- 1Department of Physiological Sciences, Center for Neuroscience and Cardiovascular Research, Biological Sciences Institute, Federal University of Goiás, Goiânia, Brazil
- 2Postgraduate Programme in Pharmaceutical Sciences, Faculty of Pharmacy, Federal University of Goiás, Goiânia, Brazil
- 3Department of Biochemistry and Molecular Biology, Biological Sciences Institute, Federal University of Goiás, Goiânia, Brazil
- 4Department of Morphology, Biological Sciences Institute, Federal University of Goiás, Goiânia, Brazil
- 5Department of Physiology, College of Physical Education, Federal University of Uberlândia, Uberlândia, Brazil
- 6Department of Physiology and Pathology, School of Dentistry, São Paulo State University, Araraquara, Brazil
Intrathecal injection of bombesin (BBS) promoted hypertensive and sympathoexcitatory effects in normotensive (NT) rats. However, the involvement of rostral ventrolateral medulla (RVLM) in these responses is still unclear. In the present study, we investigated: (1) the effects of BBS injected bilaterally into RVLM on cardiorespiratory and sympathetic activity in NT and spontaneously hypertensive rats (SHR); (2) the contribution of RVLM BBS type 1 receptors (BB1) to the maintenance of hypertension in SHR. Urethane-anesthetized rats (1.2 g · kg−1, i.v.) were instrumented to record mean arterial pressure (MAP), diaphragm (DIA) motor, and renal sympathetic nerve activity (RSNA). In NT rats and SHR, BBS (0.3 mM) nanoinjected into RVLM increased MAP (33.9 ± 6.6 and 37.1 ± 4.5 mmHg, respectively; p < 0.05) and RSNA (97.8 ± 12.9 and 84.5 ± 18.1%, respectively; p < 0.05). In SHR, BBS also increased DIA burst amplitude (115.3 ± 22.7%; p < 0.05). BB1 receptors antagonist (BIM-23127; 3 mM) reduced MAP (–19.9 ± 4.4 mmHg; p < 0.05) and RSNA (−17.7 ± 3.8%; p < 0.05) in SHR, but not in NT rats (−2.5 ± 2.8 mmHg; −2.7 ± 5.6%, respectively). These results show that BBS can evoke sympathoexcitatory and pressor responses by activating RVLM BB1 receptors. This pathway might be involved in the maintenance of high levels of arterial blood pressure in SHR.
Introduction
Bombesin (BBS), a tetradecapeptide isolated from the skin of the frog Bombina bombina (Anastasi et al., 1971), have shown broad spectrum of biological activities (Brown, 1983; Gonzalez et al., 2008; Jensen et al., 2008). The BBS activates three G protein-coupled receptors: bombesin receptor 1 (BB1), bombesin receptor 2 (BB2), and bombesin receptor 3 (BB3). BBS-like peptides—Neuromedin B (NB) and gastrin releasing peptide (GRP) are natural ligand of the BB1 and BB2 receptors, respectively (Jensen et al., 2008). Natural agonist of the BB3 receptor still remains unknown. However, it seems that BB3 receptor plays an important physiological role, since BB3 receptor knockout mice developed obesity associated with hypertension and impairment of glucose metabolism (Ohki-Hamazaki et al., 1997). In human, the BB1 receptor gene is at chromosome 6p21-pter (Jensen et al., 2008). The BB1 receptor signal occurs primarily through phospholipase-C-mediated cascades, that involve activation of Gqα protein and consequent stimulation of protein kinase C (Jensen et al., 2008).
In mammals, BBS receptors and BBS-like peptides are distributed in the Central Nervous System (CNS) (Woodruff et al., 1996; Jensen et al., 2008) including regions involved in the cardiorespiratory control (Chung et al., 1989; Lynn et al., 1996; Li et al., 2016). The administration of BBS has been reported to enhancebreathing (Holtman et al., 1983; Glazkova and Inyushkin, 2006), raise plasma concentration of catecholamine (Brown and Fisher, 1984), tachycardia (Zogovic and Pilowsky, 2011), increase blood pressure (Brown, 1983; Zogovic and Pilowsky, 2011), and sympathetic tone (Zogovic and Pilowsky, 2011) in normotensive (NT) rats. Zogovic and Pilowsky (2011) showed that intrathecal injection of BBS is associated with sympathoexcitatory and pressor responses. In the same study, the authors also reported that the administration of an antagonist of BBS receptor 2 attenuated the effects of BBS on blood pressure of NT rats. However, the involvement of the rostral ventral medulla (RVLM) in the BBS-induced cardiorespiratory and autonomic responses as well as the contribution of the BBS receptor type 1 to the maintenance of blood pressure in NT rats and spontaneously hypertensive rats (SHR) is still unclear.
The RVLM contains neurons that regulate peripheral sympathetic vasomotor tone and blood pressure (Guertzenstein, 1973; Guertzenstein and Silver, 1974; Guyenet et al., 1989; Guyenet, 2006; Toney and Stocker, 2010). The RVLM is localized ventral to the rostral part of the nucleus ambigus (NA), caudal to the facial nucleus and ventral to the Bötzinger complex. The RVLM neurons project to the sympathetic preganglionic neurons located in the intermediolateral (IML) cell column of the spinal cord (Loewy, 1981; Millhorn and Eldridge, 1986; Guyenet, 2006). The neurons projecting from RVLM could modulate peripheral sympathetic activity to the kidneys, vessels, heart, and adrenal gland.
The hyperactivity of RVLM neurons has been implicated in the maintenance of hypertension in different experimental models (Yang et al., 1995; Fink, 1997; Ito et al., 2000, 2003; Matsuura et al., 2002; Adams et al., 2007; Stocker et al., 2007; Toney and Stocker, 2010). Previous studies have shown that the injection of excitatory amino acid (EAA) antagonist into the RVLM reduced arterial pressure in SHR but not in NT rats (Ito et al., 2000). In addition, the electrophysiological studies have shown that firing rate of RVLM neurons is significantly faster in neonatal and adult SHR than NT rats (Chan et al., 1991; Matsuura et al., 2002). These findings indicate that hyperactivity of the RVLM neurons could contribute to the development and maintenance of hypertension in SHR.
Hence, we hypothesized whether or not the injection of BBS into RVLM affects cardiorespiratory and sympathetic activities in NT rats and SHR. In order to test this hypothesis, the recording of the mean arterial pressure (MAP), renal sympathetic nerve activity (RSNA) and diaphragm (DIA) motor activity were carried out to evaluate changes induced by unilateral and bilateral injection of BBS into RVLM of urethane-anesthetized NT rats and SHR. In addition, the contribution of tonic activation of BBS receptor 1 in the maintenance of high levels of arterial pressure in SHR was also evaluated.
Methods
Animals and Ethical Approval
Male Wistar NT rats and SHR weighing 250 to 330 g were used. Animals were housed in a temperature-controlled room (22–24°C) with a 12:12-h light-dark cycle (lights on at 07:00), free access to food, and tap water. All experimental procedures were designed in strict adherence to the National Health Institute Guidelines for Care and Use of Laboratory Animals, and approved by Ethics Committee for Animal Care and Use (CEUA) of the Federal University of Goiás (number of ethical committee: 025/12).
Surgical Procedures
Rats were anesthetized with halothane (2–3% in O2; Tanohalo; Cristália, SP, Brazil). The right femoral vein and artery were catheterized for drug administration and blood pressure recording, respectively. After catheterization, anesthesia was maintained by intravenous administration of urethane (1.2 g · kg−1 b.wt.; Sigma–Aldrich, St. Louis, MO, USA). The trachea was cannulated to reduce airway resistance. Bipolar stainless steel electrodes were implanted in the DIA muscle for electromyography (EMG) recording of inspiratory motor activity. Rats were later mounted prone in a stereotaxic apparatus for craniotomy and instrumented for the recording of RSNA. The body temperature of rats was maintained at 37 ± 0.5°C with a thermostatically controlled heated table.
Recording of Cardiorespiratory Parameters
In order to record the arterial pressure, the arterial catheter was connected to a pressure transducer which is coupled to an amplifier (Bridge Amp FE221; ADInstruments, Colorado Springs, CO, USA). The pulsatile pressure was recorded continuously with a data acquisition system (PowerLab; ADInstruments, Colorado Springs, CO, USA). The MAP was calculated from the pulsatile signal using the LabChart software (v.7.3.7, ADInstruments, Colorado Springs, CO, USA). Analogical signals of the electrocardiogram (ECG), obtained through electrodes positioned in the forelimbs, were amplified 1000 times and filtered between 100 and 1000 Hz (Bridge Amp; ADInstruments, Colorado Springs, CO, USA). The heart rate (HR) was calculated as instantaneous frequency of the ECG signal (LabChart v.7.3.7, ADInstruments, Colorado Springs, CO, USA). The DIA motor activity signals was amplified 10,000 times (Bridge Amp; ADInstruments, Colorado Springs, CO, USA) and band-pass filtered (100–2000 Hz). The signal were rectified and integrated in 50 ms intervals using LabChart software (v.7.3.7; ADInstruments, Colorado Springs, CO, USA). The DIA motor activity was evaluated by burst amplitude (expressed as percentage difference from baseline) and frequency (considered as respiratory frequency, fR, and expressed in cycles per minute, cpm).
Recording of Renal Sympathetic Nerve Activity
RSNA was recorded through the left renal nerve with bipolar silver electrodes. The renal nerve was located, dissected and covered with mineral oil prior to the placement of electrodes for recording (Nujol - Schering-Plough, São Paulo, SP, Brazil). The signals were obtained using a high-impedance probe connected to the amplifier (P511; Grass Instruments, Quincy, MA, USA). The signal was amplified 20,000 times, digitized and band-pass filtered (30–1000 Hz). The nerve signal was recorded continuously (with a PowerLab System-ADInstruments; Colorado Springs, CO, USA), rectified and integrated at 1 s intervals using LabChart software (v.7.3.7.; ADInstruments; Colorado Springs, CO, USA). At the end of each experiment, ganglionic blocker hexamethonium (30 mg · kg−1, b.wt., i.v.; Sigma–Aldrich, St. Louis, MO, USA) was administered to determine the background noise. The level of RSNA was expressed as a percentage of baseline after subtraction of the noise.
Respiratory Synchronization
Analyses of the respiratory synchronization were made offline using Spike2 software (version 8; Cambridge Electronic Design Limited, Cambridge, CAM, England). In order to analyze respiratory modulation, RSNA was rectified and signals were smoothed using a time constant of 50 ms. The DIA-triggered averages of RSNA were generated after nanoinjection of vehicle and bilateral BBS into RVLM. Averages of RSNA were made using 15 DIA burst as trigger events. The time of inspiration was determined based on the duration of the inspiratory DIA burst while expiratory time was determined between consecutive DIA burst. The RSNA post-inspiratory peak was later evaluated.
Nanoinjections into RVLM
Animals were mounted prone in a stereotaxic apparatus (David Kopf Instruments, Tujunga, CA, USA) with incisor bar 11 mm below the interaural line. After partial removal of the occipital bone, the meninges covering the dorsal surface of the brainstem was opened up surgically to visualize the calamus scriptorius. In order to nanoinject into the RVLM, a glass micropipette was positioned as follows: 2.5 mm rostral from the calamus scriptorius, ±2.0 mm lateral from the midline and 2.5 mm ventral from the dorsal surface.
Firstly, RVLM was localized through unilateral nanoinjection (50 nl) of L-glutamate (10 mM; Sigma-Aldrich, St. Louis, MO, USA) in all experimental groups. RVLM was considered previously identified when L-glutamate nanoinjection increased MAP in approximately 20 mmHg. After the return of cardiorespiratory and sympathetic activities to basaline, the bilateral nanoinjection (50 nl each) of vehicle (150 mM NaCl; Sigma-Aldrich, St. Louis, MO, USA), BBS (0.3 mM; Bachem AG, Bubendorf, Switzerland) or bombesin receptor 1 (BB1) antagonist (3 mM; [D-2- NaI5-Cys6, 11-Tyr7,D-Trp8,Val10,2-NaI12-Somatostatin-14 (5–12) amide trifluoroacetate salt]; BIM-23127; Bachem AG, Bubendorf, Switzerland) was injected into RVLM. In order to confirm the integrity of RVML, L-glutamate (10 mM; 50 nl) was nanoinjected again. This nanoinjection produced similar increase in MAP (~20 mmHg) as previously observed in the initial L-glutamate nanoinjection.
Histology
At the end of the experiment, 2% Evans Blue solution (50 nl; Sigma-Aldrich, St. Louis, MO, USA) was nanoinjected bilaterally into RVLM for histological analyses with the aim of confirming the accuracy of injection sites. Rats were perfused transcardially with saline (150 mM NaCl; 300 mL), followed by 10% formaldehyde (300 mL; Synth Ltda, Diadema, SP, Brazil). The brains were later removed and fixed in 10% formaldehyde. Frozen brains were cut into 40 μm coronal sections and stained with 1% neutral red to determine the nanoinjection sites.
Experimental Protocols
The MAP, DIA motor activity and RSNA were recorded (NT, n = 7; SHR, n = 7). After a period of stabilization, bilateral nanoinjections of BBS (0.3 mM in 50 nl each) or equivalent volume of vehicle (150 mM NaCl in 50 nl each) was nanoinjected into RVLM. In another group of NT rats (n = 6) and SHR (n = 6), BIM-23127 (BB1 receptor antagonist; 3 mM in 50 nl each) was nanoinjected bilaterally into RVLM. In order to confirm the blockade of BB1 receptors, in separated groups of NT rats (n = 3) and SHR (n = 6), unilateral nanoinjection of BBS (0.3 mM in 50 nl each) 15 min before and 15 min after BIM-23127 (3 mM in 50 nl each) was carried out.
Statistical Analysis
The GraphPad Prism software (v.6; GraphPad Software, Inc., La Jolla, CA, USA) was used for the statistical analysis of experimental data. The basal values and changes in the respiratory synchronization induced by BBS nanoinjection were compare between the groups using an unpaired and paired Student's t-test. The autonomic and cardiovascular effects induced by nanoinjection of vehicle, BBS, and BIM into the RVLM were analyzed using two-way ANOVA prior to the Newman–Keuls test. The value of p < 0.05 was considered statistically significant.
Results
Histological Analysis
Figure 1A show a representative photomicrograph of the brainstem section that indicates the accuracy of nanoinjection site—RVLM. The center of the nanoinjection site distributions at rostral and caudal levels of RVLM are shown in Figure 1B. Only animal with RVLM confined nanoinjections were analyzed.
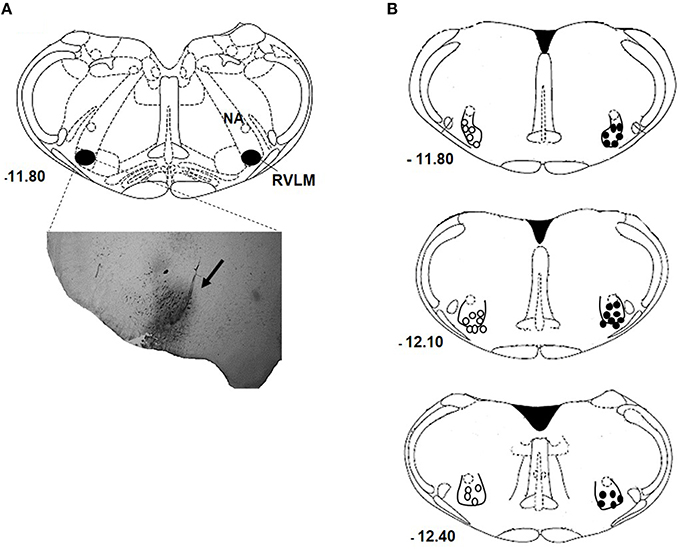
Figure 1. RVLM nanoinjection site. Photomicrograph of a coronal section of the medulla showing a representative site of injection labeled with 4% Evans Blue dye into RVLM (arrow; A). Schematic drawing showing the center of the nanoinjection site to the bregma in normotensive (open circles) and hypertensive (closed circles) rats nanoinjected with either bombesin (0.3 mM) or BIM-23127 (3 mM; B), and rats that received unilateral nanoinjection of bombesin before and after BIM-23127. NA, nucleus ambiguous.
Cardiorespiratory and Sympathetic Changes Produced by the Injections of BBS into RVLM
Table 1 shows the body weight and basal values of MAP, RSNA, and fR of NT rats and SHR. There were no changes in RSNA and body weight among groups. However, higher MAP and lower fR were observed in SHR when compared with NT rats (p < 0.05).
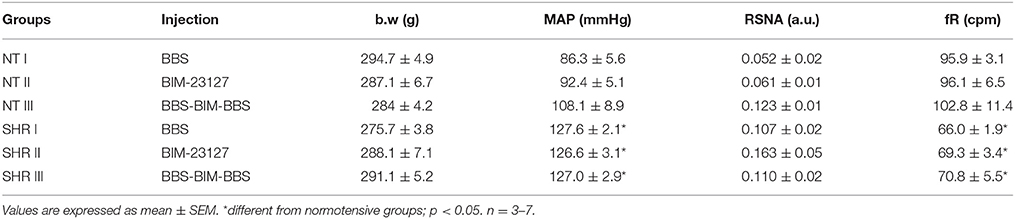
Table 1. Body weight (b.w) and basal values of mean arterial pressure (MAP), renal sympathetic nerve activity (RSNA), and respiratory frequency (fR) of normotensive (NT) and spontaneously hypertensive rats (SHR) that received nanoinjections of bombesin (BBS; 0.3 mM) or BIM-23127 (3 mM) into RVLM.
Unilateral nanoinjection of L-glutamate into RVLM increased MAP in both NT rats (ΔMAP: 21.6 ± 5.6 mmHg vs. vehicle −0.7 ± 0.5 mmHg; p < 0.05) and SHR (26.3 ± 6.3 mmHg vs. vehicle 0.4 ± 0.5 mmHg; p < 0.05; Figures 2, 3A). The injection of L-glutamate did not elicit significant alteration in RSNA, DIA burst amplitude and fR in NT rats (ΔRSNA: 28.8 ± 5.2% vs. vehicle −0.3 ± 1.1%; ΔDIA Burst Amp: −19.9 ± 15.3% vs. vehicle −0.5 ± 1.7%; ΔfR: −6.7 ± 8,2 cpm vs. vehicle −2,5 ± 1.8 cpm; Figures 2, 3C,D) and SHR (ΔRSNA: 40.0 ± 3.2% vs. vehicle 1.4 ± 0.6%; ΔDIA Burst Amp: 10.7 ± 12% vs. vehicle −3.5 ± 1.6%; ΔfR: −3.5 ± 2.4 cpm vs. vehicle −0.03 ± 1.2 cpm; Figures 2, 3B–D).
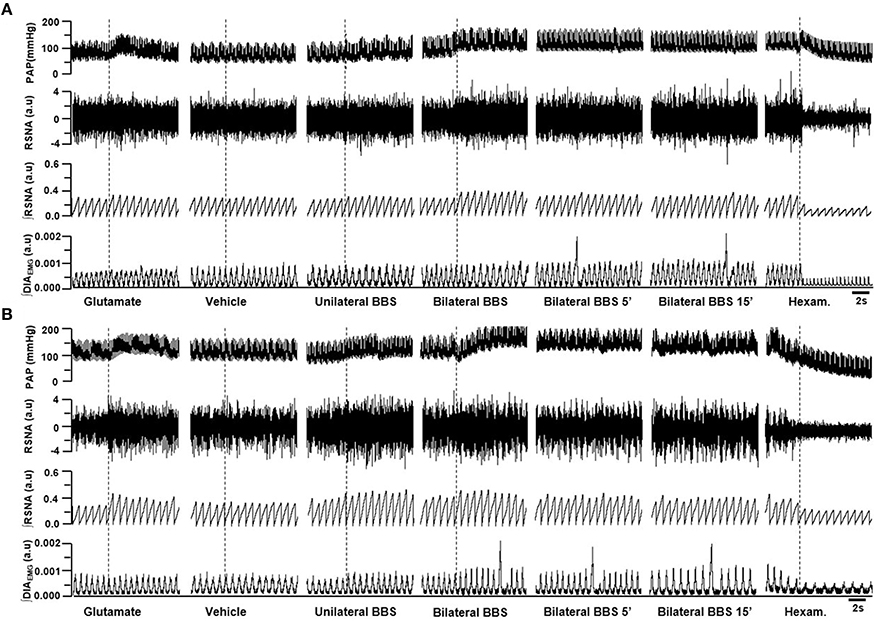
Figure 2. Examples showing the cardiorespiratory and sympathetic effects induced by BBS into RVLM. Digitized record of cardiovascular, sympathetic, and respiratory responses produced by unilateral nanoinjections of L-glutamate (10 mM; 50 nl), vehicle (150 mM NaCl), unilateral bombesin (BBS; 0.3 mM), bilateral bombesin (BBS; 0.3 mM; 1, 5, and 15 min) into RVLM of normotensive (A) and hypertensive rats (B). Pulsatile arterial pressure (PAP), renal sympathetic nerve activity (RSNA), integrate of renal sympathetic nerve activity (∫RSNA), integrate of diaphragm motor activity (∫DIAEMG) and hexam (hexamethonium).
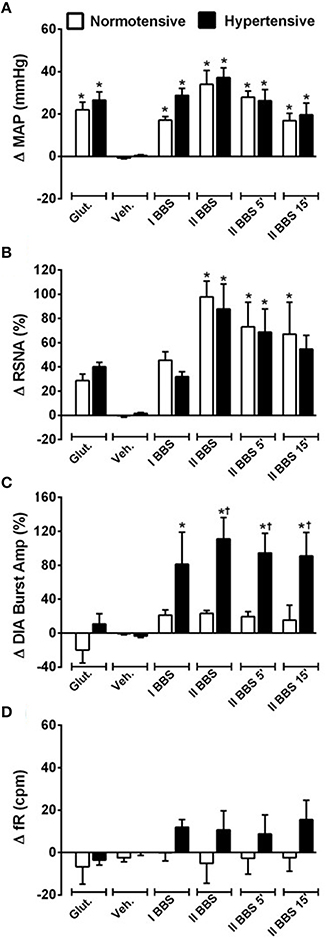
Figure 3. Cardiovascular, sympathetic, and respiratory responses induced by bombesin naoinjected into RVLM. Changes induced by unilateral nanoinjecton of L-glutamate (Glut, 10 mM; 50 nl), vehicle (Veh, 150 mM NaCl), bombesin (I BBS, 0.3 mM), and bilateral nanoinjection of bombesin (II BBS; 0.3 mM; 1, 5, and 15 min) into RVLM on mean arterial pressure (MAP; A), renal sympathetic nerve activity (RSNA; B), diaphragm burst amplitude (DIA Burst Amp; C) and respiratory frequency (fR; D) in normotensive and hypertensive rats. *different from vehicle; †different from normotensive rats. p < 0.05; n = 7.
Unilateral nanoinjection of BBS increased MAP in NT rats (17.1 ± 1.7 mmHg vs. vehicle −0.7 ± 0.3 mmHg; p < 0.05; Figures 2A, 3A) without significant change in RSNA (45.5 ± 6.9% vs. vehicle −0.3 ± 1.1%; Figures 2A, 3B), DIA burst amplitude (21.3 ± 6.1% vs. vehicle −0.5 ± 1.7%; Figures 2A, 3C) and fR (−0.1 ± 3.7 cpm vs. vehicle −2.5 ± 1.8 cpm; Figures 2A, 3D). In SHR, unilateral nanoinjection of BBS increased MAP (28.8 ± 3.2 mmHg vs. vehicle 0.3 ± 0.4 mmHg; p < 0.05; Figures 2B, 3A) without significant alteration in RSNA (31.8 ± 3.7% vs. vehicle 1.4 ± 0.6%; Figures 2B, 3B) and fR (11.9 ± 3.5 cpm vs. vehicle 0.0 ± 1.2 cpm; Figures 2B, 3D). Unilateral nanoinjection of BBS enhanced DIA burst amplitude (81.2 ± 37.9% vs. vehicle −3.5 ± 1.5%; p < 0.05; Figures 2B, 3C).
In NT rats, bilateral nanoinjection of BBS increased MAP (33.9 ± 6.6; 27.8 ± 2.9; 16.8 ± 3.4 mmHg vs. vehicle −0.7 ± 0.3 mmHg at 1, 5, and 15 min, respectively; p < 0.05; Figures 2A, 3A) and RSNA (97.8 ± 12.9; 73.1 ± 23.2; 66.9 ± 30.2% vs. vehicle −0.3 ± 1.1%, at 1, 5, and 15 min, respectively; p < 0.05; Figures 2A, 3B). An increase in MAP (37.1 ± 4.6; 26.2 ± 5.2; 19.5 ± 5.5 mmHg vs. vehicle 0.3 ± 0.4 mmHg, at 1, 5, and 15 min, respectively; p < 0.05; Figures 2B, 3A) and RSNA (87.9 ± 18.1; 68.7 ± 16.8% vs. vehicle 1.4 ± 0.6%, at 1 and 5 min, respectively; p < 0.05; Figures 2B, 3B) were observed in SHR.
The DIA burst amplitude was not altered by bilateral nanoinjections of BBS in NT rats (23.2 ± 3.3; 19.3 ± 6.0; 15.3 ± 17.3% vs. vehicle −0.5 ± 1.7%; at 1, 5, and 15 min, respectively; Figures 2A, 3C). On the other hand, bilateral nanoinjections of BBS increased DIA burst amplitude in SHR (111.0 ± 25.3; 94.5 ± 23.1; 90.9 ± 27.7% vs. vehicle −3.5 ± 1.5%; at 1, 5, and 15 min, respectively; p < 0.05; Figures 2B, 3C). The bilateral nanoinjection of BBS did not induce significant changes in fR of NT rats (−5.1 ± 9.3; −2.6 ± 7.4 cpm; −2.4 ± 6.4 vs. vehicle −2.5 ± 1.8 cpm; at 1, 5, and 15 min, respectively; Figures 2A, 3D) and SHR (10.5 ± 9.1; 8.7 ± 8.9; 15.4 ± 9.2 cpm vs. vehicle 0.0 ± 1.2 cpm; at 1, 5, and 15 min, respectively; Figures 2B, 3D).
The unilateral nanoinjection of BBS did not elicit significant alteration in HR of NT rats (−14.5 ± 4.9 bpm vs. vehicle −3.2 ± 1.6 bpm). The bilateral nanoinjection of BBS decreased HR (−30.1 ± 7.3 bpm; −27.0 ± 6.8 bpm; −23.9 ± 6.8 bpm vs. vehicle −3.2 ± 1.6 bpm; at 1, 5, and 15 min, respectively; p < 0.05). In SHR, the unilateral (18.4 ± 3.8 bpm vs. vehicle −8.5 ± 1.9 bpm; p < 0.05) and bilateral nanoinjections of BBS (26.3 ± 7.0 bpm; 27.4 ± 7.7 bpm; 25.8 ± 8.2 bpm vs. vehicle −8.5 ± 1.9 bpm; at 1, 5, and 15 min; respectively; p < 0.05) increased HR.
Synchronization of Sympathetic Discharge During the Respiratory Cycle after Bilateral Nanoinjection of BBS into RVLM
The BBS nanoinjected into RVLM elicited an increase in post-inspiratory RSNA peak in SHR (0.309 ± 0.07 a.u vs. vehicle 0.180 ± 0.05 a.u.; p < 0.05; Figure 4B) but not in NT rats (0.227 ± 0.06 a.u vs. vehicle 0.103 ± 0.05 a.u.; Figure 4A).
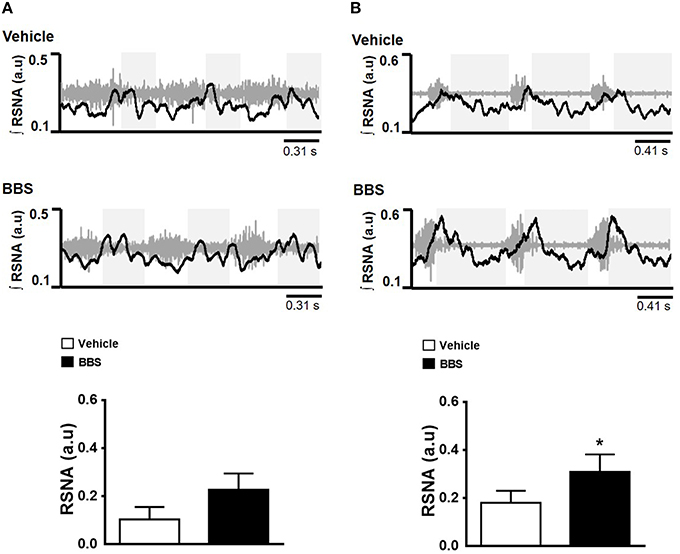
Figure 4. Effects of bilateral nanoinjection of bombesin into RVLM on respiratory synchronization of RSNA. Respiratory modulation after bilateral nanoinjection of vehicle (150 mM NaCl) and bombesin (BBS; 0.3 mM) into RVLM of normotensive (A) and hypertensive rats (B). Examples show three respiratory cycles. The gray boxes show the expiratory phase. Maximum amplitude of renal sympathetic nerve activity (RSNA) during post-inspiratory are present in the graphics. *Different from vehicle. p < 0.05; n = 7.
Cardiorespiratory and Sympathetic Changes Produced by BB1 Receptor Blockade in the RVLM Neurons in NT Rats and SHR
The bilateral nanoinjection of BIM-23127 into RVLM did not produce significant changes in MAP (−2.2 ± 2.8; −1.4 ± 2.7; −2.4 ± 1.9 mmHg vs. vehicle 1.0 ± 1.0 mmHg, at 1, 5, and 15 min, respectively; Figures 5A, 6A), RSNA (−2.9 ± 2.5; −3.4 ± 2.7; −3.3 ± 2.3% vs. vehicle 0.0 ± 0.9% at 1, 5, and 15 min, respectively; Figures 5A, 6B), DIA burst amplitude (−0.7 ± 3.5; −0.5 ± 3.1; −3.7 ± 3.5% vs. vehicle 2.1 ± 3.5% at 1, 5, and 15 min, respectively; Figures 5A, 6C) and fR (−0.1 ± 1.9; −4.0 ± 4.2; −7.8 ± 4.1 cpm vs. vehicle −1.1 ± 1.1 cpm at 1, 5, and 15 min, respectively; Figures 5A, 6D) in NT rats.
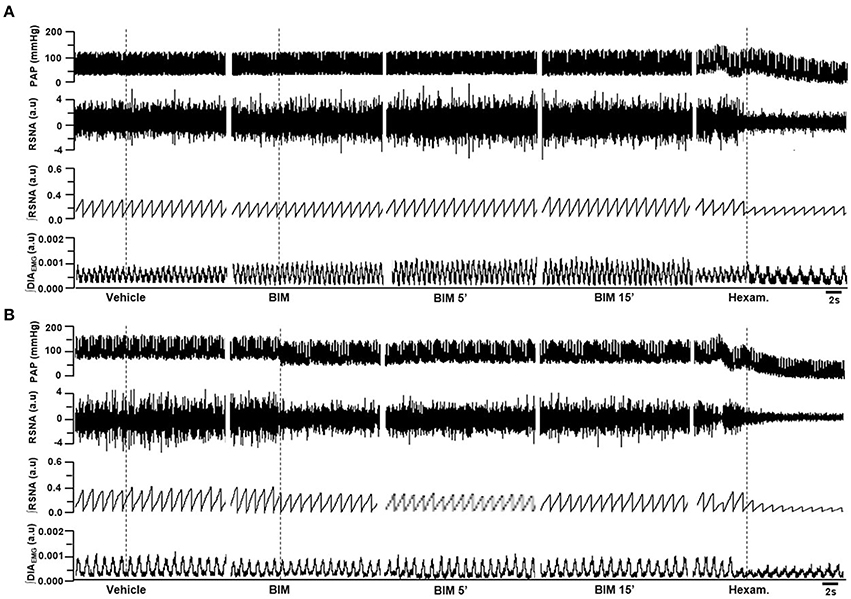
Figure 5. Examples showing the cardiorespiratory and sympathetic effects induced by BB1 receptors blockade in the RVLM. Digitized record of cardiovascular, sympathetic and respiratory responses produced by vehicle (150 mM NaCl) and bilateral nanoinjection of BIM-23127 (3 mM; BB1 receptor antagonist; 1, 5, and 15 min after BIM) into RVLM in normotensive (A) rats and hypertensive rats (B). Pulsatile arterial pressure (PAP), renal sympathetic nerve activity (RSNA), integrate of renal sympathetic nerve activity (∫RSNA) and integrate of diaphragm motor activity (∫ DIAEMG) and hexam (hexamethonium).
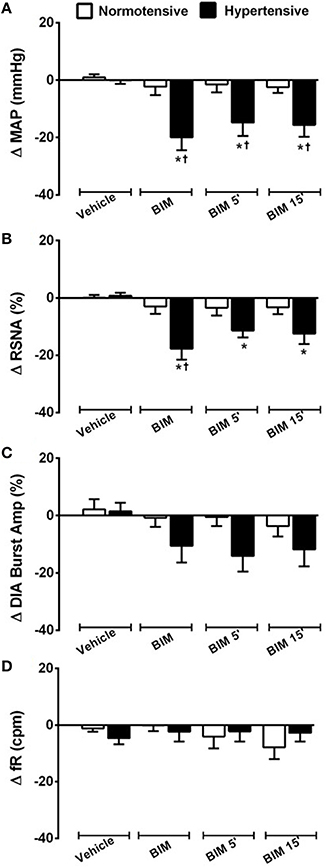
Figure 6. Cardiovascular, sympathetic, and respiratory responses induced by BB1 receptors blockade into RVLM. Changes produced by bilateral nanoinjecton of vehicle (150 mM NaCl) and BIM-23127 (3 mM; BB1 receptor antagonist; 1, 5, and 15 min) into RVLM on mean arterial pressure (MAP; A), renal sympathetic nerve activity (RSNA; B), diaphragm burst amplitude (DIA Burst Amp; C), and respiratory frequency (fR; D) in normotensive and hypertensive rats. *different from vehicle; †different from normotensive rats. p < 0.05; n = 6.
In SHR, bilateral nanoinjection of BIM-23127 reduced MAP (−19.9 ± 4.4; −14.8 ± 4.6; −15.6 ± 4.1 mmHg vs. vehicle −0.0 ± 1.2 mmHg, at 1, 5, and 15 min respectively; p < 0.05; Figures 5B, 6A) and RSNA (−17.7 ± 3.8; −11.3 ± 2.4; −12.4 ± 3.5% vs. vehicle 0.7 ± 1.0% at 1, 5, and 15 min, respectively; p < 0.05; Figures 5B, 6B). No significant changes were observed in the DIA burst amplitude (−10.5 ± 5.9; –14.0 ± 5.4; −11.8 ± 5.9% vs. vehicle 1.5 ± 2.9% at 1, 5, and 15 min, respectively; Figures 5B, 6C) and fR (−2.3 ± 3.5; −2.2 ± 3.5; −2.7 ± 3.0 cpm vs. vehicle −4.6 ± 2.2 cpm at 1, 5, and 15 min, respectively; Figures 5B, 6D) in SHR.
The blockade of BB1 receptor did not alter HR in both NT rats (−3.8 ±3.0 bpm; −4.9 ± 2.4 bpm; −7.8 ± 2.6 bpm vs. vehicle 1.9 ± 1.6 bpm, at 1, 5, and 15 min, respectively) and SHR (−11.5 ±6.7 bpm; −6.1 ± 5.0 bpm; −5.7 ± 6.2 bpm vs. vehicle 0.4 ± 1.2 bpm, at 1, 5, and 15 min, respectively).
Cardiorespiratory and Sympathetic Responses to BBS Injected after Blockade of RVLM BB1 Receptors
Table 2 shows the cardiorespiratory and sympathetic changes induced by unilateral nanoinjection of BBS into RVLM before and after injection of BIM-23127 (BB1 receptor antagonist) in NT rats and SHR. In both groups, the BBS-induced increase in MAP and RSNA was abolished by BIM-23127. The significant changes in BBS-induced DIA burst amplitude was abolished by the blockade of BB1 receptors SHR.

Table 2. Changes in the mean arterial pressure (MAP), renal sympathetic nerve activity (RSNA) and diaphragm burst amplitude (DIA burst Amp) induced by unilateral injection of bombesin (BBS; 0.3 mM) into RVLM before and after BB1 receptor blockade with BIM-23127 (3 mM) in normotensive (NT) and spontaneously hypertensive (SHR) rats.
Discussion
Previous studies have shown that BBS increases blood pressure (Brown and Guyenet, 1985; Zogovic and Pilowsky, 2011) and sympathetic tone (Zogovic and Pilowsky, 2011). However, these studies did not established the effects of BBS in the brainstem and their role in the maintenance of hypertension. In the present study, we provided the first evidence that BBS acting in RVLM elicits a significant increase in MAP and RSNA of both NT rats and SHR. In addition, the administration of BBS into RVLM increased DIA burst amplitude and post-inspiratory RSNA burst in SHR. The blockade of BB1 receptors in the RVLM reduces MAP and RSNA in SHR but not in NT rats. These results strongly indicate that the sympathoexcitation associated with pressor response to BBS administration is mediated by BB1 receptors that are located on RVLM neurons. It has been suggested that tonic activation of BB1 receptors is involved in the maintenance of high arterial pressure in SHR.
Zogovic and Pilowsky (2011), showed a long-lasting increase in the splanchnic SNA (sSNA), blood pressure and phrenic nerve amplitude by the intrathecal injection of BBS. Glazkova and Inyushkin (2006) reported that microinjection of BBS in the solitary tract nucleus (NTS) stimulated respiration, and increased the level of pulmonary ventilation, respiratory volume, and bioelectrical activity of the inspiratory muscles. Our results showed that BBS injected into the RVLM increased arterial blood pressure and sympathetic vasomotor tone in both NT and SHR. This result suggests a modulatory action of BBS in RVLM neurons.
Recently, Li et al. (2016) showed that small neural subpopulation is involved in the control of breathing. The retrotrapezoid nucleus/parafacial respiratory group (RTN/pFRG) expresses neuromedin B (BBS-like peptide genes) and GRP. This neural subpopulation project to preBötzinger Complex (preBötC, the respiratory rhythm generator that expresses neuromedin B and GRP receptors). The vasomotor presympathetic neurons in all anteroposterior extension of RVLM are intercalated with ventral respiratory column neurons (Smith et al., 2007; Alheid and McCrimmon, 2008; Wang et al., 2009). As a result of the proximity with respiratory neurons, the BBS injection into the RVLM could activate neuromedin B or GRP receptors and contribute to the increase in respiratory drive that was observed in SHR.
The RVLM neurons play essential role in the generation of sympathetic outflow (Guertzenstein, 1973; Guyenet, 2006; Wang et al., 2009) and regulation of peripheral chemosensitive and barosensitive sympathetic efferents (Sun and Reis, 1995; Miyawaki et al., 1996; Dampney et al., 2002; Alheid and McCrimmon, 2008). The neuronal activity of the RVLM is determined by the action of excitatory and inhibitory synapses that involve glutamate and γ-aminobutyric acid (GABA) neurotransmitters, respectively (Sun and Reis, 1995; Miyawaki et al., 1996; Ito et al., 2000; Schreihofer et al., 2000; Alheid and McCrimmon, 2008). In addition, some neuropeptides have been reported to play important modulatory role in the integration cardiovascular responses (Ito et al., 2000; Alheid and McCrimmon, 2008; Abbott and Pilowsky, 2009).
Several studies have shown that the activation or blockade of neuropeptide receptors could cause a long-term response (Abbott and Pilowsky, 2009; Zogovic and Pilowsky, 2011). The nature of response can be explained (partly) by the receptor-ligand type. For instance, the sensitization of G-protein coupled receptors are related to a wide range of intracellular event cascades such as changes in ion channel permeability, activation of kinases, and protein phosphorylation (Springell et al., 2005a,b).
Zogovic and Pilowsky (2011) reported that intrathecal injection of BBS elicited gradual increase in MAP and splanchnic sympathetic nerve activity (sSNA) within 5 min. This increase returned to control level after approximately 35 min of BBS injection. In our study, we showed that BBS injection into RVLM caused a rapid increase in blood pressure. This increase persisted during 15 min of bilateral nanoinjection of BBS. The injection of BIM induced rapid decrease in the MAP and RSNA (the decrease was maintained during 15 min). Some authors have reported instantaneous response to BBS administration (Erspamer et al., 1972; Chahl and Walker, 1981; Bayorh and Feuerstein, 1985; Kaczynska and Szereda-Przestaszewska, 2011). Intravenous administration of BBS caused immediate increase in MAP of anesthetized animals (Erspamer et al., 1972; Kaczynska and Szereda-Przestaszewska, 2011). According to the author, this cardiovascular response appeared to be mediated via α-adrenergic receptors (Kaczynska and Szereda-Przestaszewska, 2011).
Experimental evidences have demonstrated that an increase in RVLM neuronal activity could contribute to the development and maintenance of hypertension in SHR (Smith and Barron, 1990; Yang et al., 1995; Matsuura et al., 2002; Ito et al., 2003). The bicuculline (GABAa receptor antagonist) injection into RVLM slightly increased the arterial blood pressure in SHR when compared to NT rats. This result suggest excessive excitatory drive of RVLM pre-sympathetic neurons in hypertensive rats (Smith and Barron, 1990). The inhibition of glutamatergic neurotransmission by kynurenic acid (KYN) injection into RVLM of SHR decreased the arterial pressure (Ito et al., 2000). Matsuura et al., (2002), showed that basal membrane potential in irregularly firing RVLM neurons is less negative in neonatal SHR. In consequence, the RVLM neurons in these animals are more easily excitable. The firing rate is faster in neonatal SHR when compared with NT rats. In our study, we demonstrated that the blockade of RVLM BB1 receptors decreased MAP and RSNA in SHR, but not in NT rats. These findings suggest that the activation of BB1 receptors in the RVLM could have contributed to the maintenance of high arterial pressure in SHR.
Our findings are consistent with previous studies, which showed a decrease blood pressure of anesthetized hypertensive rats as a result of pharmacological blockade of RVLM neurons (Bergamaschi et al., 1999, 2014; Ito et al., 2000, 2003; Suhaimi et al., 2010; Du et al., 2013). The BBS injection into RVLM, in the present study, induced similar increase in the MAP and RSNA of SHR when compared with NT rats. The anesthesia reduced MAP in hypertensive rats more than in NT controls. Unlike non-anesthetized rats, the subjection of rats to anesthesia is assumed to have prevented the some responses to BBS.
Our results showed that BBS injection into RVLM induced sympathoexcitation with an increase in the blood pressure of NT rats and SHR. This effect indicates that RVLM could integrate neuronal pathway that are involved in BBS induced cardiorespiratory and sympathetic effects. The blockade of BB1 receptors in the RVLM of SHR, but not NT rats, decreased MAP and RSNA. This result suggests that the tonic activation of BB1 receptors is involved in the maintenance of high blood pressure in anesthetized SHR. The changes in the cardiorespiratory and sympathetic activity as a result of an activation or inhibition of RVLM BBS receptors suggests an important cardiorespiratory role of BBS and related peptides.
Author Contributions
Conceived and designed the experiments: GP, CD, AF, AD, AR, DC. Performed the experiments: ID, AM, SM, AC, KG. Analyzed the data: ID, AM, ED, MF, JF, DC, EC, GP. Contributed reagents/materials/analysis tools: DC, CD, DR, EC, AF, AD, AR, GP. Wrote the paper: ID, AM, ED, MF, JF, DR, EC, AF, AR, GP.
Conflict of Interest Statement
The authors declare that the research was conducted in the absence of any commercial or financial relationships that could be construed as a potential conflict of interest.
Acknowledgments
This work was supported by Fundação de Amparo a Pesquisa do Estado de Goiás (FAPEG) grants 2012/0055431086 (GP) and 2009/10267000352 (GP) and by Conselho Nacional de Desenvolvimento Científico e Tecnológico (CNPq) grants 477832/2010-5 (GP), 483411/2012-4 (GP), and 447496/2014-0 (GP). The funders had no role in study design, data collection, analysis, decision to publish, or preparation of the manuscript. Moreover, all authors have contributed sufficiently in this study to be included as authors.
References
Abbott, S. B., and Pilowsky, P. M. (2009). Galanin microinjection into rostral ventrolateral medulla of the rat is hypotensive and attenuates sympathetic chemoreflex. Am. J. Physiol. Regul. Integr. Comp. Physiol. 296, R1019–R1026. doi: 10.1152/ajpregu.90885.2008
Adams, J. M., Madden, C. J., Sved, A. F., and Stocker, S. D. (2007). Increased dietary salt enhances sympathoexcitatory and sympathoinhibitory responses from the rostral ventrolateral medulla. Hypertension 50, 354–359. doi: 10.1161/HYPERTENSIONAHA.107.091843
Alheid, G. F., and McCrimmon, D. R. (2008). The chemical neuroanatomy of breathing. Respir. Physiol. Neurobiol. 164, 3–11. doi: 10.1016/j.resp.2008.07.014
Anastasi, A., Espamer, V., and Bucci, M. (1971). Isolation and structure of bombesin and alytesin, two analogous active peptides from the skin of the European amphibians Bombina and Alytes. Experientia 27, 166–167.
Bayorh, M. A., and Feuerstein, G. (1985). Bombesin and substance P modulate peripheral sympathetic and cardiovascular activity. Peptides 6(Suppl. 1), 115–120. doi: 10.1016/0196-9781(85)90019-1
Bergamaschi, C. T., Campos, R. R., and Lopes, O. U. (1999). Rostral ventrolateral medulla: a source of sympathetic activation in rats subjected to long-term treatment with L-NAME. Hypertension 34, 744–747. doi: 10.1161/01.HYP.34.4.744
Bergamaschi, C. T., Silva, N. F., Pires, J. G., Campos, R. R., and Neto, H. A. (2014). Participation of 5-HT and AT1 receptors within the rostral ventrolateral medulla in the maintenance of hypertension in the goldblatt 1 kidney-1 clip model. Int. J. Hypertens. 2014:723939. doi: 10.1155/2014/723939
Brown, M. R. (1983). Central nervous system sites of action of bombesin and somatostatin to influence plasma epinephrine levels. Brain Res. 276, 253–257. doi: 10.1016/0006-8993(83)90732-1
Brown, M. R., and Fisher, L. A. (1984). Brain peptide regulation of adrenal epinephrine secretion. Am. J. Physiol. 247, E41–E46.
Brown, M. R., and Guyenet, P. G. (1985). Electrophysiological study of cardiovascular neurons in the rostral ventrolateral medulla in rats. Brain Res. 549, 118–126. doi: 10.1161/01.res.56.3.359
Chahl, L. A., and Walker, S. B. (1981). Responses of the rat cardiovascular system to substance P, neurotensin and bombesin. Life Sci. 29, 2009–2015. doi: 10.1016/0024-3205(81)90611-1
Chan, R. K., Chan, Y. S., and Wong, T. M. (1991). Electrophysiological properties of neurons in the rostral ventrolateral medulla of normotensive and spontaneously hypertensive rats. Brain Res. 549, 118–126. doi: 10.1016/0006-8993(91)90607-W
Chung, K., Briner, R. P., Carlton, S. M., and Westlund, K. N. (1989). Immunohistochemical localization of seven different peptides in the human spinal cord. J. Comp. Neurol. 280, 158–170. doi: 10.1002/cne.902800111
Dampney, R. A., Coleman, M. J., Fontes, M. A., Hirooka, Y., Horiuchi, J., Li, Y. W., et al. (2002). Central mechanisms underlying short- and long-term regulation of the cardiovascular system. Clin. Exp. Pharmacol. Physiol. 29, 261–268. doi: 10.1046/j.1440-1681.2002.03640.x
Du, D., Chen, J., Liu, M., Zhu, M., Jing, H., Fang, J., et al. (2013). The effects of angiotensin II and angiotensin-(1-7) in the rostral ventrolateral medulla of rats on stress-induced hypertension. PLoS ONE 8:e70976. doi: 10.1371/journal.pone.0070976
Erspamer, V., Melchiorri, P., and Sopranzi, N. (1972). The action of bombesin on systemic arteria blood pressure of some experimental animals. Br. J. Pharinc. 45, 442–450. doi: 10.1111/j.1476-5381.1972.tb08100.x
Fink, G. D. (1997). Long-term sympatho-excitatory effect of angiotensin II: a mechanism of spontaneous and renovascular hypertension. Clin. Exp. Pharmacol. Physiol. 24, 91–95. doi: 10.1111/j.1440-1681.1997.tb01789.x
Glazkova, E. N., and Inyushkin, A. N. (2006). Respiratory reactions to microinjection of bombesin into the solitary tract nucleus and their mechanisms. Neurosci. Behav. Physiol. 36, 403–408. doi: 10.1007/s11055-006-0031-y
Gonzalez, N., Moody, T. W., Igarashi, H., Ito, T., and Jensen, R. T. (2008). Bombesin-related peptides and their receptors: recent advances in their role in physiology and disease states. Curr. Opin. Endocrinol. Diabetes Obes. 15, 58–64. doi: 10.1097/MED.0b013e3282f3709b
Guertzenstein, P. G. (1973). Blood pressure effects obtained by drugs applied to the ventral surface of the brain stem. J. Physiol. 229, 395–408. doi: 10.1113/jphysiol.1973.sp010145
Guertzenstein, P. G., and Silver, A. (1974). Fall in blood pressure produced from discrete regions of the ventral surface of the medulla by glycine and lesions. J. Physiol. 242, 489–503. doi: 10.1113/jphysiol.1974.sp010719
Guyenet, P. G. (2006). The sympathetic control of blood pressure. Nat. Rev. Neurosci. 7, 335–346. doi: 10.1038/nrn1902
Guyenet, P. G., Haselton, J. R., and Sun, M. K. (1989). Sympathoexcitatory neurons of the rostroventrolateral medulla and the origin of the sympathetic vasomotor tone. Prog. Brain Res. 81, 105–116. doi: 10.1016/S0079-6123(08)62002-6
Holtman, J. R. Jr., Jensen, R. T., Buller, A., Hamosh, P., Taveira Da Silva, A. M., and Gillis, R. A. (1983). Central respiratory stimulant effect of bombesin in the cat. Eur. J. Pharmacol. 90, 449–451. doi: 10.1016/0014-2999(83)90571-X
Ito, S., Hiratsuka, M., Komatsu, K., Tsukamoto, K., Kanmatsuse, K., and Sved, A. F. (2003). Ventrolateral medulla AT1 receptors support arterial pressure in dahl salt-sensitive rats. Hypertension 41, 744–75. doi: 10.1161/01.HYP.0000052944.54349.7B
Ito, S., Komatsu, K., Tsukamoto, K., and Sved, A. F. (2000). Excitatory amino acids in the rostral ventrolateral medulla support blood pressure in spontaneously hypertensive rats. Hypertension 35, 413–417. doi: 10.1161/01.HYP.35.1.413
Jensen, R. T., Battey, J. F., Spindel, E. R., and Benya, R. V. (2008). International union of pharmacology. LXVIII. mammalian bombesin receptors: nomenclature, distribution, pharmacology, signaling, and functions in normal and disease states. Pharmacol. Rev. 60, 1–42. doi: 10.1124/pr.107.07108
Kaczynska, K., and Szereda-Przestaszewska, M. (2011). Vasopressor and heart rate responses to systemic administration of bombesin in anesthetized rats. Pharmacol. Rep. 63, 448–454. doi: 10.1016/S1734-1140(11)70511-4
Li, P., Janczewski, W. A., Yackle, K., Kam, K., Pagliardini, S., Krasnow, M. A., et al. (2016). The peptidergic control circuit for sighing. Nature 530, 293–297. doi: 10.1038/nature16964
Loewy, A. D. (1981). Descending pathways to sympathetic and parasympathetic preganglionic neurons. J. Auton. Nerv. Syst. 3, 265–275. doi: 10.1016/0165-1838(81)90068-0
Lynn, R. B., Hyde, T. M., Cooperman, R. R., and Miselis, R. R. (1996). Distribution of bombesin-like immunoreactivity in the nucleus of the solitary tract and dorsal motor nucleus of the rat and human: colocalization with tyrosine hydroxylase. J. Comp. Neurol. 369, 552–570.
Matsuura, T., Kumagai, H., Kawai, A., Onimaru, H., Imai, M., Oshima, N., et al. (2002). Rostral ventrolateral medulla neurons of neonatal wistar-kyoto and spontaneously hypertensive rats. Hypertension 40, 560–565. doi: 10.1161/01.HYP.0000032043.64223.87
Millhorn, D. E., and Eldridge, F. L. (1986). Role of ventrolateral medulla in regulation of respiratory and cardiovascular systems. J. Appl. Physiol. 61, 1249–1263.
Miyawaki, T., Minson, J., Arnolda, L., Llewellyn-Smith, I., Chalmers, J., and Pilowsky, P. (1996). AMPA/kainate receptors mediate sympathetic chemoreceptor reflex in the rostral ventrolateral medulla. Brain Res. 726, 64–68.
Ohki-Hamazaki, H., Watase, K., Yamamoto, K., Ogura, H., Yamano, M., Yamada, K., et al. (1997). Mice lacking bombesin receptor subtype-3 develop metabolic defects and obesity. Nature 390, 165–169. doi: 10.1038/36568
Schreihofer, A. M., Stornetta, R. L., and Guyenet, P. G. (2000). Regulation of sympathetic tone and arterial pressure by rostral ventrolateral medulla after depletion of C1 cells in rat. J. Physiol. 529(Pt 1), 221–236. doi: 10.1111/j.1469-7793.2000.00221.x
Smith, J. C., Abdala, A. P., Koizumi, H., Rybak, I. A., and Paton, J. F. (2007). Spatial and functional architecture of the mammalian brain stem respiratory network: a hierarchy of three oscillatory mechanisms. J. Neurophysiol. 98, 3370–3387. doi: 10.1152/jn.00985.2007
Smith, J. K., and Barron, K. W. (1990). GABAergic responses in ventrolateral medulla in spontaneously hypertensive rats. Am. J. Physiol. 258, R450–R456.
Springell, D. A., Costin, N. S., Pilowsky, P. M., and Goodchild, A. K. (2005a). Hypotension and short-term anaesthesia induce ERK1/2 phosphorylation in autonomic nuclei of the brainstem. Eur. J. Neurosci. 22, 2257–2270. doi: 10.1111/j.1460-9568.2005.04413.x
Springell, D. A., Powers-Martin, K., Phillips, J. K., Pilowsky, P. M., and Goodchild, A. K. (2005b). Phosphorylated extracellular signal-regulated kinase 1/2 immunoreactivity identifies a novel subpopulation of sympathetic preganglionic neurons. Neuroscience 133, 583–590. doi: 10.1016/j.neuroscience.2005.02.036
Stocker, S. D., Meador, R., and Adams, J. M. (2007). Neurons of the rostral ventrolateral medulla contribute to obesity-induced hypertension in rats. Hypertension 49, 640–646. doi: 10.1161/01.HYP.0000254828.71253.dc
Suhaimi, F. W., Yusoff, N. H., Dewa, A., and Yusof, A. P. (2010). Role of excitatory amino acid input in rostral ventrolateral medulla neurons in rats with obesity-induced hypertension. Acta Neurol. Belg. 110, 57–64.
Sun, M. K., and Reis, D. J. (1995). NMDA receptor-mediated sympathetic chemoreflex excitation of RVL-spinal vasomotor neurones in rats. J. Physiol. 482(Pt 1), 53–68. doi: 10.1113/jphysiol.1995.sp020499
Toney, G. M., and Stocker, S. D. (2010). Hyperosmotic activation of CNS sympathetic drive: implications for cardiovascular disease. J. Physiol. 588, 3375–3384. doi: 10.1113/jphysiol.2010.191940
Wang, W. Z., Gao, L., Wang, H. J., Zucker, I. H., and Wang, W. (2009). Tonic glutamatergic input in the rostral ventrolateral medulla is increased in rats with chronic heart failure. Hypertension 53, 370–374. doi: 10.1161/HYPERTENSIONAHA.108.122598
Woodruff, G. N., Hall, M. D., Reynolds, T., and Pinnock, R. D. (1996). Bombesin receptors in the brain. Ann. N.Y. Acad. Sci. 780, 223–243. doi: 10.1111/j.1749-6632.1996.tb15126.x
Yang, T. L., Chai, C. Y., and Yen, C. T. (1995). Enhanced sympathetic reactivity to glutamate stimulation in medulla oblongata of spontaneously hypertensive rats. Am. J. Physiol. 268, H1499–H1509.
Keywords: rostral ventrolateral medulla, bombesin, BB1 receptors, BIM-23127, SHR
Citation: Pinto IS, Mourão AA, da Silva EF, Camargo AS, Marques SM, Gomes KP, Fajemiroye JO, da Silva Reis AA, Rebelo ACS, Ferreira-Neto ML, Rosa DA, Freiria-Oliveira AH, Castro CH, Colombari E, Colugnati DB and Pedrino GR (2016) Blockade of Rostral Ventrolateral Medulla (RVLM) Bombesin Receptor Type 1 Decreases Blood Pressure and Sympathetic Activity in Anesthetized Spontaneously Hypertensive Rats. Front. Physiol. 7:205. doi: 10.3389/fphys.2016.00205
Received: 11 February 2016; Accepted: 20 May 2016;
Published: 02 June 2016.
Edited by:
Valdir Andrade Braga, Federal University of Paraíba, BrazilReviewed by:
Simon McMullan, Macquarie University, AustraliaJosiane Campos Cruz, Federal University of Paraíba, Brazil
Copyright © 2016 Pinto, Mourão, da Silva, Camargo, Marques, Gomes, Fajemiroye, da Silva Reis, Rebelo, Ferreira-Neto, Rosa, Freiria-Oliveira, Castro, Colombari, Colugnati and Pedrino. This is an open-access article distributed under the terms of the Creative Commons Attribution License (CC BY). The use, distribution or reproduction in other forums is permitted, provided the original author(s) or licensor are credited and that the original publication in this journal is cited, in accordance with accepted academic practice. No use, distribution or reproduction is permitted which does not comply with these terms.
*Correspondence: Gustavo R. Pedrino, pedrino@pq.cnpq.br; gpedrino@gmail.com