and Tadamichi, H. (2003). Synthesis of aliphatic polyesters by direct polyesterification of dicarboxylic acids with diols under mild conditions catalyzed by reusable rare-earth triflate. Macromolecules 36, 1772–1774. doi:10.1021/ma021462v
Albertsson, A. C., and Varma, I. K. (2002). “Aliphatic polyesters: synthesis, properties and applications” in Degradable Aliphatic Polyesters, ed. A. C. Albertsson (Berlin: Springer-Verlag), 1–40.
Albertsson, A. Ch, and Srivastava, K. R. (2008). Recent developments in enzyme-catalyzed ring-opening polymerization. Adv. Drug Deliv. Rev. 60, 1077–1093. doi:10.1016/j.addr.2008.02.007
Arrieta-Baez, D., Cruz-Carrillo, M., Gómez-Patiño, M. B., and Zepeda-Vallejo, L. G. (2011). Derivatives of 10,16-dihydroxyhexadecanoic acid isolated from tomato (Solanum lycopersicum) as potential material for aliphatic polyesters. Molecules 16, 4923–4936. doi:10.3390/molecules16064923
Bayer, I. S., Guzman-Puyol, S., Heredia-Guerrero, J. A., Ceseracciu, L., Pignatelli, F., Ruffilli, R., et al. (2004). Direct transformation of edible vegetable waste into bioplastics. Macromolecules 47, 5135–5143. doi:10.1021/ma5008557
Benitez, J. J., García-Segura, R., and Heredia, A. (2004). Plant biopolyester cutin: a tough way to its chemical synthesis. Biochim. Biophys. Acta 1674, 1–3. doi:10.1016/j.bbagen.2004.06.012
Benítez, J. J., Heredia-Guerrero, J. A., Guzmán-Puyol, S., Domínguez, E., and Heredia, A. (2015). Long-chain polyhydroxyesters from natural occurring aleuritic acid as potential material for food packaging. Soft Mater. 13, 5–11. doi:10.1080/1539445X.2014.993476
Chen, G. Q., and Wu, Q. (2005). The application of polyhydroxyalkanoates as tissue engineering materials. Biomaterials 26, 6565–6578. doi:10.1016/j.biomaterials.2005.04.036
Gómez-Patiño, M. B., Cassani, J., Jaramillo-Flores, M. E., Zepeda-Vallejo, L. G., Sandoval, G., Jimenez-Estrada, M., et al. (2013). Oligomerization of 10,16-dihydroxyhexadecanoic acid and methyl-10,16-dihydroxyhexadecanoate catalyzed by lipases. Molecules 18, 9317–9333. doi:10.3390/molecules18089317
Heredia-Guerrero, J. A., Heredia, A., García-Segura, R., and Benitez, J. J. (2009). Synthesis and characterization of a plant cutin mimetic polymer. Polymer 50, 5633–5637. doi:10.1016/j.polymer.2009.10.018
Lecomte, P., Riva, R., Schmeits, S., Rieger, J., Van Butsele, K., and Jerome, C. (2006). New prospects for the grafting of functional groups onto aliphatic polyesters. Ring opening polymerization of alpha- or gamma-substituted epsilon-caprolactone followed by chemical derivatization of the substituents. Macromol. Symp. 240, 157–165. doi:10.1002/masy.200650820
Nair, L. S., and Laurencin, C. T. (2007). Biodegradable polymers as biomaterials. Prog. Polym. Sci. 32, 762–798. doi:10.1016/j.progpolymsci.2007.05.017
Okada, M. (2002). Chemical synthesis of biodegradable polymers. Prog. Polym. Sci. 27, 87–133. doi:10.1016/S0079-6700(01)00039-9
Olsson, A., Lindstrom, M., and Iversen, T. (2007). Lipase-catalyzed synthesis of an epoxy-functionalized polyester from the suberin monomer cis-9,10-Epoxy-18-hydroxyoctadecanoic acid. Biomacromolecules 8, 757–760. doi:10.1021/bm060965w
Osman, S. F., Gerard, H. C., Fett, W. F., Moreau, R. A., and Dudley, R. L. (1995). Method for the production and characterization of tomato cutin oligomers. J. Agric. Food Chem. 43, 2134–2137. doi:10.1021/jf00056a033
Osman, S. F., Irwin, P., Fett, W. F., O’Connor, J. V., and Parris, N. (1999). Preparation, isolation, and characterization of cutin monomers and oligomers from tomato peels. J. Agric. Food Chem. 47, 799–802. doi:10.1021/jf980693r
Parrish, B., and Emrick, T. (2006). Strategies in aliphatic polyester synthesis for biomaterial and drug delivery applications. ACS Symp. Ser. 939, 248–266. doi:10.1021/bk-2006-0939.ch016
Philip, S., Keshavarz, T., and Roy, I. (2007). Polyhydroxyalkanoates: biodegradable polymers with a range of applications. J. Chem. Technol. Biotechnol. 82, 233–247. doi:10.1002/jctb.1667
Quinzler, D., and Mecking, S. (2010). Linear semicrystalline polyesters from fatty acids by complete feedstock molecule utilization. Angew. Chem. Int. Ed. 49, 4306. doi:10.1002/anie.201001510
Seddon, K. R. (1997). Ionic liquids for clean technology. J. Chem. Tech. Biotechnol. 68, 351. doi:10.1002/(SICI)1097-4660(199704)68
Shu, K., Iwao, K., and Yoshinori, Y. (1994). Repeated use of the catalyst in Ln(OTf)3-catalyzed aldol and allylation reactions. J. Chem. Soc. Jpn. 67, 2342–2344. doi:10.1246/bcsj.67.2342
Stempfle, F., Ritter, B. S., Mülhaupt, R., and Mecking, S. (2014). Long-chain aliphatic polyesters from plant oils for injection molding, film extrusion and electrospinning. Green Chem. 16, 2008–2014. doi:10.1039/c4gc00114a
Sunitha, S., Kanjilal, S., Reddy, P. S., and Prasad, R. B. N. (2007). Liquid–liquid biphasic synthesis of long chain wax esters using the Lewis acidic ionic liquid choline chloride·2ZnCl2. Tetrahedron Lett. 48, 6962–6965. doi:10.1016/j.tetlet.2007.07.159
Welton, T. (1999). Room-temperature ionic liquids. Solvents for synthesis and catalysis. Chem. Rev. 99, 2071. doi:10.1021/cr980032t
Williams, C. K. (2007). Synthesis of functionalized biodegradable polyesters. Chem. Soc. Rev. 36, 1573–1580. doi:10.1039/b614342n
Yamamoto, M., Witt, U., Skupin, G., Beimborn, D., and Muller, R.-J. (2002). “Biodegradable aliphatic-aromatic polyesters: “Ecoflex®,” in Biopolymers, Polyesters III, Chap. 11. Vol. 4, eds A. Steinbuchel and Y. Doi (Weinheim: Wiley-VCH), 299–311.
Keywords: ionic liquids, long-chain aliphatic polyesters, tomato, 10,16-DHPA, choline chloride·2ZnCl2
Citation: Gómez-Patiño MB, Gutiérrez-Salgado DY, García-Hernández E, Mendez-Mendez JV, Andraca Adame JA, Campos-Terán J and Arrieta-Baez D (2015) Polymerization of 10,16-Dihydroxyhexadecanoic Acid, Main Monomer of Tomato Cuticle, Using the Lewis Acidic Ionic Liquid Choline Chloride·2ZnCl2. Front. Mater. 2:67. doi: 10.3389/fmats.2015.00067
Received: 01 August 2015; Accepted: 26 October 2015;
Published: 11 November 2015
Edited by:
José Alejandro Heredia-Guerrero, Fondazione Istituto Italiano di Tecnologia, ItalyReviewed by:
Daniela Pappalardo, Università del Sannio, ItalyAntonio Perejon, Universidad de Sevilla, Spain
Copyright: © 2015 Gómez-Patiño, Gutiérrez-Salgado, García-Hernández, Mendez-Mendez, Andraca Adame, Campos-Terán and Arrieta-Baez. This is an open-access article distributed under the terms of the Creative Commons Attribution License (CC BY). The use, distribution or reproduction in other forums is permitted, provided the original author(s) or licensor are credited and that the original publication in this journal is cited, in accordance with accepted academic practice. No use, distribution or reproduction is permitted which does not comply with these terms.
*Correspondence: Daniel Arrieta-Baez, darrieta@ipn.mx