- 1Research and Development Service, VA Portland Health Care System, Portland, OR, USA
- 2Mental Health and Clinical Neurosciences Division, VA Portland Health Care System, Portland, OR, USA
- 3Department of Psychiatry, Oregon Health & Science University, Portland, OR, USA
- 4Methamphetamine Abuse Research Center, Oregon Health & Science University, Portland, OR, USA
Objectives: It is hypothesized that immune factors influence addictive behaviors and contribute to relapse. The primary study objectives were to (1) compare neuropsychiatric symptoms across adults with active methamphetamine (MA) dependence, in early remission from MA dependence, and with no history of substance dependence, (2) determine whether active or recent MA dependence affects the expression of immune factors, and (3) evaluate the association between immune factor levels and neuropsychiatric symptoms.
Methods: A cross-sectional study was conducted using between group comparisons and regression analyses to investigate associations among variables. Eighty-four adults were recruited into control (CTL) (n = 31), MA-active (n = 17), or MA-remission (n = 36) groups. Participants completed self-report measures of anxiety, depression, and memory complaints and objective tests of attention and executive function. Blood samples were collected, and a panel of immune factors was measured using multiplex technology.
Results: Relative to CTLs, MA-dependent adults evidenced greater anxiety and depression during active use (p < 0.001) and remission (p < 0.007), and more attention, memory, and executive problems during remission (p < 0.01) but not active dependence. Regression analyses identified 10 immune factors (putatively associated with cytokine–cytokine receptor interactions) associated with anxiety, depression, and memory problems.
Conclusion: While psychiatric symptoms are present during active MA dependence and remission, at least some cognitive difficulties emerge only during remission. Altered expression of a network of immune factors contributes to neuropsychiatric symptom severity.
Introduction
Methamphetamine (MA) addiction is a pressing health concern and is a substance use disorder that faces many treatment challenges, including, but not limited to, the lack of effective treatments and the high prevalence of co-occurring mental and physical health conditions associated with the addiction. According to the 2012 National Survey on Drug Use and Health, approximately 1.2 million people reported using MA in the past year, and 440,000 reported using it in the past month (1). The costs associated with MA use are numerous, with contributions from crime/criminal justice, child endangerment, lost productivity, drug treatment, health care, MA production hazards, and premature death estimated at more than $23.4 billion in 2005 (2).
Exposure to MA impacts a range of both peripheral and central immune functions, such as alterations in B- and T-cell expression and response (e.g., antibody production, proliferation), natural killer cell activation, macrophage function (e.g., phagocytosis), glial cell-mediated cytokine expression, and immune cell trafficking – which likely contribute to the chronicity of the drug’s adverse and neurotoxic effects (3–23). Furthermore, MA use is associated with structural and functional changes to regions of the brain that regulate cognitive and psychiatric function and promote drug-seeking behaviors, making recovery from MA addiction very difficult (24–28). Approximately 40% of chronic MA users experience global neuropsychiatric difficulties (e.g., memory, attention, and executive functions) (26, 29, 30), and one-third to one-half or more of MA-dependent adults evidence psychiatric disorders (e.g., anxiety and depression) during remission (31–35). Neuropsychiatric impairments that persist following abstinence are associated with poorer treatment outcomes, including increased relapse rates, lower treatment retention rates, and reduced daily functioning (36–38).
Research is beginning to link immune factor signaling with neural and behavioral aspects of addiction, such as impaired cognitive function (10), drug seeking behaviors, and resilience to relapse (39–42). The immune system modulates central nervous system functions through a variety of signaling mechanisms, both in the absence and in the presence of immunological challenges (43–46). Likewise, numerous studies have demonstrated that peripheral alterations in the expression and function of immune factors (e.g., pro- and anti-inflammatory cytokines and chemokines) are evident in patients diagnosed with a range of neuropsychiatric and mental health disorders, including depression (47, 48), anxiety (49, 50), chronic fatigue syndrome (51), cancer-related fatigue and cognitive impairment (52), pain disorders (53–56), hepatitis C virus (HCV)-associated neuropsychiatric impairment (57), age-related cognitive decline and dementia (58–60), and, more recently, substance use disorders (10, 61). Collectively, these studies highlight the impact that immune activation and immune factor dysregulation (both peripherally and centrally) can have on central nervous system function.
In a translational study, we previously reported that mice exposed with repeated doses of MA evidence significantly altered expression of brain and plasma immune factors, both immediately following drug exposure and after a remission period, and that altered expression of several plasma immune factors is significantly correlated with reduced cognitive function in humans during remission from MA dependence (10). However, this study was limited to a panel of nine immune factors, and it did not include active MA users who may demonstrate different neuropsychiatric and immune consequences than those in remission from MA dependence. Although research investigating pathophysiological mechanisms and treatment approaches for MA addiction has been expanding, few studies have examined the relationship between immune factor expression and the development of commonly occurring neuropsychiatric symptoms in adults with MA use disorders. The primary objectives of the present study, therefore, were to (1) compare levels of anxiety, depression, and cognitive difficulties across three study groups – adults with active MA dependence, adults in early remission from MA dependence, and adult controls (CTLs) with no history of drug or alcohol dependence, (2) determine whether active or recent MA dependence and other covariates [age, gender, ethnicity, body mass index (BMI), nicotine use, or medical comorbidities] significantly influence the expression of a larger array of immune factors, and (3) evaluate the association between peripheral immune factor expression and anxiety, depression, and cognition in adults with and without MA dependence. The functional significance of the immune factors found to be predictive of neuropsychiatric impairments in our regression models was assessed using bioinformatics pathway analysis.
Materials and Methods
Research Participants
Eighty-four research participants gave informed consent, met all eligibility criteria, and were enrolled in the study; 29 others gave informed consent but were not enrolled in the study because they did not meet all eligibility criteria (i.e., they were screen fails). Participants were recruited from Portland area addiction treatment centers and the community through word of mouth and via study advertisements posted in clinics, websites, and newspapers. Participants were recruited into one of three groups: (1) CTL group (n = 31): adults with no lifetime history of dependence on any substance other than nicotine or caffeine; (2) MA-active (ACT) group (n = 17): adults actively using MA and currently meeting criteria for MA dependence; and (3) MA-remission (REM) group (n = 36): adults in remission from MA dependence ≥1 and ≤12 months.
General exclusion criteria included history of a major medical illness or current use of medications that are likely to be associated with serious neurological or immune dysfunction [e.g., stroke, traumatic brain injury, human immunodeficiency virus (HIV) infection, HCV infection, primary psychotic disorder, immunosuppressants, antivirals, anti-tumor necrosis factor (TNF)-alpha agents]. Additional exclusion criteria for the non-dependent CTL group included: (1) meets criteria for lifetime history of dependence on any substance (other than nicotine or caffeine dependence) based on diagnostic and statistical manual of mental disorders-fourth edition (DSM-IV) (62) and confirmed by the Mini-International Neuropsychiatric Interview (MINI) (63) and (2) on the day of the study visits, tests positive on a urine drug analysis for any drug of abuse. Additional inclusion criteria for the MA-ACT group included: (1) meets DSM-IV (62) criteria for dependence on MA, confirmed by the MINI (63), (2) average MA use was ≥2 days/week for ≥1 year (average years of dependence = 13.91, SD = 10.00), and (3) last use of MA was ≤2 weeks ago (average days since last use = 2.25 days, SD = 2.21 days, range = 1–8). Note that all individuals in the MA-ACT group completed a urine drug analysis, and most (58.8%) tested positive for MA. MA is generally detectable by urine drug analysis for approximately 3–5 days depending on a variety of individual factors such as the pH level of the urine. Thus, we included 7 participants (41.2% of the group) who tested negative for MA, reported having last used MA ≥ 3 days ago, and who otherwise met all criteria for the group and study. Although this is a limitation in that we could not verify MA use for those individuals, many individuals use MA only 2–3 days/week, so this procedure allowed us to include a sample that was generalizable to a broader range of use patterns. Additional inclusion criteria for the MA-REM group included: (1) meets DSM-IV (62) criteria for dependence on MA, confirmed by the MINI (63), (2) average MA use ≥2 days/week for ≥1 year (average years of dependence = 11.24, SD = 5.77), (3) last use of MA was ≥1 and ≤12 months ago (average days since last use = 108.82, SD = 73.82, range = 35–273), and (4) on the day of the study visit, does not test positive on a urine drug analysis for MA or any other drugs of abuse.
Ethical Approval
The protocol conformed to the ethical guidelines of the 1975 Declaration of Helsinki (6th revision, 2008) and was approved by the Institutional Review Boards at the Veterans Affairs Portland Health Care System and Oregon Health & Science University.
Procedures
Research participants were compensated with grocery store vouchers ($50) to complete the following study procedures: clinical interview, urine drug analysis, HCV and HIV antibody screening, blood sample collection for multiplex immune factor analysis as described in the sections below, questionnaires to assess severity of anxiety, depression and memory complaints, and objective cognitive measures to assess attention and executive function. Given the scope of the study, in order to facilitate rapid enrollment across the 14-month enrollment period, study visits were kept below 90 min; our questionnaire and cognitive assessment battery was limited to 15 min and, thus, did not include a comprehensive battery of objective cognitive tests. All procedures, including blood sample collection, were completed by certified phlebotomist/retired Licensed Practical Nurse (LPN) who was trained and supervised by a licensed psychologist and clinical neuropsychologist (Marilyn Huckans). To ensure accuracy, all measures were scored and then re-scored by separate study personnel, and all data were entered into a database initially and then double-checked by separate study personnel prior to analysis.
Questionnaires and Cognitive Assessment Measures
1. General anxiety disorder-7 scale (GAD-7) (64), a well-validated 7-item measure of anxiety.
2. Patient health questionaire-9 (PHQ-9) (65), a well-validated 9-item measure of depression severity.
3. Prospective and retrospective memory questionnaire (PRMQ) (66), a well-validated 16-item measure of self-reported memory complaints.
4. Digits forward, neuropsychological assessment battery (NAB) (67), well-validated and widely used objective performance measure of simple auditory attention span and tracking.
5. Letter fluency, Delis–Kaplan executive functioning system (D-KEFS) (68), a well-validated objective performance measure of verbal fluency and executive function.
Multiplex Immune Factor Assessments
Following all other study procedures, blood was drawn in the afternoon (mean time was 1:28 p.m., SD = 2:35 h) by one-time venipuncture into cell preparation tubes (BD Vacutainer Systems, Franklin Lakes, NJ, USA) containing 1 mL of 0.1M sodium citrate solution. The blood was then centrifuged at 1500 rpm for 20 min at room temperature (22–25°C). Plasma was separated, collected, and immediately aliquoted in polypropylene tubes (Phenix Research Products, Hayward, CA, USA) and frozen at −80°C until assayed. Samples were sent frozen in a single batch to Myriad Rules Based Medicine, Inc. (Austin, TX, USA) where they were thawed for assay without additional freeze–thaw cycles. Immune factors were measured using Myriad Rules Based Medicine, Inc.’s Human InflammationMAP v 1.0 panel, a biomarker panel designed to discern inflammatory patterns in biological samples including plasma. Myriad Rules Based Medicine, Inc. is a Clinical Laboratory Improvement Amendments (CLIA) certified laboratory. Assays conducted by this company utilizing this methodology have been published previously [e.g., Ref. (57, 69–71)]. This multiplex microbead assay is based on Luminex technology (72) and measures proteins in a similar manner to standard sandwich enzyme-linked immunosorbent assays (ELISAs), with comparable sensitivity and range. Multiplex assays have been compared to regular high sensitivity ELISAs in studies of Alzheimer’s disease, Parkinson’s disease, parasite infection, HIV, and others (73–76). Twenty-one factors from the original panel of 47 factors were undetectable (not measurable on the standard curve) in more than one-third of the total sample and were excluded from analyses [i.e., granulocyte-macrophage colony-stimulating factor, interferon-gamma, interleukin (IL)-1alpha, IL-1beta, IL-1 receptor antagonist, IL-2, IL-3, IL-4, IL-5, IL-6, IL-7, IL-10, IL-12p40, IL-12p70, IL-15, IL-17, macrophage inflammatory protein-1 alpha, matrix metalloproteinase-9, tumor necrosis factor (TNF)-alpha, and TNF-beta]. Table 2 summarizes the 26 remaining immune factors used for our analyses, including the factor’s abbreviation, unit of measurement, and the percentage within the total sample with detectable levels.
Bioinformatics Pathway Analysis
Functional analysis of the immune factor expression data was performed using the DAVID (Database for Annotation, Visualization and Integrated Discovery v6.7) Bioinformatics Resources,1 as previously described, with minor modifications (71). Briefly, the 10 immune factors identified as significant predictors of neuropsychiatric symptoms (Table 3) were assessed for significant enrichment of biological processes using the terms of the fifth level of gene ontology (GO). The name of each factor was converted to an analyzable identifier via Universal Protein Resource (UniProt) and then entered in the DAVID functional annotation tool. Pathway mapping analysis was carried out using the Kyoto Encyclopedia of Genes and Genomes (KEGG) module within DAVID.
Statistical Analysis
Data analyses were conducted using Stata v.12 (StataCorp LP, College Station, TX, USA), with specific methodologies described in the footnotes for Tables 1–3. Significant p values were ≤0.050.
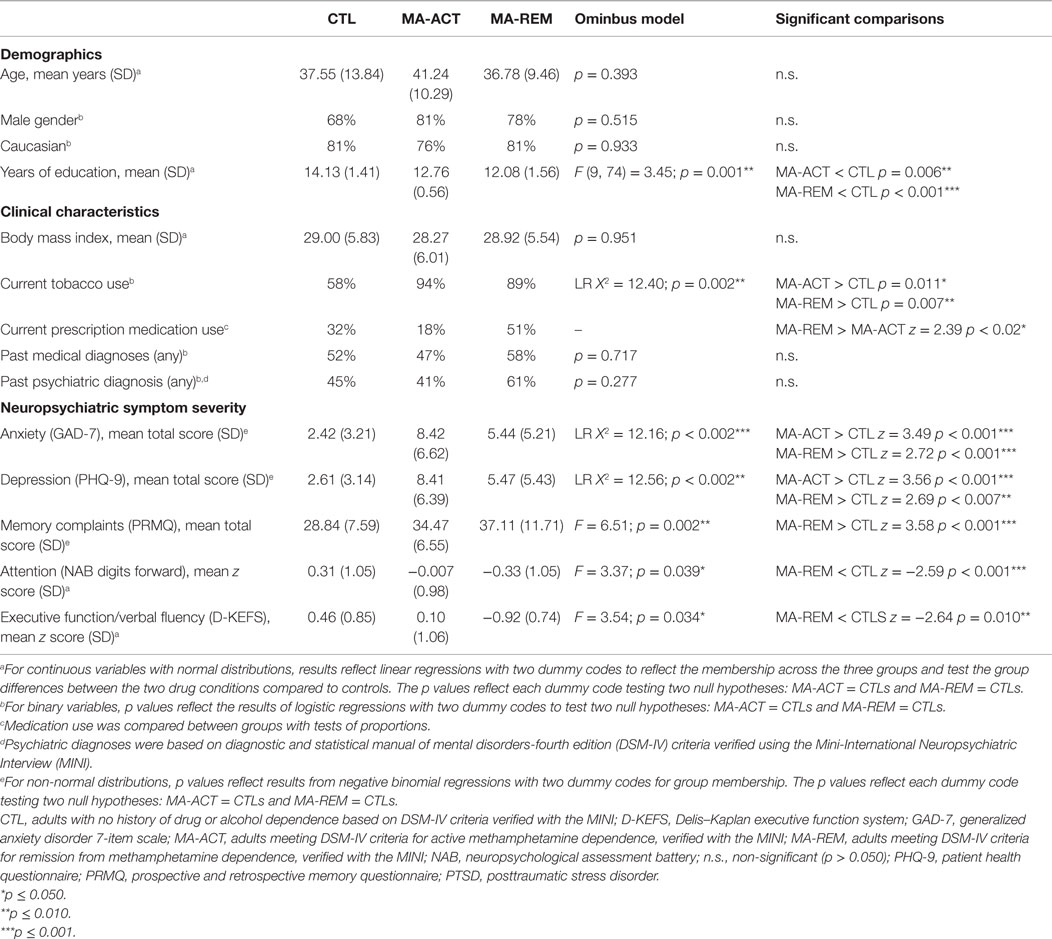
Table 1. Between group comparisons of demographics, clinical characteristics, anxiety, depression, and cognition in adults with and without methamphetamine dependence.
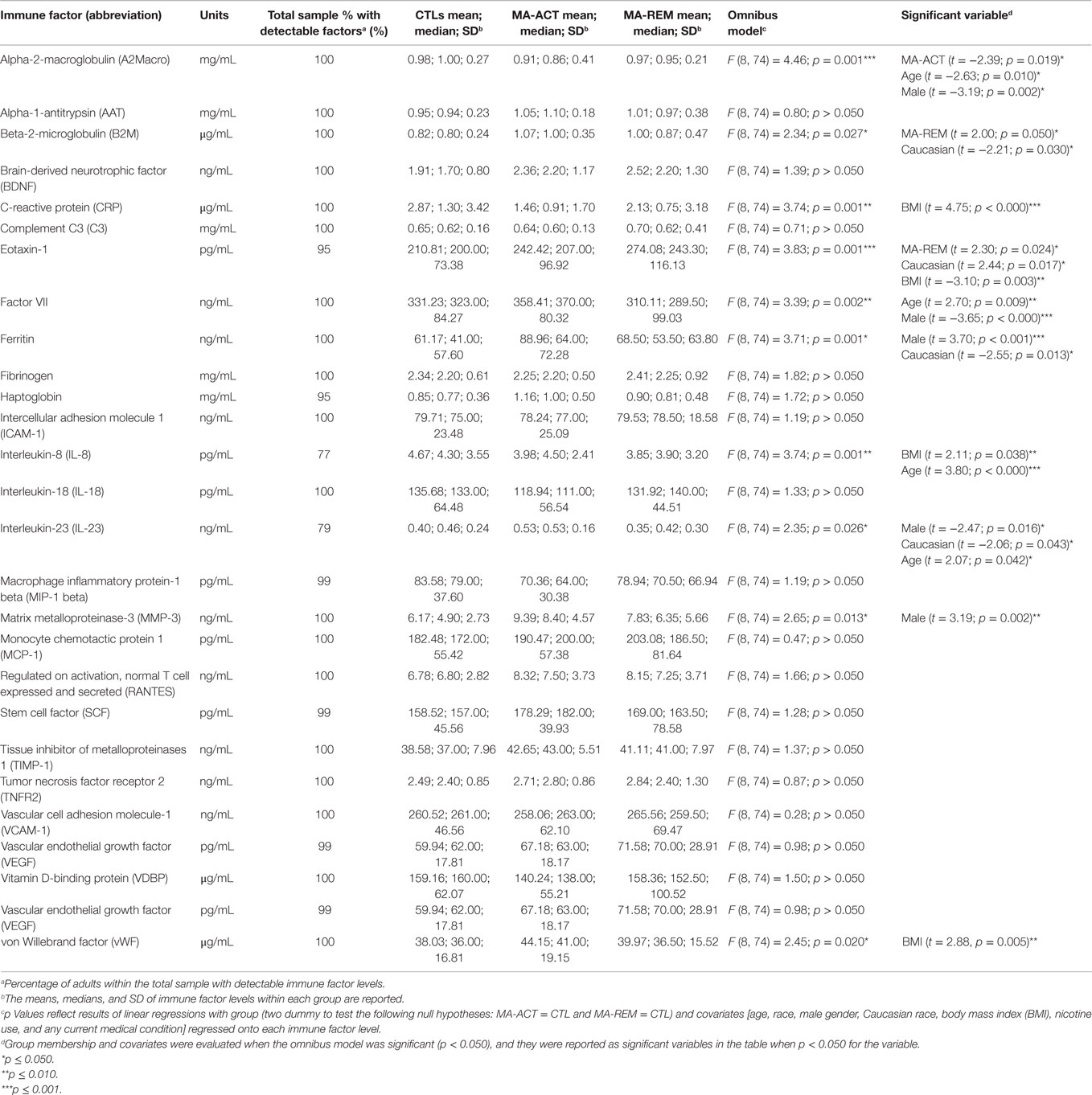
Table 2. Covariate analysis of plasma immune factor levels in adults with and without methamphetamine dependence.
Results
Demographics and Clinical Data
Table 1 summarizes demographic data, clinical characteristics, MA use characteristics, and neuropsychiatric outcomes by study group. Groups differed significantly by education (on average CTLs had two more years of education than the MA groups), tobacco use (a lower proportion of CTLs used tobacco), and current medications (the MA-ACT group was significantly less likely to be taking any medications than the MA-REM group, but there were no significant differences between MA-ACT and CLTs or MA-REM and CTLs). There were no significant group differences in terms of age, gender, race, BMI, or rates of medical or psychiatric diagnoses; adults with severe or unstable medical or psychiatric disorders were excluded from the study. MA-ACT and MA-REM groups reported significantly higher levels of anxiety and depression than CTLs. Relative to CTLs, the MA-REM group, but not the MA-ACT group, reported more memory problems and performed worse on tests of attention and executive function.
Covariate Analyses of Plasma Immune Markers
Table 2 summarizes the results of regression analyses to determine if study group or covariates (age, race, gender, BMI, tobacco use, and any medical condition) significantly predicted each of the peripheral immune factor levels in the total sample. Each of the regression models had a single Type I error rate for the predictors (p < 0.05) that was determined by the omnibus test of the model fit, limiting the risk of Type I error due to multiple comparisons. Being in the MA-ACT group versus CTL group was significantly associated with lower levels of A2Macro, and being in the MA-REM group versus the CTL group was significantly associated with higher levels of B2M and eotaxin-1. Older age was significantly associated with lower levels of A2Macro and higher levels of factor VII, IL-23, and IL-8. Male gender was significantly associated with lower levels of A2Macro, factor VII, and IL-23, and higher levels of ferritin and MMP-3. Caucasian race was significantly associated with lower levels of B2M, ferritin, and IL-23, and higher levels of eotaxin-1. BMI was significantly associated with higher levels of CRP, IL-8, and vWF, and lower levels of eotaxin-1. Neither using tobacco nor having a medical condition was significantly associated with any immune factor level.
Immune Factor Predictors of Anxiety, Depression, and Cognition
As summarized in Table 3, across all participants, regressions (controlling for study group and age, race, gender, BMI, tobacco use, and any medical condition as covariates) revealed a direct relationship between plasma immune factor levels and anxiety, depression, and memory complaints; the overall regression models were not significant for attention or executive function and so were not included in the table. Each of the regression models had a single Type I error rate for the predictors that was determined by the omnibus test of the model fit, limiting the risk of Type I error due to multiple comparisons. Figure 1 summarizes results from the regression models and shows the immune factors that were found to be significantly associated with each neuropsychiatric outcome. A total of 10 variables were found to be significantly associated with neuropsychiatric outcomes (i.e., CRP, eotaxin-1, fibrinogen, haptoglobin, ICAM-1, IL-8, IL-23, MMP-3, SCF, and VEGF).
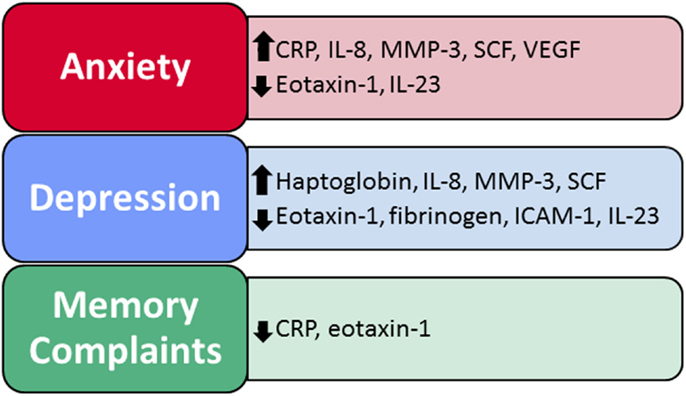
Figure 1. Immune factors predict self-reported anxiety, depression, and memory problems. Two to eight immune factors were significant predictors of neuropsychiatric function within each of the regression models, for a total of 10 significant immune factors across all models.
Bioinformatic Functional Analysis
Using the Kyoto Encyclopedia of Genes and Genomes (KEGG) pathways analysis feature of the database for annotation, visualization, and integrated discovery (DAVID), we analyzed the 10 immune factors found to be significantly associated with neuropsychiatric symptoms in Table 3 for significant interaction within biologically relevant pathways. In doing so, the cytokine–cytokine receptor interaction pathway was determined to be most relevant (p = 0.0002), incorporating five (i.e., eotaxin-1, IL-8, IL-23, SCF, and VEGF) of the previously identified 10 immune factors (Figure S1 in Supplementary Material; Table 4). Four other pathways – bladder cancer (p = 0.056), pathways in cancer (p = 0.07), NOD-like receptor signaling pathway (p = 0.082), and complement and coagulation (p = 0.091) – were also generated by the database; however, they did not reach statistical significance.
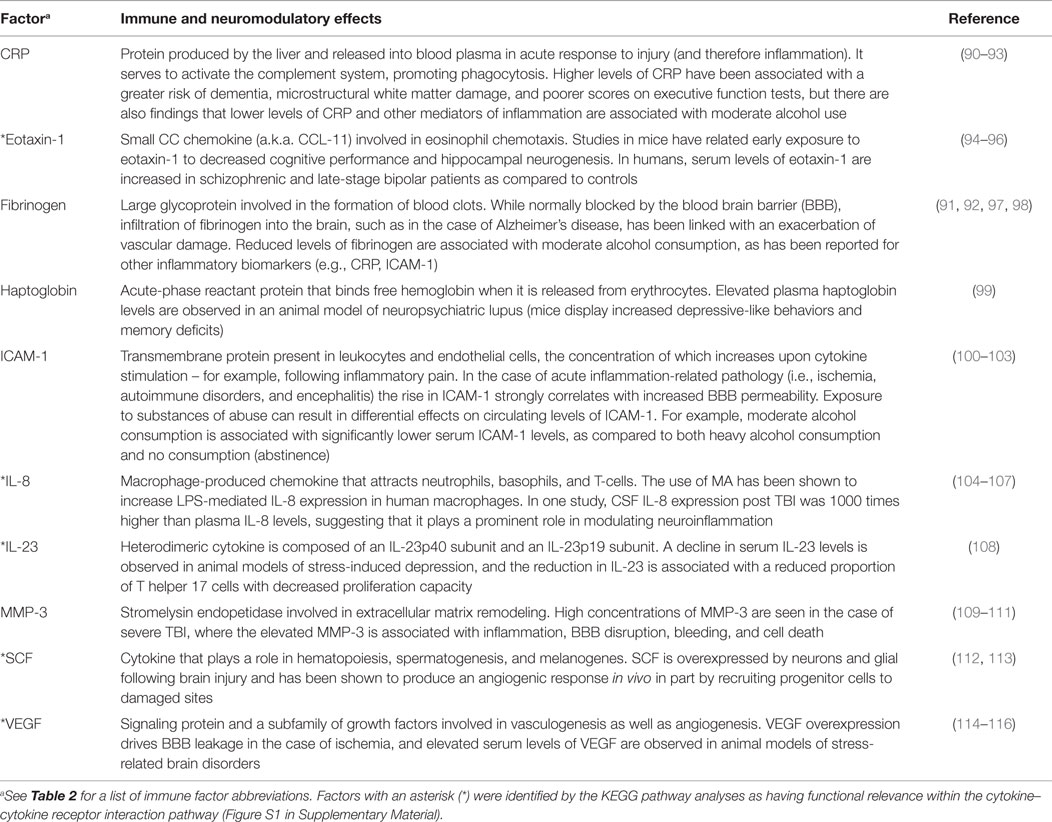
Table 4. Immune and neuromodulatory effects of inflammatory factors associated with neuropsychiatric symptomatology.
Discussion
This cross-sectional analysis of adults with and without a history of MA dependence indicates that, compared with non-dependent CTLs, both active MA users and those in recent remission from MA dependence report significantly (p < 0.050) higher levels of anxiety and depression (Table 1). Notably, while adults in remission from MA dependence reported significantly more memory problems and performed worse on attention and executive function tasks than CTLs, active MA users did not evidence these cognitive deficits relative to CTLs (Table 1). While numerous studies have documented cognitive impairments in adults in remission from MA dependence (10, 26, 29, 30), relatively few have examined cognitive function in non-intoxicated adults during active dependence (i.e., non-remission). Our findings suggest that pathological processes that occur following abstinence from MA dependence, rather than during active use per se, result in at least some cognitive problems that initially appear and persist during remission.
Results additionally suggest that current and recent MA dependence, age, gender, race, and BMI can significantly impact peripheral immune signaling, as they were each significant predictors of some but not all plasma immune factor levels in our sample (Table 2). This is consistent with the previous literature showing altered peripheral immune signaling in substance users (10, 61), older adults (59), women (77), black Americans (78), and adults with higher BMI (79).
Moreover, results show that differences in the expression of a network of peripheral immune proteins significantly impact neuropsychiatric function in adults both with and without present or past MA dependence. In our regression models (which controlled for covariates that might impact neuropsychiatric function or immune factor expression – MA dependence, age, gender, race, BMI, nicotine use, and medical comorbidities), a subset of immune factors significantly predicted self-reported anxiety, depression, and memory problems (Table 3). Specifically, two to eight immune factors were significant predictors of neuropsychiatric function within each of the regression models, for a total of 10 significant immune factors across all models – CRP, eotaxin-1, fibrinogen, haptoglobin, ICAM-1, IL-8, IL-23, MMP-3, SCF, and VEGF (Figure 1).
A major goal of this study was to identify novel biomarkers that might be relevant to the treatment of neuropsychiatric symptoms, including the context of MA use disorder, and these 10 significant predictors are thus potentially worthy of further investigation through additional studies (e.g., as treatment targets). It is notable that each of these factors have both immunoregulatory and neuromodulatory functions (see Table 4 for a summary of their actions), with the potential to both enhance inflammatory responses and adversely impact neuronal functions (e.g., induce microstructural white matter damage, impair phagocytosis, alter cell trafficking, and compromise BBB integrity) when dysregulated. Thus, our results suggest that efforts to develop and investigate novel immunotherapies as treatments for neuropsychiatric symptoms (particularly in the context of substance use disorders) are warranted.
In animal models of MA addiction and other substance use disorders, a variety of addictive behaviors (e.g., withdrawal, craving, conditioned place preference, locomotor sensitization, self-administration, sedation, and motor impairments) have been significantly altered through interventions that directly impact immune signaling (80, 81). Consequently, immunotherapies that are designed to simultaneously “normalize” immunoregulation and neuromodulation may be particularly effective in treating neuropsychiatric symptoms that can contribute to and exacerbate substance abuse. Clinical trials have already demonstrated the antidepressant benefits of several immunotherapies, such as etanercept (TNF-alpha antagonist used to treat a range of autoimmune conditions, including psoriasis and arthritis), infliximab (monoclonal antibody against TNF-alpha also used for the treatment of autoimmune diseases), and celecoxib [cyclooxygenase (COX)-2 inhibitor used to treat pain and arthritis] (82, 83). Additional immunotherapies for the treatment of neuropsychiatric and substance use disorders are currently under investigation by our lab and others. For example, our recent preclinical efficacy data demonstrate that RTL551, a partial major histocompatibility construct (pMHC) and novel neuroimmune modulator, effectively reduces MA-induced memory deficits in mice (84) and regulates the expression of pro-inflammatory cytokines (84), indicating that the immune system modulates the pharmacodynamics and behavioral consequences of drug actions. Similarly, ibudilast (AV-411 or MN-166), a less specific peripheral and central anti-inflammatory agent, reduces MA self-administration and stress-induced relapse in rodents (85–87) and is currently being evaluated as a potential neuroimmune therapy for adults with MA, opioid, or alcohol use disorders.2
The present study includes several limitations. A cross-sectional study design does not allow for definitive conclusions on causality, and group sample sizes may have limited our statistical power. Regression analyses are considered exploratory in nature and should be interpreted cautiously prior to replication. Our sample was largely middle aged, male, and Caucasian, with all adults residing in one Northwest metropolitan area (the greater Portland area), so results may not be generalizable to more diverse populations. Our study was limited to a panel of peripheral plasma immune proteins; additional biomarkers and functional pathways of interest may be revealed by future human studies, including gene arrays, or through animal studies that are better able to evaluate brain tissue for central immune factor expression. Our study included only a brief battery of questionnaires and cognitive assessment measures. Although we did not find a significant relationship between our immune factors and our two objective cognitive measures (i.e., digit span and fluency), we did find a significant relationship between our immune factors and self-reported memory complaints. Future studies could, therefore, include a more comprehensive battery of objective cognitive tests to determine whether immune factors are more predictive of subjective cognitive complaints versus objective cognitive performance. Lastly, it should be noted that this study was exploratory in nature (i.e., included a large array of immune factors without a priori hypotheses about each) and should be replicated before results are deemed definitive.
Despite limitations, our results demonstrate that, relative to non-dependent CTL participants, MA-dependent adults evidence anxiety and depressive symptoms both during active use and remission, and they experience difficulties with aspects of cognition (attention, memory, and executive function) that initially develop and then persist only during remission – an observation with noteworthy treatment implications (88). To the best of our knowledge, this is one of first studies to investigate the role of inflammatory immune factors on neuropsychiatric symptoms in adults with active MA dependence, as compared to both adults in remission from MA and to non-dependent CTLs. Results suggest that altered expression of a network of plasma immune factors contributes to neuropsychiatric symptom severity (i.e., anxiety, depression, and memory problems) in adults with and without MA addiction. Moreover, our study identified 10 immune factors (CRP, eotaxin-1, fibrinogen, haptoglobin, ICAM-1, IL-8, IL-23, MMP-3, SCF, and VEGF) that may be particularly relevant to neuropsychiatric symptoms, given their putative roles in cytokine–cytokine receptor interactions (Figure S1 in Supplementary Material) and in the regulation of both immune and neuronal functions (Table 4). Thus, although the pathophysiological mechanisms contributing to MA addiction are not fully understood, immune dysregulation and immune factors, such as cytokines, chemokines, and cellular adhesion molecules, likely play a critical role in perpetuating MA-induced neuronal injury and neuropsychiatric impairments (10, 89).
Author Contributions
The work presented here was carried out in collaboration among all authors. JL and MH designed the research plan and methods. MH supervised the research participant procedures. JL directed the laboratory work. BF, MH, and JL analyzed the data, interpreted the results and wrote the paper. AC and JL conducted the DAVID analyses. MA and AC performed literature searches, contributed to writing the paper, and discussed analyses, interpretation, and presentation. All authors have contributed to, seen and approved the manuscript.
Conflict of Interest Statement
The authors have the following conflicts: Oregon Health & Science University, the VA Portland Health Care System, Dr. Marilyn Huckans and Dr. Jennifer M. Loftis have a significant financial interest in Artielle Immunotherapeutics, Inc., a company that may have a commercial interest in the results of this research and technology. These potential individual and institutional conflicts of interest have been reviewed and managed by Oregon Health & Science University.
Acknowledgments
The authors would like to thank the study participants and staff at each of the recruitment sites and Patricia Newman for study coordination. The authors also acknowledge Drs. John Marshall and Bryan Yamamoto for valuable consultations. The contents do not represent the views of the U.S. Department of Veterans Affairs or the United States Government.
Funding
This material is the result of work supported with resources and the use of facilities at the Veterans Affairs Portland Health Care System, Oregon Health & Science University, and the Methamphetamine Abuse Research Center (NIDA P50DA018165), Portland, OR, USA. This work was supported by National Institutes of Health grants RC1DA028537 and R41DA029345 to MH and JL and by the U.S. Department of Veterans Affairs Biomedical Laboratory Research and Development Merit Review grant (#I01 BX002061) to JL.
Supplementary Material
The Supplementary Material for this article can be found online at http://journal.frontiersin.org/article/10.3389/fpsyt.2015.00178
Footnotes
References
1. Substance Abuse and Mental Health Services Administration. Results from the 2012 National Survey on Drug Use and Health: Summary of National Findings, NSDUH Series H-46, HHS Publication No. (SMA) 13-4795. Rockville, MD: Substance Abuse and Mental Health Services Administration (2013).
2. Nicosia N, Pacula RL, Kilmer B, Lundberg R, Chiesa J. The Economic Cost of Methamphetamine Use in the United States, 2005, MG-829-MPF/NIDA (2009). Available from: http://www.rand.org/pubs/monographs/MG829
3. Assis MA, Hansen C, Lux-Lantos V, Cancela LM. Sensitization to amphetamine occurs simultaneously at immune level and in met-enkephalin of the nucleus accumbens and spleen: an involved NMDA glutamatergic mechanism. Brain Behav Immun (2009) 23(4):464–73. doi:10.1016/j.bbi.2009.01.003
4. Assis MA, Valdomero A, Garcia-Keller C, Sotomayor C, Cancela LM. Decrease of lymphoproliferative response by amphetamine is mediated by dopamine from the nucleus accumbens: influence on splenic met-enkephalin levels. Brain Behav Immun (2011) 25(4):647–57. doi:10.1016/j.bbi.2011.01.001
5. Buchanan JB, Sparkman NL, Johnson RW. Methamphetamine sensitization attenuates the febrile and neuroinflammatory response to a subsequent peripheral immune stimulus. Brain Behav Immun (2010) 24(3):502–11. doi:10.1016/j.bbi.2009.12.008
6. Carrico AW, Johnson MO, Morin SF, Remien RH, Riley ED, Hecht FM, et al. Stimulant use is associated with immune activation and depleted tryptophan among HIV-positive persons on anti-retroviral therapy. Brain Behav Immun (2008) 22(8):1257–62. doi:10.1016/j.bbi.2008.07.010
7. In SW, Son EW, Rhee DK, Pyo S. Modulation of murine macrophage function by methamphetamine. J Toxicol Environ Health A (2004) 67(23–24):1923–37. doi:10.1080/15287390490514589
8. Liang H, Wang X, Chen H, Song L, Ye L, Wang SH, et al. Methamphetamine enhances HIV infection of macrophages. Am J Pathol (2008) 172(6):1617–24. doi:10.2353/ajpath.2008.070971
9. Llorente-Garcia E, Abreu-Gonzalez P, Gonzalez-Hernandez MC. Hematological, immunological and neurochemical effects of chronic amphetamine treatment in male rats. J Physiol Biochem (2009) 65(1):61–9. doi:10.1007/BF03165970
10. Loftis JM, Choi D, Hoffman W, Huckans MS. Methamphetamine causes persistent immune dysregulation: a cross-species, translational report. Neurotox Res (2011) 20(1):59–68. doi:10.1007/s12640-010-9223-x
11. Mahajan SD, Hu Z, Reynolds JL, Aalinkeel R, Schwartz SA, Nair MP. Methamphetamine modulates gene expression patterns in monocyte derived mature dendritic cells: implications for HIV-1 pathogenesis. Mol Diagn Ther (2006) 10(4):257–69. doi:10.1007/BF03256465
12. Marcondes MC, Flynn C, Watry DD, Zandonatti M, Fox HS. Methamphetamine increases brain viral load and activates natural killer cells in simian immunodeficiency virus-infected monkeys. Am J Pathol (2010) 177(1):355–61. doi:10.2353/ajpath.2010.090953
13. Martinez LR, Mihu MR, Gacser A, Santambrogio L, Nosanchuk JD. Methamphetamine enhances histoplasmosis by immunosuppression of the host. J Infect Dis (2009) 200(1):131–41. doi:10.1086/599328
14. Nair MP, Saiyed ZM, Nair N, Gandhi NH, Rodriguez JW, Boukli N, et al. Methamphetamine enhances HIV-1 infectivity in monocyte derived dendritic cells. J Neuroimmune Pharmacol (2009) 4(1):129–39. doi:10.1007/s11481-008-9128-0
15. Potula R, Hawkins BJ, Cenna JM, Fan S, Dykstra H, Ramirez SH, et al. Methamphetamine causes mitrochondrial oxidative damage in human T lymphocytes leading to functional impairment. J Immunol (2010) 185(5):2867–76. doi:10.4049/jimmunol.0903691
16. Reynolds JL, Mahajan SD, Aalinkeel R, Nair B, Sykes DE, Schwartz SA. Proteomic analyses of the effects of drugs of abuse on monocyte-derived mature dendritic cells. Immunol Invest (2009) 38(6):526–50. doi:10.1080/08820130902874110
17. Saito M, Yamaguchi T, Kawata T, Ito H, Kanai T, Terada M, et al. Effects of methamphetamine on cortisone concentration, NK cell activity and mitogen response of T-lymphocytes in female cynomolgus monkeys. Exp Anim (2006) 55(5):477–81. doi:10.1538/expanim.55.477
18. Shah A, Silverstein PS, Singh DP, Kumar A. Involvement of metabotropic glutamate receptor 5, AKT/PI3K signaling and NF-kappaB pathway in methamphetamine-mediated increase in IL-6 and IL-8 expression in astrocytes. J Neuroinflammation (2012) 9:52. doi:10.1186/1742-2094-9-52
19. Talloczy Z, Martinez J, Joset D, Ray Y, Gacser A, Toussi S, et al. Methamphetamine inhibits antigen processing, presentation, and phagocytosis. PLoS Pathog (2008) 4(2):e28. doi:10.1371/journal.ppat.0040028
20. Wang X, Wang Y, Ye L, Li J, Zhou Y, Sakarcan S, et al. Modulation of intracellular restriction factors contributes to methamphetamine-mediated enhancement of acquired immune deficiency syndrome virus infection of macrophages. Curr HIV Res (2012) 10(5):407–14. doi:10.2174/157016212802138797
21. Wey SP, Wu HY, Chang FC, Jan TR. Methamphetamine and diazepam suppress antigen-specific cytokine expression and antibody production in ovalbumin-sensitized BALB/c mice. Toxicol Lett (2008) 181(3):157–62. doi:10.1016/j.toxlet.2008.07.015
22. Ye L, Peng JS, Wang X, Wang YJ, Luo GX, Ho WZ. Methamphetamine enhances hepatitis C virus replication in human hepatocytes. J Viral Hepat (2008) 15(4):261–70. doi:10.1111/j.1365-2893.2007.00940.x
23. Yu Q, Zhang D, Walston M, Zhang J, Liu Y, Watson RR. Chronic methamphetamine exposure alters immune function in normal and retrovirus-infected mice. Int Immunopharmacol (2002) 2(7):951–62. doi:10.1016/S1567-5769(02)00047-4
24. Hoffman WF, Schwartz DL, Huckans MS, McFarland BH, Meiri G, Stevens AA, et al. Cortical activation during delay discounting in abstinent methamphetamine dependent individuals. Psychopharmacology (2008) 201(2):183–93. doi:10.1007/s00213-008-1261-1
25. Schwartz DL, Mitchell AD, Lahna DL, Luber HS, Huckans MS, Mitchell SH, et al. Global and local morphometric differences in recently abstinent methamphetamine-dependent individuals. Neuroimage (2010) 50(4):1392–401. doi:10.1016/j.neuroimage.2010.01.056
26. Scott JC, Woods SP, Matt GE, Meyer RA, Heaton RK, Atkinson JH, et al. Neurocognitive effects of methamphetamine: a critical review and meta-analysis. Neuropsychol Rev (2007) 17(3):275–97. doi:10.1007/s11065-007-9031-0
27. Volkow ND, Chang L, Wang GJ, Fowler JS, Leonido-Yee M, Franceschi D, et al. Association of dopamine transporter reduction with psychomotor impairment in methamphetamine abusers. Am J Psychiatry (2001) 158(3):377–82. doi:10.1176/appi.ajp.158.3.377
28. Wang GJ, Volkow ND, Chang L, Miller E, Sedler M, Hitzemann R, et al. Partial recovery of brain metabolism in methamphetamine abusers after protracted abstinence. Am J Psychiatry (2004) 161(2):242–8. doi:10.1176/appi.ajp.161.2.242
29. Fernandez-Serrano MJ, Perez-Garcia M, Verdejo-Garcia A. What are the specific vs. generalized effects of drugs of abuse on neuropsychological performance? Neurosci Biobehav Rev (2011) 35(3):377–406. doi:10.1016/j.neubiorev.2010.04.008
30. Hoffman WF, Moore M, Templin R, McFarland B, Hitzemann RJ, Mitchell SH. Neuropsychological function and delay discounting in methamphetamine-dependent individuals. Psychopharmacology (2006) 188(2):162–70. doi:10.1007/s00213-006-0494-0
31. Darke S, Kaye S, McKetin R, Duflou J. Major physical and psychological harms of methamphetamine use. Drug Alcohol Rev (2008) 27(3):253–62. doi:10.1080/09595230801923702
32. Glasner-Edwards S, Mooney LJ, Marinelli-Casey P, Hillhouse M, Ang A, Rawson RA, et al. Psychopathology in methamphetamine-dependent adults 3 years after treatment. Drug Alcohol Rev (2010) 29(1):12–20. doi:10.1111/j.1465-3362.2009.00081.x
33. London ED, Simon SL, Berman SM, Mandelkern MA, Lichtman AM, Bramen J, et al. Mood disturbances and regional cerebral metabolic abnormalities in recently abstinent methamphetamine abusers. Arch Gen Psychiatry (2004) 61(1):73–84. doi:10.1001/archpsyc.61.1.73
34. Shoptaw S, Peck J, Reback CJ, Rotheram-Fuller E. Psychiatric and substance dependence comorbidities, sexually transmitted diseases, and risk behaviors among methamphetamine-dependent gay and bisexual men seeking outpatient drug abuse treatment. J Psychoactive Drugs (2003) 35(Suppl 1):161–8. doi:10.1080/02791072.2003.10400511
35. Zweben JE, Cohen JB, Christian D, Galloway GP, Salinardi M, Parent D, et al. Psychiatric symptoms in methamphetamine users. Am J Addict (2004) 13(2):181–90. doi:10.1080/10550490490436055
36. Aharonovich E, Nunes E, Hasin D. Cognitive impairment, retention and abstinence among cocaine abusers in cognitive-behavioral treatment. Drug Alcohol Depend (2003) 71(2):207–11. doi:10.1016/S0376-8716(03)00092-9
37. Fals-Stewart W. Neurocognitive defects and their impact on substance abuse treatment. J Addict Offender Couns (1993) 13:46–57. doi:10.1002/j.2161-1874.1993.tb00083.x
38. Sadek JR, Vigil O, Grant I, Heaton RK. The impact of neuropsychological functioning and depressed mood on functional complaints in HIV-1 infection and methamphetamine dependence. J Clin Exp Neuropsychol (2007) 29(3):266–76. doi:10.1080/13803390600659384
39. Blednov YA, Mayfield RD, Belknap J, Harris RA. Behavioral actions of alcohol: phenotypic relations from multivariate analysis of mutant mouse data. Genes Brain Behav (2012) 11(4):424–35. doi:10.1111/j.1601-183X.2012.00780.x
40. Blednov YA, Ponomarev I, Geil C, Bergeson S, Koob GF, Harris RA. Neuroimmune regulation of alcohol consumption: behavioral validation of genes obtained from genomic studies. Addict Biol (2012) 17(1):108–20. doi:10.1111/j.1369-1600.2010.00284.x
41. Schwarz JM, Hutchinson MR, Bilbo SD. Early-life experience decreases drug-induced reinstatement of morphine CPP in adulthood via microglial-specific epigenetic programming of anti-inflammatory IL-10 expression. J Neurosci (2011) 31(49):17835–47. doi:10.1523/JNEUROSCI.3297-11.2011
42. Zhang XQ, Cui Y, Cui Y, Chen Y, Na XD, Chen FY, et al. Activation of p38 signaling in the microglia in the nucleus accumbens contributes to the acquisition and maintenance of morphine-induced conditioned place preference. Brain Behav Immun (2012) 26(2):318–25. doi:10.1016/j.bbi.2011.09.017
43. Capuron L, Miller AH. Immune system to brain signaling: neuropsychopharmacological implications. Pharmacol Ther (2011) 130(2):226–38. doi:10.1016/j.pharmthera.2011.01.014
44. Dantzer R, Kelley KW. Twenty years of research on cytokine-induced sickness behavior. Brain Behav Immun (2007) 21(2):153–60. doi:10.1016/j.bbi.2006.09.006
45. Loftis JM, Huckans M, Morasco BJ. Neuroimmune mechanisms of cytokine-induced depression: current theories and novel treatment strategies. Neurobiol Dis (2010) 37(3):519–33. doi:10.1016/j.nbd.2009.11.015
46. McAfoose J, Baune BT. Evidence for a cytokine model of cognitive function. Neurosci Biobehav Rev (2009) 33(3):355–66. doi:10.1016/j.neubiorev.2008.10.005
47. Leonard B, Maes M. Mechanistic explanations how cell-mediated immune activation, inflammation and oxidative and nitrosative stress pathways and their sequels and concomitants play a role in the pathophysiology of unipolar depression. Neurosci Biobehav Rev (2012) 36(2):764–85. doi:10.1016/j.neubiorev.2011.12.005
48. Loftis JM, Huckans M, Ruimy S, Hinrichs DJ, Hauser P. Depressive symptoms in patients with chronic hepatitis C are correlated with elevated plasma levels of interleukin-1beta and tumor necrosis factor-alpha. Neurosci Lett (2008) 430(3):264–8. doi:10.1016/j.neulet.2007.11.001
49. Hoge EA, Brandstetter K, Moshier S, Pollack MH, Wong KK, Simon NM. Broad spectrum of cytokine abnormalities in panic disorder and posttraumatic stress disorder. Depress Anxiety (2009) 26(5):447–55. doi:10.1002/da.20564
50. Hou R, Baldwin DS. A neuroimmunological perspective on anxiety disorders. Hum Psychopharmacol (2012) 27(1):6–14. doi:10.1002/hup.1259
51. Arnett SV, Clark IA. Inflammatory fatigue and sickness behaviour – lessons for the diagnosis and management of chronic fatigue syndrome. J Affect Disord (2012) 141(2–3):130–42. doi:10.1016/j.jad.2012.04.004
52. Meyers CA, Albitar M, Estey E. Cognitive impairment, fatigue, and cytokine levels in patients with acute myelogenous leukemia or myelodysplastic syndrome. Cancer (2005) 104(4):788–93. doi:10.1002/cncr.21234
53. Alexander GM, Peterlin BL, Perreault MJ, Grothusen JR, Schwartzman RJ. Changes in plasma cytokines and their soluble receptors in complex regional pain syndrome. J Pain (2012) 13(1):10–20. doi:10.1016/j.jpain.2011.10.003
54. de Oliveira CM, Sakata RK, Issy AM, Gerola LR, Salomao R. Cytokines and pain. Rev Bras Anestesiol (2011) 61(2):255–9, 60–5, 137–42. doi:10.1016/S0034-7094(11)70029-0
55. Slade GD, Conrad MS, Diatchenko L, Rashid NU, Zhong S, Smith S, et al. Cytokine biomarkers and chronic pain: association of genes, transcription, and circulating proteins with temporomandibular disorders and widespread palpation tenderness. Pain (2011) 152(12):2802–12. doi:10.1016/j.pain.2011.09.005
56. Starkweather AR, Lyon DE, Schubert CM. Pain and inflammation in women with early-stage breast cancer prior to induction of chemotherapy. Biol Res Nurs (2013) 15(2):234–41. doi:10.1177/1099800411425857
57. Huckans M, Fuller BE, Olavarria H, Sasaki AW, Chang M, Flora KD, et al. Multi-analyte profile analysis of plasma immune proteins: altered expression of peripheral immune factors is associated with neuropsychiatric symptom severity in adults with and without chronic hepatitis C virus infection. Brain Behav (2014) 4(2):123–42. doi:10.1002/brb3.200
58. Britschgi M, Wyss-Coray T. Blood protein signature for the early diagnosis of Alzheimer disease. Arch Neurol (2009) 66(2):161–5. doi:10.1001/archneurol.2008.530
59. Corona AW, Fenn AM, Godbout JP. Cognitive and behavioral consequences of impaired immunoregulation in aging. J Neuroimmune Pharmacol (2012) 7(1):7–23. doi:10.1007/s11481-011-9313-4
60. Yaffe K, Kanaya A, Lindquist K, Simonsick EM, Harris T, Shorr RI, et al. The metabolic syndrome, inflammation, and risk of cognitive decline. JAMA (2004) 292(18):2237–42. doi:10.1001/jama.292.18.2237
61. Loftis JM, Huckans M. Substance use disorders: psychoneuroimmunological mechanisms and new targets for therapy. Pharmacol Ther (2013) 139(2):289–300. doi:10.1016/j.pharmthera.2013.04.011
62. American Psychiatric Association. Diagnostic and Statistical Manual for Mental Disorders. 4th Edition, Text Revision ed. Washington, DC: American Psychiatric Association (2000).
63. Sheehan DV, Lecrubier Y, Sheehan KH, Amorim P, Janavs J, Weiller E, et al. The Mini-International Neuropsychiatric Interview (M.I.N.I.): the development and validation of a structured diagnostic psychiatric interview for DSM-IV and ICD-10. J Clin Psychiatry (1998) 59(Suppl 20):22–33; quiz4–57.
64. Spitzer RL, Kroenke K, Williams JB, Lowe B. A brief measure for assessing generalized anxiety disorder: the GAD-7. Arch Intern Med (2006) 166(10):1092–7. doi:10.1001/archinte.166.10.1092
65. Kroenke K, Spitzer RL, Williams JB. The PHQ-9: validity of a brief depression severity measure. J Gen Intern Med (2001) 16(9):606–13. doi:10.1046/j.1525-1497.2001.016009606.x
66. Smith G, Della Sala S, Logie RH, Maylor EA. Prospective and retrospective memory in normal ageing and dementia: a questionnaire study. Memory (2000) 8(5):311–21. doi:10.1080/09658210050117735
67. Stern RA, White T. Neuropsychological Assessment Battery: Administration, Scoring, and Interpretation Manual. Lutz, FL: Psychological Assessment Resources (2003).
68. Delis D, Kaplan E, Kramer J. Delis-Kaplan Executive Functioning System. New York, NY: Harcourt Assessment Company (2001).
69. Freeman WM, Salzberg AC, Gonzales SW, Grant KA, Vrana KE. Classification of alcohol abuse by plasma protein biomarkers. Biol Psychiatry (2010) 68(3):219–22. doi:10.1016/j.biopsych.2010.01.028
70. Schrijvers EM, Koudstaal PJ, Hofman A, Breteler MM. Plasma clusterin and the risk of Alzheimer disease. JAMA (2011) 305(13):1322–6. doi:10.1001/jama.2011.381
71. Wilhelm CJ, Choi D, Huckans M, Manthe L, Loftis JM. Adipocytokine signaling is altered in flinders sensitive line rats, and adiponectin correlates in humans with some symptoms of depression. Pharmacol Biochem Behav (2013) 103(3):643–51. doi:10.1016/j.pbb.2012.11.001
72. Vignali DA. Multiplexed particle-based flow cytometric assays. J Immunol Methods (2000) 243(1–2):243–55. doi:10.1016/S0022-1759(00)00238-6
73. Camargo JF, Quinones MP, Mummidi S, Srinivas S, Gaitan AA, Begum K, et al. CCR5 expression levels influence NFAT translocation, IL-2 production, and subsequent signaling events during T lymphocyte activation. J Immunol (2009) 182(1):171–82. doi:10.4049/jimmunol.182.1.171
74. Codices V, Martins C, Novo C, de Sousa B, Lopes A, Borrego M, et al. Dynamics of cytokines and immunoglobulins serum profiles in primary and secondary Cryptosporidium parvum infection: usefulness of Luminex(R) xMAP technology. Exp Parasitol (2013) 133(1):106–13. doi:10.1016/j.exppara.2012.11.003
75. Hu WT, Chen-Plotkin A, Arnold SE, Grossman M, Clark CM, Shaw LM, et al. Biomarker discovery for Alzheimer’s disease, frontotemporal lobar degeneration, and Parkinson’s disease. Acta Neuropathol (2010) 120(3):385–99. doi:10.1007/s00401-010-0723-9
76. O’Bryant SE, Waring SC, Hobson V, Hall JR, Moore CB, Bottiglieri T, et al. Decreased C-reactive protein levels in Alzheimer disease. J Geriatr Psychiatry Neurol (2010) 23(1):49–53. doi:10.1177/0891988709351832
77. Jansen R, Batista S, Brooks AI, Tischfield JA, Willemsen G, van Grootheest G, et al. Sex differences in the human peripheral blood transcriptome. BMC Genomics (2014) 15:33. doi:10.1186/1471-2164-15-33
78. Longo DM, Louie B, Putta S, Evensen E, Ptacek J, Cordeiro J, et al. Single-cell network profiling of peripheral blood mononuclear cells from healthy donors reveals age- and race-associated differences in immune signaling pathway activation. J Immunol (2012) 188(4):1717–25. doi:10.4049/jimmunol.1102514
79. Esser N, Legrand-Poels S, Piette J, Scheen AJ, Paquot N. Inflammation as a link between obesity, metabolic syndrome and type 2 diabetes. Diabetes Res Clin Pract (2014) 105(2):141–50. doi:10.1016/j.diabres.2014.04.006
80. Coller JK, Hutchinson MR. Implications of central immune signaling caused by drugs of abuse: mechanisms, mediators and new therapeutic approaches for prediction and treatment of drug dependence. Pharmacol Ther (2012) 134(2):219–45. doi:10.1016/j.pharmthera.2012.01.008
81. Loftis JM, Janowsky A. Neuroimmune basis of methamphetamine toxicity. Int Rev Neurobiol (2014) 118:165–97. doi:10.1016/B978-0-12-801284-0.00007-5
82. Muller N, Schwarz MJ, Dehning S, Douhe A, Cerovecki A, Goldstein-Muller B, et al. The cyclooxygenase-2 inhibitor celecoxib has therapeutic effects in major depression: results of a double-blind, randomized, placebo controlled, add-on pilot study to reboxetine. Mol Psychiatry (2006) 11(7):680–4. doi:10.1038/sj.mp.4001805
83. Tyring S, Gottlieb A, Papp K, Gordon K, Leonardi C, Wang A, et al. Etanercept and clinical outcomes, fatigue, and depression in psoriasis: double-blind placebo-controlled randomised phase III trial. Lancet (2006) 367(9504):29–35. doi:10.1016/S0140-6736(05)67763-X
84. Loftis JM, Wilhelm CJ, Vandenbark AA, Huckans M. Partial MHC/neuroantigen peptide constructs: a potential neuroimmune-based treatment for methamphetamine addiction. PLoS One (2013) 8(2):e56306. doi:10.1371/journal.pone.0056306
85. Beardsley PM, Shelton KL, Hendrick E, Johnson KW. The glial cell modulator and phosphodiesterase inhibitor, AV411 (ibudilast), attenuates prime- and stress-induced methamphetamine relapse. Eur J Pharmacol (2010) 637(1–3):102–8. doi:10.1016/j.ejphar.2010.04.010
86. Snider SE, Hendrick ES, Beardsley PM. Glial cell modulators attenuate methamphetamine self-administration in the rat. Eur J Pharmacol (2013) 701(1–3):124–30. doi:10.1016/j.ejphar.2013.01.016
87. Snider SE, Vunck SA, van den Oord EJ, Adkins DE, McClay JL, Beardsley PM. The glial cell modulators, ibudilast and its amino analog, AV1013, attenuate methamphetamine locomotor activity and its sensitization in mice. Eur J Pharmacol (2012) 679(1–3):75–80. doi:10.1016/j.ejphar.2012.01.013
88. Loftis JM, Huckans M. Cognitive enhancement in combination with “brain repair” may be optimal for the treatment of stimulant addiction. Addiction (2011) 106(5):1021–2. doi:10.1111/j.1360-0443.2010.03354.x
89. Narita M, Suzuki M, Kuzumaki N, Miyatake M, Suzuki T. Implication of activated astrocytes in the development of drug dependence: differences between methamphetamine and morphine. Ann N Y Acad Sci (2008) 1141:96–104. doi:10.1196/annals.1441.032
90. Badía E, Sacanella E, Fernández-Solá J, Nicolás JM, Antunez E, Rotilio D, et al. Decreased tumor necrosis factor-induced adhesion of human monocytes to endothelial cells after moderate alcohol consumption. Am J Clin Nutr (2004) 80(1):225–30.
91. Messaoudi I, Pasala S, Grant K. Could moderate alcohol intake be recommended to improve vaccine responses? Expert Rev Vaccines (2014) 13(7):817–9. doi:10.1586/14760584.2014.924405
92. Wang JJ, Tung TH, Yin WH, Huang CM, Jen HL, Wei J, et al. Effects of moderate alcohol consumption on inflammatory biomarkers. Acta Cardiol (2008) 63(1):65–72. doi:10.2143/AC.63.1.2025334
93. Wersching H, Duning T, Lohmann H, Mohammadi S, Stehling C, Fobker M, et al. Serum C-reactive protein is linked to cerebral microstructural integrity and cognitive function. Neurology (2010) 74(13):1022–9. doi:10.1212/WNL.0b013e3181d7b45b
94. Villeda SA, Luo J, Mosher KI, Zou B, Britschgi M, Bieri G, et al. The ageing systemic milieu negatively regulates neurogenesis and cognitive function. Nature (2011) 477(7362):90–4. doi:10.1038/nature10357
95. Panizzutti B, Gubert C, Schuh AL, Ferrari P, Bristot G, Fries GR, et al. Increased serum levels of eotaxin/CCL11 in late-stage patients with bipolar disorder: an accelerated aging biomarker? J Affect Disord (2015) 182:64–9. doi:10.1016/j.jad.2014.12.010
96. Teixeira AL, Reis HJ, Nicolato R, Brito-Melo G, Correa H, Teixeira MM, et al. Increased serum levels of CCL11/eotaxin in schizophrenia. Prog Neuropsychopharmacol Biol Psychiatry (2008) 32(3):710–4. doi:10.1016/j.pnpbp.2007.11.019
97. Muradashvili N, Lominadze D. Role of fibrinogen in cerebrovascular dysfunction after traumatic brain injury. Brain Inj (2013) 27(13–14):1508–15. doi:10.3109/02699052.2013.823562
98. Ryu JK, McLarnon JG. A leaky blood-brain barrier, fibrinogen infiltration and microglial reactivity in inflamed Alzheimer’s disease brain. J Cell Mol Med (2009) 13(9A):2911–25. doi:10.1111/j.1582-4934.2008.00434.x
99. Li Y, Eskelund AR, Zhou H, Budac DP, Sanchez C, Gulinello M. Behavioral deficits are accompanied by immunological and neurochemical changes in a mouse model for neuropsychiatric lupus (NP-SLE). Int J Mol Sci (2015) 16(7):15150–71. doi:10.3390/ijms160715150
100. Hess DC, Bhutwala T, Sheppard JC, Zhao W, Smith J. ICAM-1 expression on human brain microvascular endothelial cells. Neurosci Lett (1994) 168(1–2):201–4. doi:10.1016/0304-3940(94)90450-2
101. Huber JD, Campos CR, Mark KS, Davis TP. Alterations in blood-brain barrier ICAM-1 expression and brain microglial activation after lambda-carrageenan-induced inflammatory pain. Am J Physiol Heart Circ Physiol (2006) 290(2):H732–40. doi:10.1152/ajpheart.00747.2005
102. Sacanella E, Badia E, Nicolas JM, Fernández-Solá J, Antúnez E, Urbano-Márquez A, et al. Differential effects of moderate or heavy alcohol consumption on circulating adhesion molecule levels. Thromb Haemost (2002) 88(1):52–5.
103. Steiner O, Coisne C, Cecchelli R, Boscacci R, Deutsch U, Engelhardt B, et al. Differential roles for endothelial ICAM-1, ICAM-2, and VCAM-1 in shear-resistant T cell arrest, polarization, and directed crawling on blood-brain barrier endothelium. J Immunol (2010) 185(8):4846–55. doi:10.4049/jimmunol.0903732
104. Ellman LM, Deicken RF, Vinogradov S, Kremen WS, Poole JH, Kern DM, et al. Structural brain alterations in schizophrenia following fetal exposure to the inflammatory cytokine interleukin-8. Schizophr Res (2010) 121(1–3):46–54. doi:10.1016/j.schres.2010.05.014
105. Kushi H, Saito T, Makino K, Hayashi N. IL-8 is a key mediator of neuroinflammation in severe traumatic brain injuries. Acta Neurochir Suppl (2003) 86:347–50.
106. Liu X, Silverstein PS, Singh V, Shah A, Qureshi N, Kumar A. Methamphetamine increases LPS-mediated expression of IL-8, TNF-alpha and IL-1beta in human macrophages through common signaling pathways. PLoS One (2012) 7(3):e33822. doi:10.1371/journal.pone.0033822
107. Yamada K, Nabeshima T. Pro- and anti-addictive neurotrophic factors and cytokines in psychostimulant addiction: mini review. Ann N Y Acad Sci (2004) 1025:198–204. doi:10.1196/annals.1316.025
108. Hong M, Zheng J, Ding ZY, Chen JH, Yu L, Niu Y, et al. Imbalance between Th17 and Treg cells may play an important role in the development of chronic unpredictable mild stress-induced depression in mice. Neuroimmunomodulation (2013) 20(1):39–50. doi:10.1159/000343100
109. Falo MC, Fillmore HL, Reeves TM, Phillips LL. Matrix metalloproteinase-3 expression profile differentiates adaptive and maladaptive synaptic plasticity induced by traumatic brain injury. J Neurosci Res (2006) 84(4):768–81. doi:10.1002/jnr.20986
110. Grossetete M, Phelps J, Arko L, Yonas H, Rosenberg GA. Elevation of matrix metalloproteinases 3 and 9 in cerebrospinal fluid and blood in patients with severe traumatic brain injury. Neurosurgery (2009) 65(4):702–8. doi:10.1227/01.NEU.0000351768.11363.48
111. Mendes O, Kim HT, Stoica G. Expression of MMP2, MMP9 and MMP3 in breast cancer brain metastasis in a rat model. Clin Exp Metastasis (2005) 22(3):237–46. doi:10.1007/s10585-005-8115-6
112. Sun L, Hui AM, Su Q, Vortmeyer A, Kotliarov Y, Pastorino S, et al. Neuronal and glioma-derived stem cell factor induces angiogenesis within the brain. Cancer Cell (2006) 9(4):287–300. doi:10.1016/j.ccr.2006.03.003
113. Sun L, Lee J, Fine HA. Neuronally expressed stem cell factor induces neural stem cell migration to areas of brain injury. J Clin Invest (2004) 113(9):1364–74. doi:10.1172/JCI200420001
114. Argaw AT, Asp L, Zhang J, Navrazhina K, Pham T, Mariani JN, et al. Astrocyte-derived VEGF-A drives blood-brain barrier disruption in CNS inflammatory disease. J Clin Invest (2012) 122(7):2454–68. doi:10.1172/JCI60842
115. Stewart AM, Roy S, Wong K, Gaikwad S, Chung KM, Kalueff AV. Cytokine and endocrine parameters in mouse chronic social defeat: implications for translational ‘cross-domain’ modeling of stress-related brain disorders. Behav Brain Res (2015) 276:84–91. doi:10.1016/j.bbr.2014.08.037
Keywords: anxiety, biological markers, depression, cognition, cytokines, inflammation, substance abuse
Citation: Huckans M, Fuller BE, Chalker ALN, Adams M and Loftis JM (2015) Plasma Inflammatory Factors Are Associated with Anxiety, Depression, and Cognitive Problems in Adults with and without Methamphetamine Dependence: An Exploratory Protein Array Study. Front. Psychiatry 6:178. doi: 10.3389/fpsyt.2015.00178
Received: 04 September 2015; Accepted: 04 December 2015;
Published: 18 December 2015
Edited by:
Susana Roque, University of Minho, PortugalCopyright: © 2015 Huckans, Fuller, Chalker, Adams and Loftis. This is an open-access article distributed under the terms of the Creative Commons Attribution License (CC BY). The use, distribution or reproduction in other forums is permitted, provided the original author(s) or licensor are credited and that the original publication in this journal is cited, in accordance with accepted academic practice. No use, distribution or reproduction is permitted which does not comply with these terms.
*Correspondence: Marilyn Huckans, marilyn.huckans@va.gov;
Jennifer M. Loftis, loftisj@ohsu.edu, jennifer.loftis2@va.gov