Contribution of emotional and motivational neurocircuitry to cue-signaled active avoidance learning
- 1Leibniz Institute for Neurobiology, Magdeburg, Germany
- 2Department of Psychology, The University of Texas, Austin, USA
- 3Institute of Biology, University of Magdeburg, Magdeburg, Germany
- 4Center for Behavioral Brain Sciences (CBBS), Magdeburg, Germany
Introduction
Many animal and human subjects can learn to avoid punishment or noxious stimuli by exploiting the sensory cues predicting them. In cue-signaled active avoidance (AA) learning, subjects first learn about the predictive properties of cues and subsequently learn a behavioral strategy of an avoidance response (e.g., crossing a hurdle that divides a two-compartment cage in order to avoid a mild but unpleasant footshock). AA learning develops with fear reduction as an intervening variable (Mowrer and Lamoreaux, 1946; Miller, 1948). If execution of avoidance action occurs on the pursuit of seeking safety, an overlap of recruitments of neurocircuitry essential for reward processing and for avoidance can be expected on the basis of two-process theory. Recent insights from two-way AA (2WAA) studies, which integrate both Pavlovian and instrumental components, provide strong evidence for the recruitment of emotional circuitry centered on the amygdala and motivational circuitry centered around midbrain dopaminergic structures. In this review, we address the following: (1) the role of emotional neurocircuitry in the formation of AA, (2) the involvement of reward circuitry and its input-output pathways on AA, and (3) the possible serial and parallel processing within and between these circuitries.
Role of Emotional Circuitry in AA
Pavlovian learning increases synaptic plasticity in amygdala neurons (Tye et al., 2008) that respond to cues predicting either appetitive or aversive outcomes (Paton et al., 2006; Tye and Janak, 2007). While the amygdala has been studied extensively for its involvement in fear processing (LeDoux, 2000), recent studies highlight the amygdala as a key substrate during acquisition of AA. Studies indicate that different subnuclei of the amygdala contribute differently to the acquisition of an avoidance strategy and to the consolidation of avoidance memories. Bilateral electrolytic lesions of the basolateral amygdala (BLA) (Segura-Torres et al., 2010) and pre-training infusion of an NMDA antagonist into the BLA impair the acquisition of 2WAA learning (Savonenko et al., 2003). Central amygdala (CeA) lesions disrupt the acquisition of an AA response but have no effect on the retrieval of a previously acquired AA response (Roozendaal et al., 1993). However, for animals that failed to acquire 2WAA after 3 days of training, CeA lesions actually improved AA learning (Choi et al., 2010). Thus, the BLA appears important for all phases of AA learning whereas the role of the CeA appears to be limited to acquisition and complex, potentially facilitating or impeding AA learning depending on the specific AA response, and/or innate individual differences in AA learning ability.
During the initial stages of AA acquisition, the expression of conditioned freezing to the CS can interfere with AA learning. In such situations, the infralimbic prefrontal cortex (IL) exerts feed forward inhibition of the amygdala to reduce the expression of freezing responses to the CS while sparing the predictive association between the CS and US, which is necessary for AA learning (Moscarello and LeDoux, 2013). Studies on rabbits trained to induce wheel rotation to avoid shock during CS+ presentations confirm the involvement of amygdala (LA, B, and Ce), cingulate cortex, thalamus, and auditory cortex in the acquisition and retention of AA (Smith et al., 2001). Intra-BLA infusion of muscimol significantly affected the acquisition of discriminative avoidance with two tones but did not affect the CR to both CS after overtraining (Poremba and Gabriel, 1997, 1999).
Role of Motivational Circuitry in AA
Given the heterogeneity of the ventral tegmental area (VTA), it is not surprising that dopamine (DA) neurons play different roles ranging from signaling reward and mediating motivation to coding aversion, salience, uncertainty, and novelty (Horvitz, 2000; Bromberg-Martin et al., 2010; Ilango et al., 2012; Lammel et al., 2014). Additional complexity became evident after the discovery of subsets of DA neurons co-transmitting glutamate in the nucleus accumbens (NAc) shell (Stuber et al., 2010), GABA in the dorsal striatum (Tritsch et al., 2012) and GABA in the lateral habenula (LHb) (Stamatakis et al., 2013) raising several specific questions about the involvement of this system.
Here, we will discuss the tonic and phasic DA release associated with signaled AA. Briefly, DA antagonists impair AA responses, and electrical stimulation of reward circuitry facilitates AA [see reviews by Salamone (1994) and Ilango et al. (2012)]. NAc DA release increases during the first training block of 2WAA and progressively decreases in the second training block as the number of AA responses increase (Dombrowski et al., 2013). Signaled AA learning progresses with the increase of tonic DA release in medial prefrontal cortex (mPFC) and reaches its peak during the formation of the first successful avoidance trails (Stark et al., 1999, 2001). Elevated DA release in mPFC was also found in a training scenario in which two different cues were first associated with the same meaning (signaling Go response) and were subsequently associated with different meanings (Go and NoGo) (Stark et al., 2004).
Recent studies implicate the relevance of the phasic DA signal to punishment prediction and avoidance. During the safety period, in both avoidance and escape, DA release in the NAc was increased (Oleson et al., 2012). Furthermore, brief electrical stimulation applied to the LHb contingent to the AA response (i.e., at the initiation of the safety period) impaired acquisition but not retention of 2WAA (Shumake et al., 2010; Ilango et al., 2013; Shumake and Gonzalez-Lima, 2013). Studies utilizing viral gene therapy in DA deficient mice showed that shock escape and learning 2WAA require DA signaling in both the amygdala and striatum. And after overtraining (which is more resistant to extinction), DA signaling in the striatum alone was sufficient to maintain 2WAA. In contrast, restoring DA signaling in the PFC and amygdala was insufficient to maintain AA (Darvas et al., 2011). Careful lesion experiments in different regions of the striatum confirmed that NAc core and dorsolateral striatum (DLS) lesions delayed 2WAA acquisition without disrupting the ability to acquire AA. In contrast, dorsomedial striatum (DMS) lesion did not affect the early phase but decreased 2WAA after six training sessions (Wendler et al., 2014). Pre-training infusion of D1 or D2 antagonist into the DLS did not affect the number of AA responses during training but significantly decreased AA responses during the retention test 24 h later (Boschen et al., 2011; Wietzikoski et al., 2012).
Also, the laterodorsal tegmental nucleus (LDTg) and pedunculopontine tegmental nucleus (PPTg), which send glutamatergic and cholinergic projections to the midbrain, play an important role in 2WAA (Mena-Segovia et al., 2008; Lammel et al., 2012). Bilateral lesions of the PPTg completely abolished the acquisition of 2WAA (Fujimoto et al., 1989). Rats with ipsilateral disconnection of the SNc from the PPTg learned the 2WAA, but contralateral disconnection blocked learning even after 3 days of conditioning, suggesting that PPTg-SNc communication is necessary to acquire 2WAA (Bortolanza et al., 2010).
Perspectives
The majority of DA neurons are inhibited by aversive USs, or by CSs signaling aversive USs [for details see Ilango et al. (2012)]. Hypothetically, once the aversive CS–US association is repeated several times, the tonic inhibition mode changes and the change in DA dynamics prepare the organism to perform successful avoidance responses. Perhaps, this learning relevant change in DA signaling also led to change in synaptic plasticity occurring between hippocampal → amygdala neurons and the neurons of the direct pathway that are active at the same time, thus guiding the organism to repeat the instrumental response. There are several pathways that could provide midbrain DA neurons with information about aversive events. Nociceptive signals from the spinal cord pass pain-related signals to the parabrachial nucleus (PBN). Through its direct glutamatergic projection or indirect glutamatergic route to the rostromedial tegmental nucleus (RMTg) and VTA GABA neurons, the aversive signal is relayed to midbrain VTA/SNc DA neurons. Inactivation of the PBN either reduces the amplitude or completely abolishes the inhibitory response of DA neurons to footshock (Coizet et al., 2010). In addition, modulation of pain and aversion-related signals conveyed by the LHb reaches DA neurons directly or indirectly through the RMTg (Jhou et al., 2009). This informative signal is highly processed and capable of assigning motivational valence based on prior events. Indeed, unexpected footshock increased LHb-to-RMTg glutamate release, and optogenetic activation (60 Hz) of this pathway promoted place aversion and AA learning to prevent the activation (Stamatakis and Stuber, 2012).
There is also evidence that CeA–DA interactions may be important for AA learning. The CeA projects to substantia nigra DA neurons and to the DLS. Moreover, electrical stimulation of the CE modulates the firing of SNc DA neurons (Rouillard and Freeman, 1995). The CE is also known to strengthen the effect of Pavlovian stimuli on instrumental performance, and the CeA → DLS pathway is implicated in habit acquisition (Corbit and Balleine, 2005; Lázaro-Muñoz et al., 2010; Lingawi and Balleine, 2012). Reciprocally, the lateral part of SNc DA neurons project to the CeA, playing a role in surprise-induced enhancement of attention and learning (Lee et al., 2006, 2008). Accordingly, the first successful avoidance trial in an AA paradigm likely constitutes a “pleasant surprise” for the animal – a violation of the expectation that the CS is always followed by the US – and we hypothesize that early AA success may recruit the SNc → CeA pathway and consequently enhance attention and learning of the AA response.
From the above-mentioned evidences, it is clear that parallel streams of information might reach the amygdala and DA midbrain structures, and both systems might interact at the striatum level (Figure 1). Blocking transmission of glutamate signals by knocking out the NMDA receptor in medium spiny neurons of the striatum impaired learning of a simple FR1 operant task for food reward as well as 2WAA. This confirms that 2WAA recruits simple motor coordination circuits (Beutler et al., 2011). Moreover, striatal specific deletion of adenosine A(2A) receptors impaired 2WAA. This is interesting given the known role of these receptors in fine-tuning the DA-glutamate balance in the striatum (Singer et al., 2013).
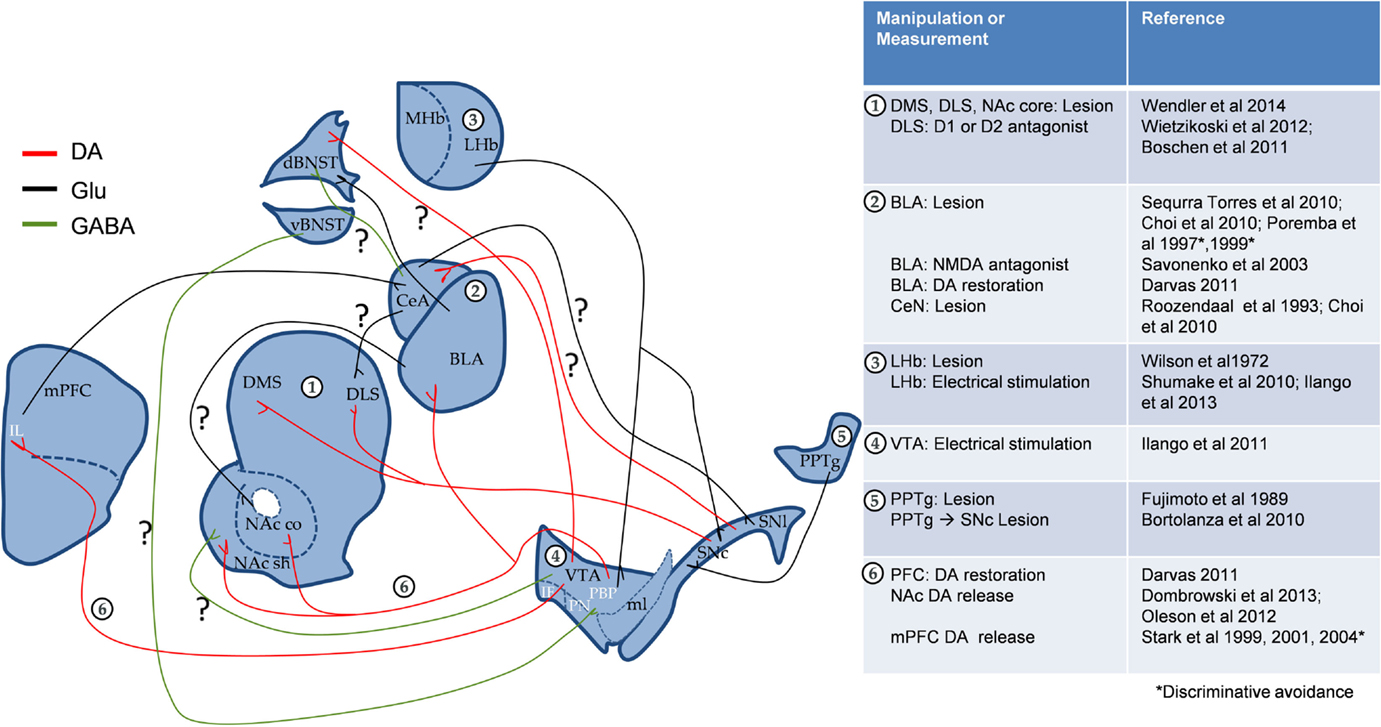
Figure 1. Proposed interaction of emotional and motivational neurocircuitry in cue-signaled AA learning. Apart from the known role of BLA DA in AA, both circuits could interact through direct SNc←→CeA projections and/or indirectly through striatum and BNST. “?” Denotes the pathways, which could potentially play a role in AA. mPFC, medial prefrontal cortex (IL, infra limbic); NAc, nucleus accumbens (c, core, and sh, shell); VTA, ventral tegmental area (PBP, parabrachial pigmented nucleus; PN, paranigral nucleus; IF, interfascicular nucleus); SN, substantia nigra (c, compact part and l, lateral part); ml, medial lemniscus; CeA, central nucleus of the amygdala; BLA, basolateral amygdala; BNST, bed nucleus of the stria terminalis; MHb and LHb, medial and lateral habenula; PPTg, pedunculopontine tegmental nucleus; DMS, dorsomedial striatum; DLS, dorsolateral striatum. To simplify the circuit, the direct and indirect pathways of striatum were excluded.
Unlike reward learning, when a subject masters AA, it no longer receives external motivation in the form of a US, providing a mystery to early learning theorists. How can behavior be sustained in the absence of reinforcement? One solution is that a fear memory of the US continues to be evoked by the CS, and alleviation of this fear state can continue to motivate the AA response in the absence of the US. But if the CS is no longer paired with the US, why does the CS–US association not undergo extinction? Moreover, animals that have mastered an AA task no longer show strong physiological signs of fear or distress. For these animals, the execution of the avoidance response takes on the quality of a habit. Therefore, we propose that AA learning ultimately recruits and depends on the same circuitry involved in habit formation, such as the so-called spiraling loop of striatal–nigral–striatal circuitry (Yin and Knowlton, 2006; Belin and Everitt, 2008; Ilango et al., 2014). We believe that this circuitry is a prime target for investigating the neural mechanisms that sustain avoidance behavior, and it may reveal novel ways of facilitating its extinction.
Conflict of Interest Statement
The authors declare that the research was conducted in the absence of any commercial or financial relationships that could be construed as a potential conflict of interest.
References
Belin, D., and Everitt, B. J. (2008). Cocaine seeking habits depend upon dopamine-dependent serial connectivity linking the ventral with the dorsal striatum. Neuron 57, 432–441. doi: 10.1016/j.neuron.2007.12.019
Pubmed Abstract | Pubmed Full Text | CrossRef Full Text | Google Scholar
Beutler, L. R., Eldred, K. C., Quintana, A., Keene, C. D., Rose, S. E., Postupna, N., et al. (2011). Severely impaired learning and altered neuronal morphology in mice lacking NMDA receptors in medium spiny neurons. PLoS ONE 6:e28168. doi:10.1371/journal.pone.0028168
Pubmed Abstract | Pubmed Full Text | CrossRef Full Text | Google Scholar
Bortolanza, M., Wietzikoski, E. C., Boschen, S. L., Dombrowski, P. A., Latimer, M., Maclaren, D. A., et al. (2010). Functional disconnection of the substantia nigra pars compacta from the pedunculopontine nucleus impairs learning of a conditioned avoidance task. Neurobiol. Learn. Mem. 94, 229–239. doi:10.1016/j.nlm.2010.05.011
Pubmed Abstract | Pubmed Full Text | CrossRef Full Text | Google Scholar
Boschen, S. L., Wietzikoski, E. C., Winn, P., and Da Cunha, C. (2011). The role of nucleus accumbens and dorsolateral striatal D2 receptors in active avoidance conditioning. Neurobiol. Learn. Mem. 96, 254–262. doi:10.1016/j.nlm.2011.05.002
Pubmed Abstract | Pubmed Full Text | CrossRef Full Text | Google Scholar
Bromberg-Martin, E. S., Matsumoto, M., and Hikosaka, O. (2010). Dopamine in motivational control: rewarding, aversive, and alerting. Neuron 68, 815–834. doi:10.1016/j.neuron.2010.11.022
Pubmed Abstract | Pubmed Full Text | CrossRef Full Text | Google Scholar
Choi, J. S., Cain, C. K., and LeDoux, J. E. (2010). The role of amygdala nuclei in the expression of auditory signaled two-way active avoidance in rats. Learn. Mem. 17, 139–147. doi:10.1101/lm.1676610
Pubmed Abstract | Pubmed Full Text | CrossRef Full Text | Google Scholar
Coizet, V., Dommett, E. J., Klop, E. M., Redgrave, P., and Overton, P. G. (2010). The parabrachial nucleus is a critical link in the transmission of short latency nociceptive information to midbrain dopaminergic neurons. Neuroscience 168, 263–272. doi:10.1016/j.neuroscience.2010.03.049
Pubmed Abstract | Pubmed Full Text | CrossRef Full Text | Google Scholar
Corbit, L. H., and Balleine, B. W. (2005). Double dissociation of basolateral and central amygdala lesions on the general and outcome-specific forms of pavlovian-instrumental transfer. J. Neurosci. 25, 962–970. doi:10.1523/JNEUROSCI.4507-04.2005
Pubmed Abstract | Pubmed Full Text | CrossRef Full Text | Google Scholar
Darvas, M., Fadok, J. P., and Palmiter, R. D. (2011). Requirement of dopamine signaling in the amygdala and striatum for learning and maintenance of a conditioned avoidance response. Learn. Mem. 18, 136–143. doi:10.1101/lm.2041211
Pubmed Abstract | Pubmed Full Text | CrossRef Full Text | Google Scholar
Dombrowski, P. A., Maia, T. V., Boschen, S. L., Bortolanza, M., Wendler, E., Schwarting, R. K., et al. (2013). Evidence that conditioned avoidance responses are reinforced by positive prediction errors signaled by tonic striatal dopamine. Behav. Brain Res. 241, 112–119. doi:10.1016/j.bbr.2012.06.031
Pubmed Abstract | Pubmed Full Text | CrossRef Full Text | Google Scholar
Fujimoto, K., Yoshida, M., Ikeguchi, K., and Niijima, K. (1989). Impairment of active avoidance produced after destruction of pedunculopontine nucleus areas in the rat. Neurosci. Res. 6, 321–328. doi:10.1016/0168-0102(89)90024-2
Pubmed Abstract | Pubmed Full Text | CrossRef Full Text | Google Scholar
Horvitz, J. C. (2000). Mesolimbocortical and nigrostriatal dopamine responses to salient non-reward events. Neuroscience 96, 651–656. doi:10.1016/S0306-4522(00)00019-1
Pubmed Abstract | Pubmed Full Text | CrossRef Full Text | Google Scholar
Ilango, A., Kesner, A. J., Keller, K. L., Stuber, G. D., Bonci, A., and Ikemoto, S. (2014). Similar roles of substantia nigra and ventral tegmental dopamine neurons in reward and aversion. J. Neurosci. 34, 817–822. doi:10.1523/JNEUROSCI.1703-13.2014
Pubmed Abstract | Pubmed Full Text | CrossRef Full Text | Google Scholar
Ilango, A., Shumake, J., Wetzel, W., Scheich, H., and Ohl, F. W. (2011). Effects of ventral tegmental area stimulation on the acquisition and long-term retention of active avoidance learning. Behav. Brain Res. 225, 515–521. doi:10.1016/j.bbr.2011.08.014
Pubmed Abstract | Pubmed Full Text | CrossRef Full Text | Google Scholar
Ilango, A., Shumake, J., Wetzel, W., Scheich, H., and Ohl, F. W. (2012). The role of dopamine in the context of aversive stimuli with particular reference to acoustically signaled avoidance learning. Front. Neurosci. 6:132. doi:10.3389/fnins.2012.00132
Pubmed Abstract | Pubmed Full Text | CrossRef Full Text | Google Scholar
Ilango, A., Shumake, J., Wetzel, W., Scheich, H., and Ohl, F. W. (2013). Electrical stimulation of lateral habenula during learning: frequency-dependent effects on acquisition but not retrieval of a two-way active avoidance response. PLoS ONE 8:e65684. doi:10.1371/journal.pone.0065684
Pubmed Abstract | Pubmed Full Text | CrossRef Full Text | Google Scholar
Jhou, T. C., Fields, H. L., Baxter, M. G., Saper, C. B., and Holland, P. C. (2009). The rostromedial tegmental nucleus (RMTg), a GABAergic afferent to midbrain dopamine neurons, encodes aversive stimuli and inhibits motor responses. Neuron 61, 786–800. doi:10.1016/j.neuron.2009.02.001
Pubmed Abstract | Pubmed Full Text | CrossRef Full Text | Google Scholar
Lammel, S., Lim, B. K., and Malenka, R. C. (2014). Reward and aversion in a heterogeneous midbrain dopamine system. Neuropharmacology 76, 351–359. doi:10.1016/j.neuropharm.2013.03.019
Pubmed Abstract | Pubmed Full Text | CrossRef Full Text | Google Scholar
Lammel, S., Lim, B. K., Ran, C., Huang, K. W., Betley, M. J., Tye, K. M., et al. (2012). Input-specific control of reward and aversion in the ventral tegmental area. Nature 491, 212–217. doi:10.1038/nature11527
Pubmed Abstract | Pubmed Full Text | CrossRef Full Text | Google Scholar
Lázaro-Muñoz, G., LeDoux, J. E., and Cain, C. K. (2010). Sidman instrumental avoidance initially depends on lateral and basal amygdala and is constrained by central amygdala-mediated Pavlovian processes. Biol. Psychiatry 67, 1120–1127. doi:10.1016/j.biopsych.2009.12.002
Pubmed Abstract | Pubmed Full Text | CrossRef Full Text | Google Scholar
LeDoux, J. E. (2000). Emotion circuits in the brain. Annu. Rev. Neurosci. 2, 155–184. doi:10.1146/annurev.neuro.23.1.155
Lee, H. J., Youn, J. M., Gallagher, M., and Holland, P. C. (2008). Temporally limited role of substantia nigra-central amygdala connections in surprise-induced enhancement of learning. Eur. J. Neurosci. 27, 3043–3049. doi:10.1111/j.1460-9568.2008.06272.x
Pubmed Abstract | Pubmed Full Text | CrossRef Full Text | Google Scholar
Lee, H. J., Youn, J. M., O, M. J., Gallagher, M., and Holland, P. C. (2006). Role of substantia nigra-amygdala connections in surprise-induced enhancement of attention. J. Neurosci. 26, 6077–6081. doi:10.1523/JNEUROSCI.1316-06.2006
Pubmed Abstract | Pubmed Full Text | CrossRef Full Text | Google Scholar
Lingawi, N. W., and Balleine, B. W. (2012). Amygdala central nucleus interacts with dorsolateral striatum to regulate the acquisition of habits. J. Neurosci. 32, 1073–1081. doi:10.1523/JNEUROSCI.4806-11.2012
Pubmed Abstract | Pubmed Full Text | CrossRef Full Text | Google Scholar
Mena-Segovia, J., Winn, P., and Bolam, J. P. (2008). Cholinergic modulation of midbrain dopaminergic systems. Brain Res. Rev. 58, 265–271. doi:10.1016/j.brainresrev.2008.02.003
Miller, N. E. (1948). Studies of fear as an acquirable drive fear as motivation and fear-reduction as reinforcement in the learning of new responses. J. Exp. Psychol. 38, 89–101. doi:10.1037/h0058455
Moscarello, J. M., and LeDoux, J. E. (2013). Active avoidance learning requires prefrontal suppression of amygdala-mediated defensive reactions. J. Neurosci. 33, 3815–3823. doi:10.1523/JNEUROSCI.2596-12.2013
Pubmed Abstract | Pubmed Full Text | CrossRef Full Text | Google Scholar
Mowrer, O. H., and Lamoreaux, R. R. (1946). Fear as an intervening variable in avoidance conditioning. J. Comp. Psychol. 39, 29–50. doi:10.1037/h0060150
Oleson, E. B., Gentry, R. N., Chioma, V. C., and Cheer, J. F. (2012). Subsecond dopamine release in the nucleus accumbens predicts conditioned punishment and its successful avoidance. J. Neurosci. 32, 14804–14808. doi:10.1523/JNEUROSCI.3087-12.2012
Pubmed Abstract | Pubmed Full Text | CrossRef Full Text | Google Scholar
Paton, J. J., Belova, M. A., Morrison, S. E., and Salzman, C. D. (2006). The primate amygdala represents the positive and negative value of visual stimuli during learning. Nature 439, 865–870. doi:10.1038/nature04490
Pubmed Abstract | Pubmed Full Text | CrossRef Full Text | Google Scholar
Poremba, A., and Gabriel, M. (1997). Amygdalar lesions block discriminative avoidance learning and cingulothalamic training-induced neuronal plasticity in rabbits. J. Neurosci. 17, 5237–5244.
Poremba, A., and Gabriel, M. (1999). Amygdala neurons mediate acquisition but not maintenance of instrumental avoidance behavior in rabbits. J. Neurosci. 19, 9635–9641.
Roozendaal, B., Koolhaas, J. M., and Bohus, B. (1993). The central amygdala is involved in conditioning but not in retention of active and passive shock avoidance in male rats. Behav. Neural Biol. 59, 143–149. doi:10.1016/0163-1047(93)90873-G
Pubmed Abstract | Pubmed Full Text | CrossRef Full Text | Google Scholar
Rouillard, C., and Freeman, A. S. (1995). Effects of electrical stimulation of the central nucleus of the amygdala on the in vivo electrophysiological activity of rat nigral dopaminergic neurons. Synapse 21, 348–356. doi:10.1002/syn.890210410
Pubmed Abstract | Pubmed Full Text | CrossRef Full Text | Google Scholar
Salamone, J. D. (1994). The involvement of nucleus accumbens dopamine in appetitive and aversive motivation. Behav. Brain Res. 61, 117–133. doi:10.1016/0166-4328(94)90153-8
Pubmed Abstract | Pubmed Full Text | CrossRef Full Text | Google Scholar
Savonenko, A., Werka, T., Nikolaev, E., Zieliñski, K., and Kaczmarek, L. (2003). Complex effects of NMDA receptor antagonist APV in the basolateral amygdala on acquisition of two-way avoidance reaction and long-term fear memory. Learn. Mem. 10, 293–303. doi:10.1101/lm.58803
Pubmed Abstract | Pubmed Full Text | CrossRef Full Text | Google Scholar
Segura-Torres, P., Aldavert-Vera, L., Gatell-Segura, A., Redolar-Ripoll, D., and Morgado-Bernal, I. (2010). Intracranial self-stimulation recovers learning and memory capacity in basolateral amygdala-damaged rats. Neurobiol. Learn. Mem. 93, 117–126. doi:10.1016/j.nlm.2009.09.001
Pubmed Abstract | Pubmed Full Text | CrossRef Full Text | Google Scholar
Shumake, J., and Gonzalez-Lima, F. (2013). Functional opposition between habenula metabolism and the brain reward system. Front. Hum. Neurosci. 7:662. doi:10.3389/fnhum.2013.00662
Shumake, J., Ilango, A., Scheich, H., Wetzel, W., and Ohl, F. W. (2010). Differential neuromodulation of acquisition and retrieval of avoidance learning by the lateral habenula and ventral tegmental area. J. Neurosci. 30, 5876–5883. doi:10.1523/JNEUROSCI.3604-09.2010
Pubmed Abstract | Pubmed Full Text | CrossRef Full Text | Google Scholar
Singer, P., Wei, C. J., Chen, J. F., Boison, D., and Yee, B. K. (2013). Deletion of striatal adenosine A(2A) receptor spares latent inhibition and prepulse inhibition but impairs active avoidance learning. Behav. Brain Res. 242, 54–61. doi:10.1016/j.bbr.2012.12.024
Pubmed Abstract | Pubmed Full Text | CrossRef Full Text | Google Scholar
Smith, D. M., Monteverde, J., Schwartz, E., Freeman, J. H. Jr., and Gabriel, M. (2001). Lesions in the central nucleus of the amygdala: discriminative avoidance learning, discriminative approach learning, and cingulothalamic training-induced neuronal activity. Neurobiol. Learn. Mem. 76, 403–425. doi:10.1006/nlme.2001.4019
Pubmed Abstract | Pubmed Full Text | CrossRef Full Text | Google Scholar
Stamatakis, A. M., Jennings, J. H., Ung, R. L., Blair, G. A., Weinberg, R. J., Neve, R. L., et al. (2013). A unique population of ventral tegmental area neurons inhibits the lateral habenula to promote reward. Neuron 80, 1039–1053. doi:10.1016/j.neuron.2013.08.023
Pubmed Abstract | Pubmed Full Text | CrossRef Full Text | Google Scholar
Stamatakis, A. M., and Stuber, G. D. (2012). Activation of lateral habenula inputs to the ventral midbrain promotes behavioral avoidance. Nat. Neurosci. 15, 1105–1107. doi:10.1038/nn.3145
Pubmed Abstract | Pubmed Full Text | CrossRef Full Text | Google Scholar
Stark, H., Bischof, A., and Scheich, H. (1999). Increase of extracellular dopamine in prefrontal cortex of gerbils during acquisition of the avoidance strategy in the shuttle-box. Neurosci. Lett. 264, 77–80. doi:10.1016/S0304-3940(99)00174-3
Pubmed Abstract | Pubmed Full Text | CrossRef Full Text | Google Scholar
Stark, H., Bischof, A., Wagner, T., and Scheich, H. (2001). Activation of the dopaminergic system of medial prefrontal cortex of gerbils during formation of relevant associations for the avoidance strategy in the shuttle-box. Prog. Neuropsychopharmacol. Biol. Psychiatry 25, 409–426. doi:10.1016/S0278-5846(00)00171-8
Pubmed Abstract | Pubmed Full Text | CrossRef Full Text | Google Scholar
Stark, H., Rothe, T., Wagner, T., and Scheich, H. (2004). Learning a new behavioral strategy in the shuttle-box increases prefrontal dopamine. Neuroscience 126, 21–29. doi:10.1016/j.neuroscience.2004.02.026
Pubmed Abstract | Pubmed Full Text | CrossRef Full Text | Google Scholar
Stuber, G. D., Hnasko, T. S., Britt, J. P., Edwards, R. H., and Bonci, A. (2010). Dopaminergic terminals in the nucleus accumbens but not the dorsal striatum corelease glutamate. J. Neurosci. 30, 8229–8233. doi:10.1523/JNEUROSCI.1754-10.2010
Pubmed Abstract | Pubmed Full Text | CrossRef Full Text | Google Scholar
Tritsch, N. X., Ding, J. B., and Sabatini, B. L. (2012). Dopaminergic neurons inhibit striatal output through non-canonical release of GABA. Nature 490, 262–266. doi:10.1038/nature11466
Pubmed Abstract | Pubmed Full Text | CrossRef Full Text | Google Scholar
Tye, K. M., and Janak, P. H. (2007). Amygdala neurons differentially encode motivation and reinforcement. J. Neurosci. 27, 3937–3945. doi:10.1523/JNEUROSCI.5281-06.2007
Pubmed Abstract | Pubmed Full Text | CrossRef Full Text | Google Scholar
Tye, K. M., Stuber, G. D., de Ridder, B., Bonci, A., and Janak, P. H. (2008). Rapid strengthening of thalamo-amygdala synapses mediates cue-reward learning. Nature 453, 1253–1257. doi:10.1038/nature06963
Pubmed Abstract | Pubmed Full Text | CrossRef Full Text | Google Scholar
Wendler, E., Gaspar, J. C., Ferreira, T. L., Barbiero, J. K., Andreatini, R., Vital, M. A., et al. (2014). The roles of the nucleus accumbens core, dorsomedial striatum, and dorsolateral striatum in learning: performance and extinction of Pavlovian fear-conditioned responses and instrumental avoidance responses. Neurobiol. Learn. Mem. 109, 27–36. doi:10.1016/j.nlm.2013.11.009
Pubmed Abstract | Pubmed Full Text | CrossRef Full Text | Google Scholar
Wietzikoski, E. C., Boschen, S. L., Miyoshi, E., Bortolanza, M., Dos Santos, L. M., Frank, M., et al. (2012). Roles of D1-like dopamine receptors in the nucleus accumbens and dorsolateral striatum in conditioned avoidance responses. Psychopharmacology (Berl.) 219, 159–169. doi:10.1007/s00213-011-2384-3
Pubmed Abstract | Pubmed Full Text | CrossRef Full Text | Google Scholar
Wilson, J. R., Mitchell, J. C., and Van Hoesen, G. W. (1972). Epithalamic and ventral tegmental contributions to avoidance behavior in rats. J. Comp. Physiol. Psychol. 78, 442–449. doi:10.1037/h0032371
Keywords: punishment, active avoidance, safety signal, neural circuits, dopamine, amygdala, striatum
Citation: Ilango A, Shumake J, Wetzel W and Ohl FW (2014) Contribution of emotional and motivational neurocircuitry to cue-signaled active avoidance learning. Front. Behav. Neurosci. 8:372. doi: 10.3389/fnbeh.2014.00372
Received: 29 June 2014; Accepted: 08 October 2014;
Published online: 27 October 2014.
Edited by:
Richard J. Servatius, Department of Veterans Affairs Medical Center, USAReviewed by:
Amy Poremba, University of Iowa, USAClaudio Da Cunha, Universidade Federal do Paraná, Brazil
Copyright: © 2014 Ilango, Shumake, Wetzel and Ohl. This is an open-access article distributed under the terms of the Creative Commons Attribution License (CC BY). The use, distribution or reproduction in other forums is permitted, provided the original author(s) or licensor are credited and that the original publication in this journal is cited, in accordance with accepted academic practice. No use, distribution or reproduction is permitted which does not comply with these terms.
*Correspondence: antonilango@hotmail.com; shumake@austin.utexas.edu