Palynology and the Ecology of the New Zealand Conifers
- 1Manaaki Whenua-Landcare Research, Lincoln, New Zealand
- 2School of Environment, University of Auckland, Auckland, New Zealand
The New Zealand conifers (20 species of trees and shrubs in the Araucariaceae, Podocarpaceae, and Cupressaceae) are often regarded as ancient Gondwanan elements, but mostly originated much later. Often thought of as tall trees of humid, warm forests, they are present throughout in alpine shrublands, tree lines, bogs, swamps, and in dry, frost-prone regions. The tall conifers rarely form purely coniferous forest and mostly occur as an emergent stratum above evergreen angiosperm trees. During Maori settlement in the thirteenth century, fire-sensitive trees succumbed rapidly, most of the drier forests being lost. As these were also the more conifer-rich forests, ecological research has been skewed toward conifer dynamics of forests wetter and cooler than the pre-human norm. Conifers are well represented in the pollen record and we here we review their late Quaternary history in the light of what is known about their current ecology with the intention of countering this bias. During glacial episodes, all trees were scarce south of c. 40° S, and extensive conifer-dominant forest was confined to the northern third of the North Island. Drought- and cold-resistant Halocarpus bidwillii and Phyllocladus alpinus formed widespread scrub in the south. During the deglacial, beginning 18,000 years ago, tall conifers underwent explosive spread to dominate the forest biomass throughout. Conifer dominance lessened in favor of angiosperms in the wetter western lowland forests over the Holocene but the dryland eastern forests persisted largely unchanged until settlement. Mid to late Holocene climate change favored the more rapidly growing Nothofagaceae which replaced the previous conifer-angiosperm low forest or shrubland in tree line ecotones and montane areas. The key to this dynamic conifer history appears to be their bimodal ability to withstand stress, and dominate on poor soils and in cool, dry regions but, in wetter, warmer locations, to slowly grow thorough competing broadleaves to occupy an exposed, emergent stratum where their inherent stress resistance ensures little effective angiosperm competition.
Introduction
In 1935, Lucy Cranwell—a young New Zealand researcher attending the VI International Botanical Congress in Amsterdam—was invited to work with Lennart von Post on the pollen analysis of peat sequences collected from southern New Zealand by the Swedish glaciologist Carl Caldenius (Cameron, 2000). Their resulting paper on the postglacial history of the far south of the South Island (Cranwell and von Post, 1936) was the first such effort for Australasia and provided a compelling narrative of vegetation and climate change that was adopted by ecologists and Quaternary researchers alike. Several decades later, palynologist Bill Harris—who had worked for Lucy Cranwell—asked whether “… the two techniques, that of the ecologist, and that of the palynologist can be mutually helpful…” (Harris, 1963), and this question remains relevant both in New Zealand and elsewhere (Rull, 2010; Reitalu et al., 2014). Palaeoecology and neoecology often appear to be proceeding on quite different tracks, publishing in different journals and addressing quite separate themes. The purpose of this paper is to address Bill Harris's question with particular emphasis on the history of the New Zealand conifers, and to assess progress in integrating the two disciplines over the 80 years since Lucy Cranwell and Lennart von Post's pioneering publication.
New Zealand conifers offer an excellent opportunity to integrate the rapidly developing understanding of their ecology and biogeography with insights derived from nearly a century of palynological research. The 20 conifer species in New Zealand (Table 1; Figure 1) are represented by three distinct families: Araucariaceae (1 genus, 1 species), Cupressaceae (1 genus, 2 species) and Podocarpaceae (including the synapomorphic Phyllocladaceae; 8 genera, 16 species). Six of the most abundant of the tree conifer species are easily identified by their pollen (Table 1); many of the conifer species are emergent; and all are wind-pollinated. These traits have resulted in a detailed representation of conifer taxa in the terrestrial and marine pollen records from across the entire geological sequence in New Zealand, allowing the long-term dynamics of conifers to be confidently reconstructed. In contrast, many of the angiosperm trees and shrubs in New Zealand are insect pollinated, have poorly dispersed pollen that is mostly identifiable to genus level, and tend to be under-represented in the pollen records relative to their local abundance (Macphail and McQueen, 1983). The New Zealand conifer pollen records therefore provide an ideal setting to expand understanding of conifer history, biogeography, and ecology.
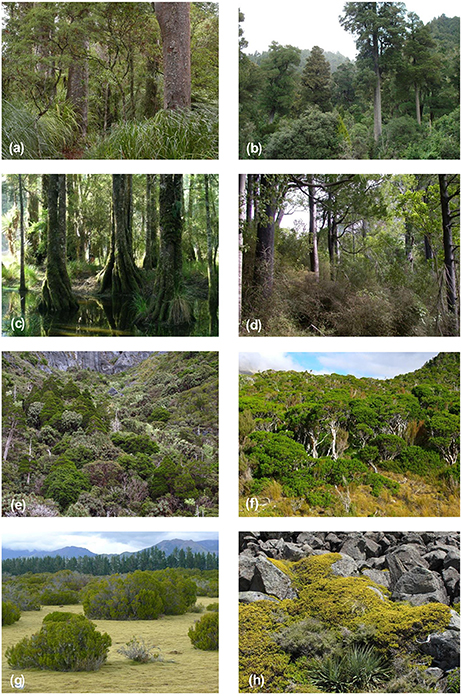
Figure 1. Examples of New Zealand conifer growth forms and habitats. (a) Agathis australis-dominated forest, Puketi Forest, northern North Island (b) alluvial conifer forest on pumice soils with Prumnopitys taxifolia, P. ferruginea, and Dacrycarpus dacrydioides, Ngaputahi, central North Island (c) Dacrycarpus dacrydioides swamp forest, Arohaki Lagoon, central North Island (d) dryland Prumnopitys taxifolia forest with dense divaricating shrub understorey, Isolation Creek, north-eastern South Island (e) even-aged, landslide-induced Libocedrus bidwillii stand, Ghost Valley, north-west South Island (f) Halocarpus biformis shrubland at treeline, Hunts Creek, Westland (g) shrubby Halocarpus bidwillii on frosty, leached terraces, south-west South Island (h) prostrate Podocarpus nivalis, Kakanui Mountains, south-eastern South Island. All images by the authors except (h) from John Barkla sourced under CC-BY-NC from iNaturalist.
Ecological studies of New Zealand conifers have focused on their forest dynamics at small spatial and limited time scales (sub-millennial) although progress has also been made in understanding their physiology, and soil preferences and climate drivers at a national level. In contrast, pollen analytical studies typically address time scales ranging from hundreds to millions of years, are often carried out by researchers with a geological or geographic background, and the major preoccupation has been interpreting pollen sequences in terms of climate or landscape change. This mismatch means integration of ecological and palynological data has been somewhat neglected.
Conifers are abundant in New Zealand forests and shrublands (Ogden and Stewart, 1995). They are found from tree line to the lowlands, from the driest to the wettest regions and from the northern tip of the North Island to Stewart Island in the far south, absent only from some of the offshore islands of the archipelago (Table 1). They include the tallest tree (50 m) and also sprawling, prostrate shrubs. Many of the conifers are large, emergent trees and often dominate forest biomass. Several (Agathis australis, Prumnopitys taxifolia, Podocarpus totara, Dacrycarpus dacrydioides, and Dacrydium cupressinum) yield valuable timber, which underpinned the New Zealand economy in the first few decades of European settlement and continued to be exploited until the closing decades of the twentieth century. Understandably, these ubiquitous, dominant and valuable trees have been a focus of biogeographic and ecological research in New Zealand, and debates over their origin, ecological role and, in particular, regeneration dynamics, have continued unabated over the last 120 years.
Leonard Cockayne was the first New Zealand ecologist and, in The Vegetation of New Zealand (Cockayne, 1928), formulated ideas about the ecology of the conifers, many of which remain current. However, more controversially, drawing on both ecological and macrofossil evidence he argued that conifer and angiosperm species were locked in a longstanding evolutionary conflict. The historical tendency, as he saw it, was for conifer retreat in the face of angiosperm competition and, although disturbance and poor soils could give them a temporary advantage from time to time, his opinion was that eventually they would become relic: “…a remnant merely of ancient conifer forests which have been in the process of gradual extinction by certain broad-leaved dicotyledonous trees—a process of extreme slowness” (Cockayne, 1928, p. 21). Cockayne's ideas were championed by Robbins (1962) who, after a descriptive survey of the conifer-angiosperm forests of the North Island, likewise claimed the angiosperm forest “represents a broadleaf forest climax which is surely replacing a more ancient podocarp forest climax, remnants of which still remain mingled with the broadleaf forest” (p 34). This view has persisted that the conifers and other older broadleaved genera represent an unchanging rainforest element from a Gondwana predating the 80–85 Ma separation of the ancestral Zealandia continental fragment (Kirkpatrick and DellaSala, 2011). The popular conservation literature often refers to the conifer-rich lowland forests of New Zealand as “dinosaur forest” (http://www.aucklandbotanicgardens.co.nz/whats-on/events/dinosaurs-in-the-gardens/). A recent publication on the fossil history of the Southern Hemisphere rainforests referred to their characteristic taxa as “southern wet forest survivors” (Kooyman et al., 2014), thus emphasizing their antiquity and embattled persistence. It has been claimed that the conifers—because of their antiquity and slow adaptation to Pleistocene climates—are photosynthetically adapted to function at higher temperatures than are optimal for present day New Zealand (Hawkins and Sweet, 1989). It is not unreasonable to see this presumption of “primitiveness” as implicitly guiding the tenor of much ecological discussion about southern conifers, which becomes focussed on their survival in an “advanced” angiosperm dominated world.
Here we provide an overview of the reaction of New Zealand conifers to climate and landscape transformation during and after the Last Glacial Maximum (LGM), including the impact of recent human arrival and the introduction of fire. We then use this background to explore to what extent the long-term perspective provided by pollen analytical data can shed light on their current ecology and if, the concept of southern conifers as besieged relics is either valid or useful.
New Zealand Vegetation Change Over the Last 30,000 Years
General locations are given in Figure 2 and Figures 3, 4 and 5–9 provide representative pollen diagrams illustrating the changes discussed. Table 2 summarizes the typical climatic regimes of important conifer-dominant vegetation types in relation to their current and past distributions.
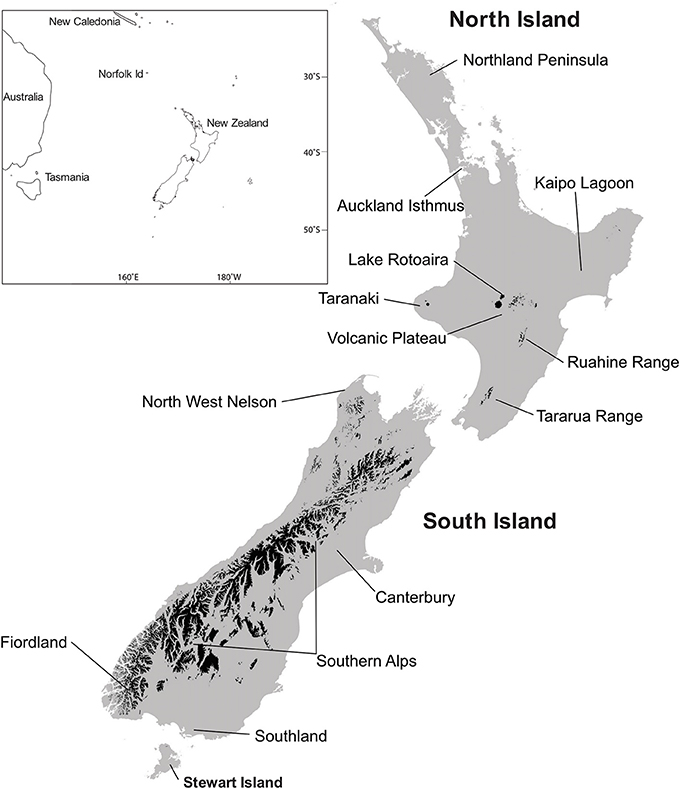
Figure 2. Localities mentioned in the text. Areas in black, above tree line. Baseline data, Landcare Research.
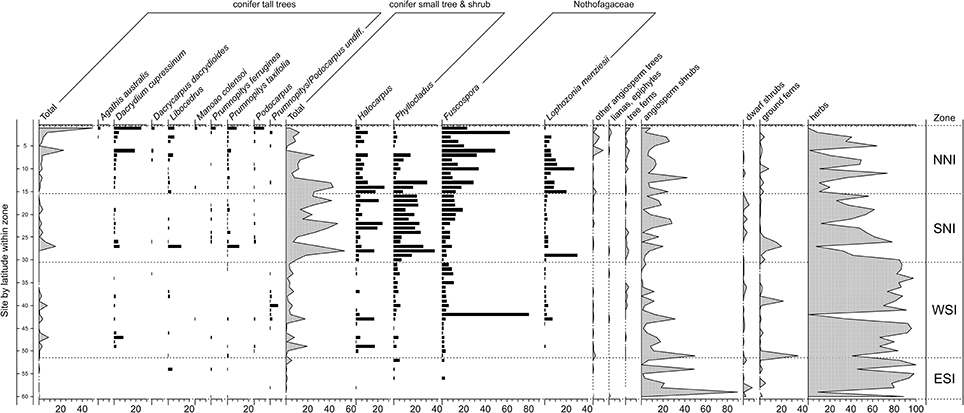
Figure 3. Pollen and spore results for representative samples within sites dating to the LGM. In descending order of increasing latitude within broad zones: northern North Island (NNI–north of latitude 37° S); southern North Island (SNI–south of 37° S but including the overlapping portion of the South Island); western South Island (WSI–west of the Southern Alps); eastern South Island (ESI–east of the Southern Alps). Pollen sum: all terrestrial types excluding ferns, lycopods, and wetland forbs, rushes, and sedges. After McGlone et al. (2010).
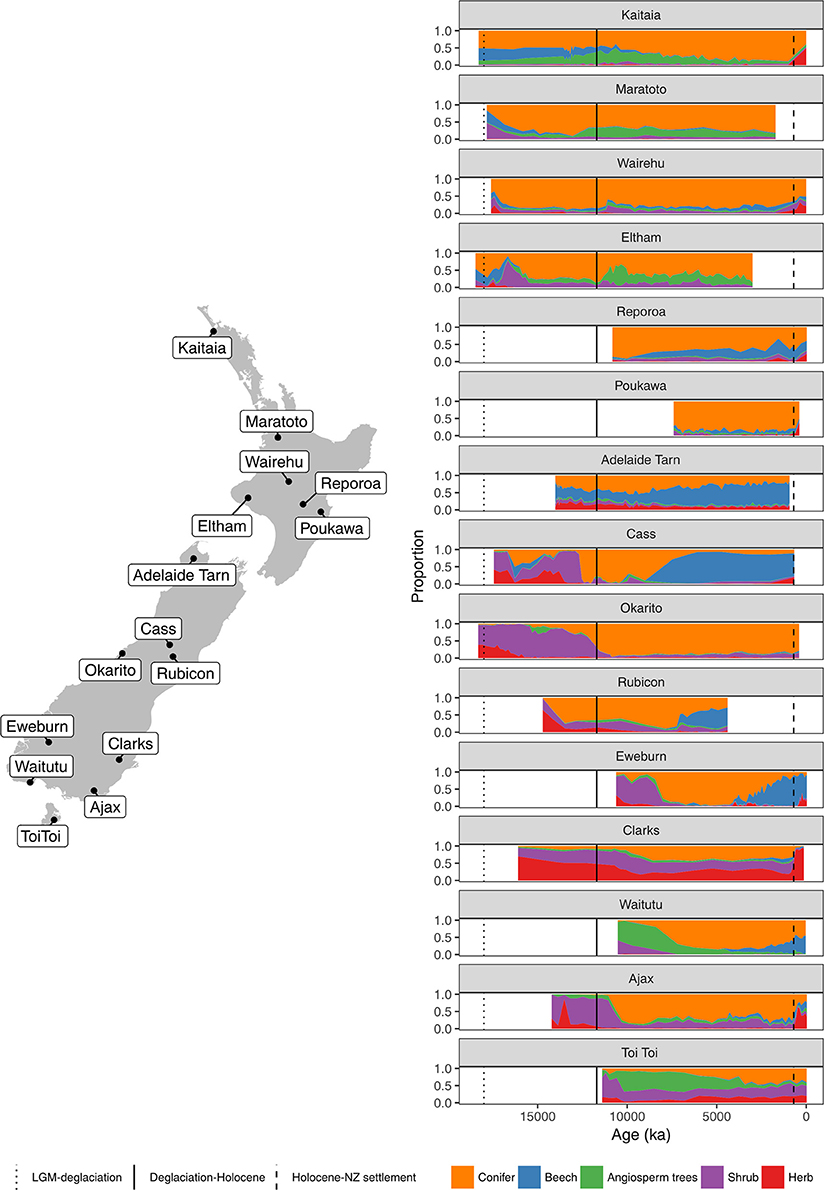
Figure 4. Summary percentage pollen diagrams (sum: all terrestrial types excluding ferns, lycopods and wetland forbs, rushes, and sedges) and site locations.
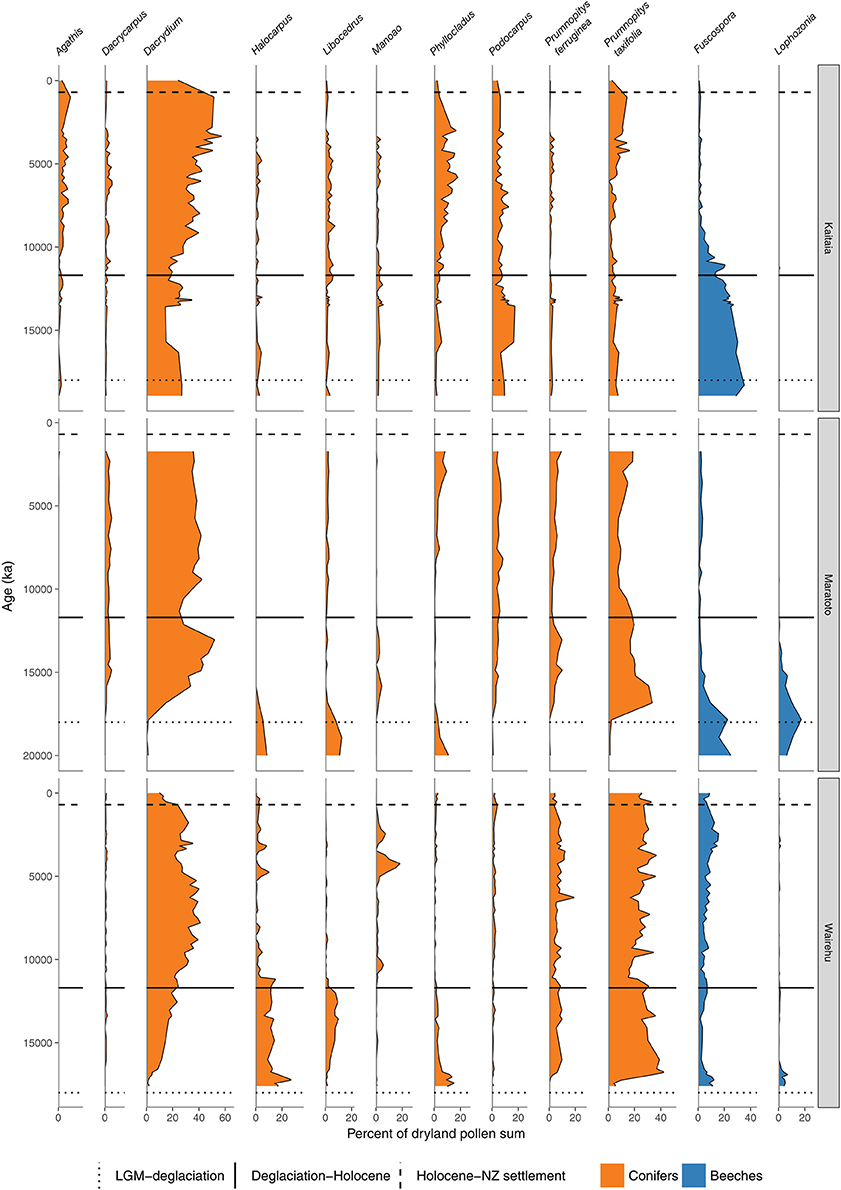
Figure 5. Conifer and Nothofagaceae pollen sequences. Kaitaia bog, Northland (Elliot, 1998). Lake Maratoto, Hamilton Basin (McGlone, 2001a). Wairehu, Rotoaira Basin (McGlone and Topping, 1977).
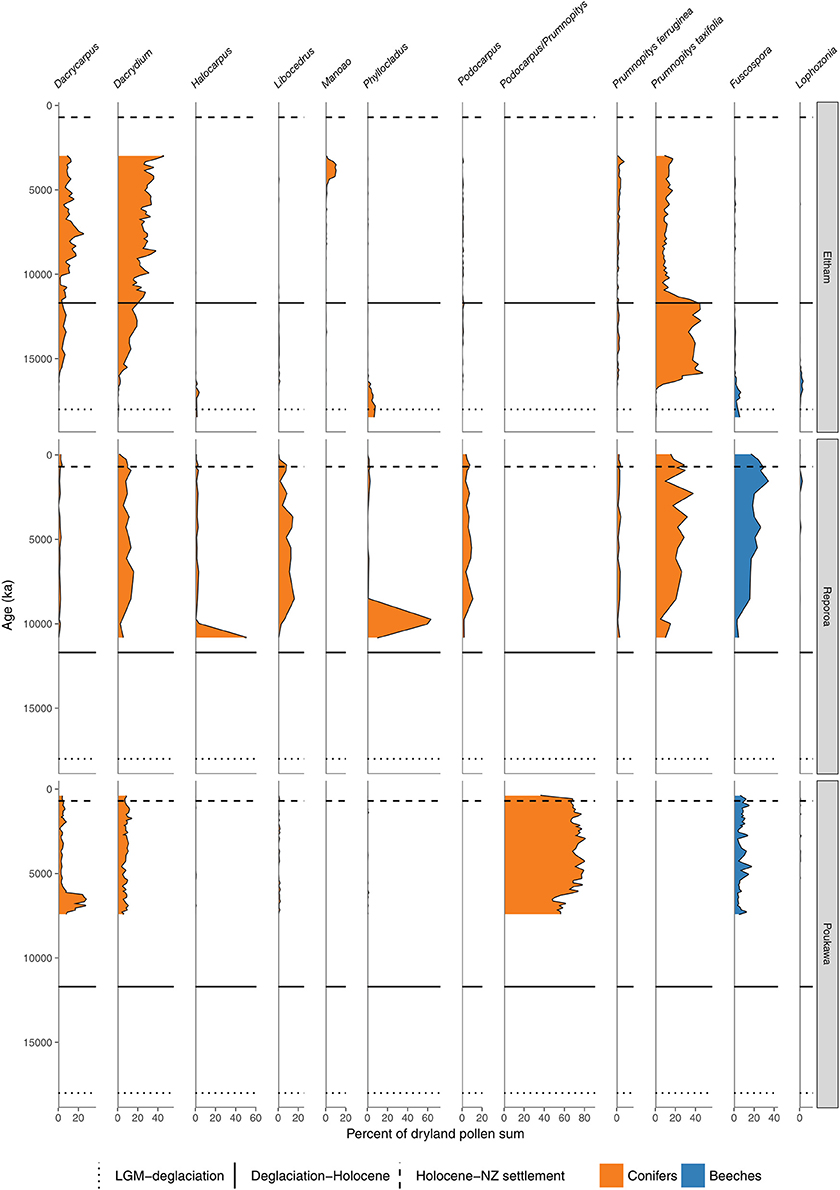
Figure 6. Conifer and Nothofagaceae pollen sequences (cont.). Eltham Bog, Taranaki (McGlone and Neall, 1994). Reporoa Bog, upland central North Island (Rogers and McGlone, 1989). Lake Poukawa, Hawkes Bay (McGlone, 2002).
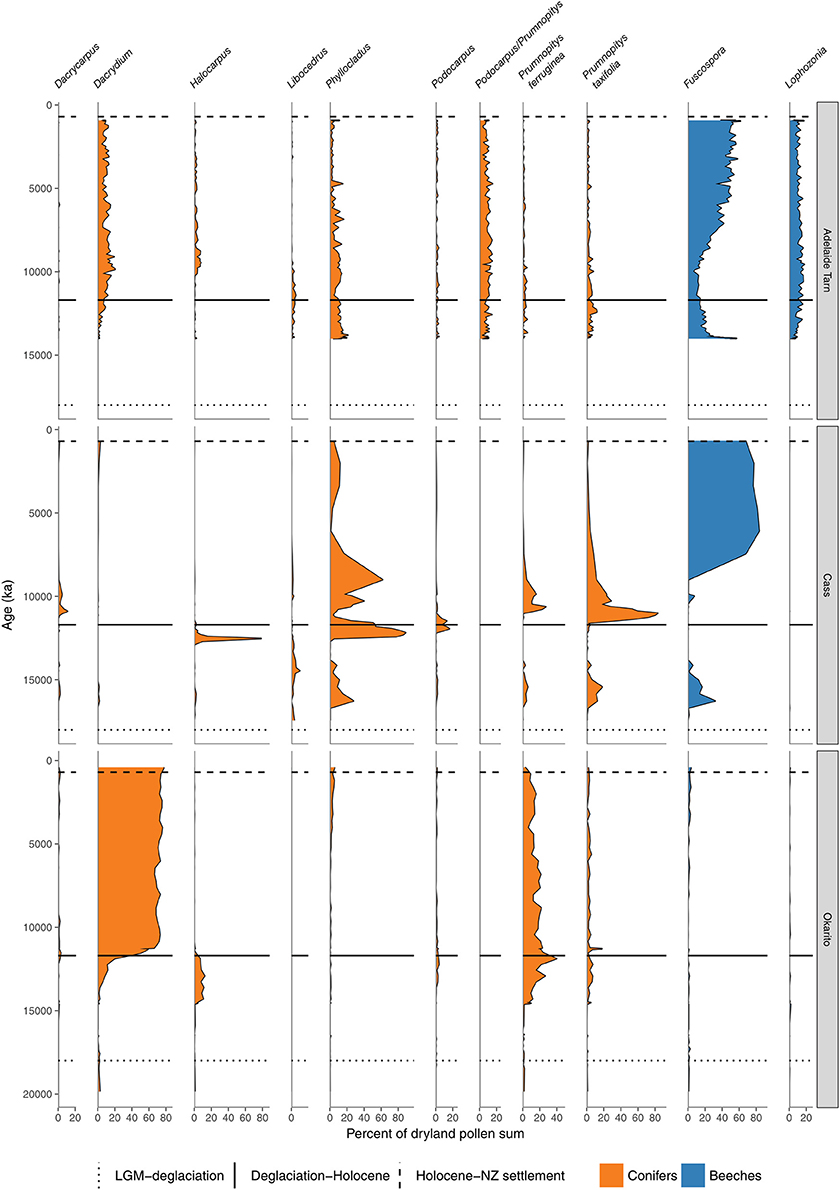
Figure 7. Conifer and Nothofagaceae pollen sequences (cont.). Adelaide Tarn, treeline, Northwest Nelson (Jara et al., 2015). Cass Basin, Kettlehole Tarn, inland Canterbury (McGlone et al., 2004). Okarito bog, central West Coast (Newnham et al., 2007).
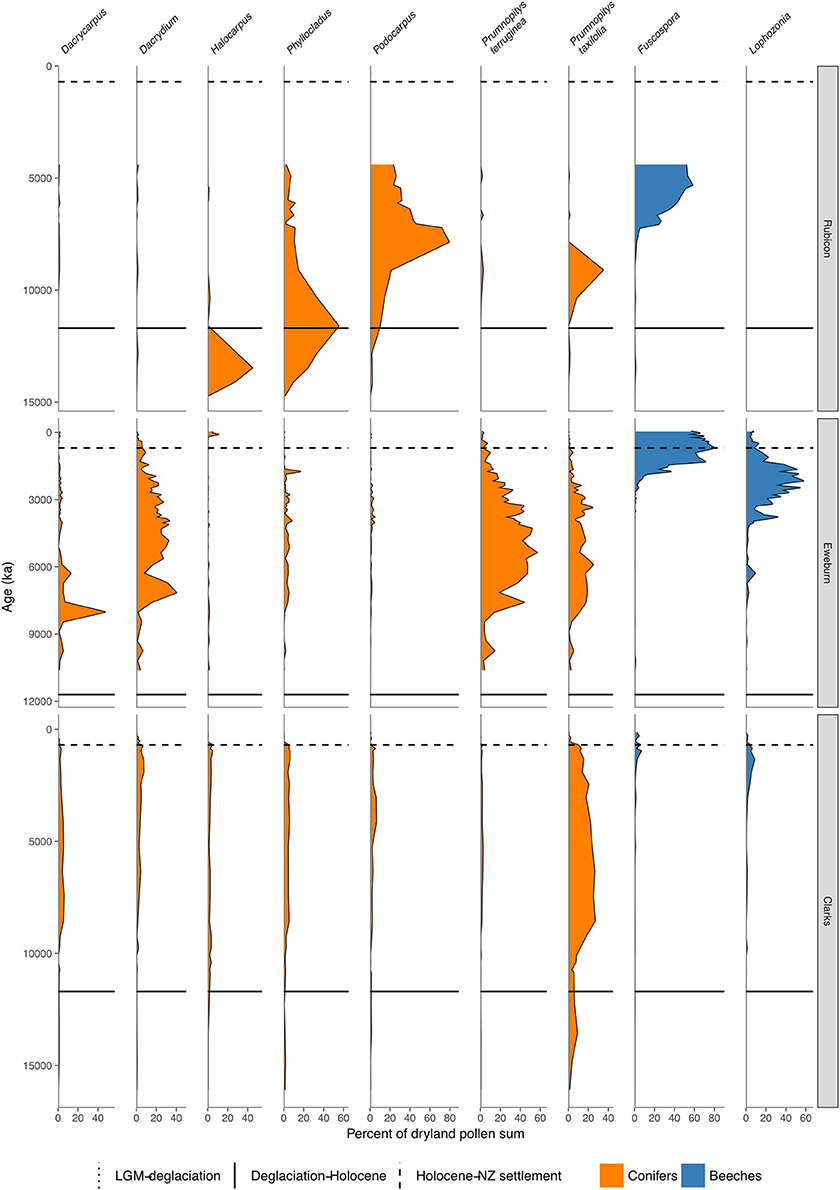
Figure 8. Conifer and Nothofagaceae pollen sequences (cont.). Rubicon River, inland Canterbury (Moar, 1973). Eweburn Bog, Southland (Wilmshurst et al., 2002). Clarks Junction, Otago (McGlone et al., 2003).
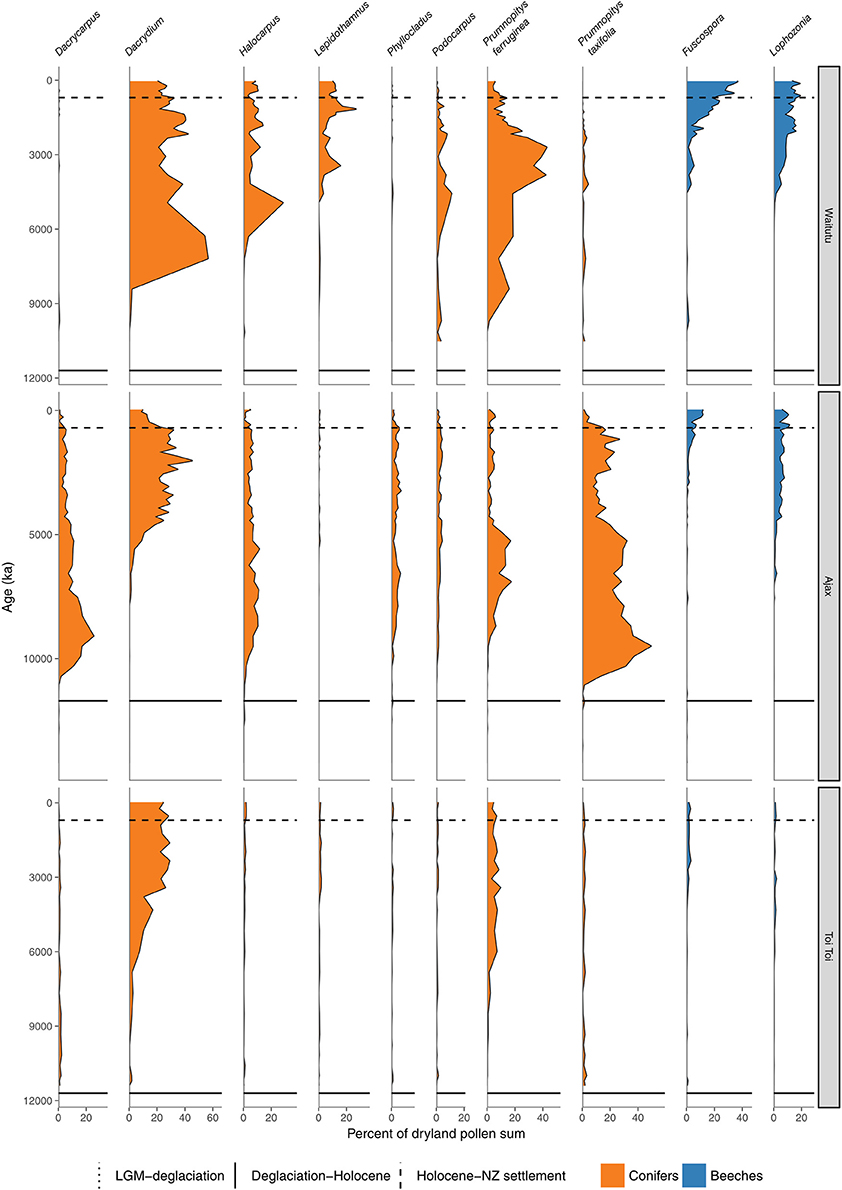
Figure 9. Conifer and Nothofagaceae pollen sequences. Waitutu, Fiordland (Turney et al., 2017). Ajax Bog, Southland (McGlone et al., 2003). Toitoi Flat, Stewart Island (McGlone and Wilson, 1996).
Last Glacial Maximum
The LGM (29 to 19 ka) (ka = thousands of calibrated radiocarbon years before 1950 CE) was the coldest period of the present glacial-interglacial cycle (Lorrey et al., 2012). During this period mean annual temperatures fell by 4–7°C (Newnham et al., 2013), glaciers advanced throughout the Southern Alps, extending below current sea level in the west. Overall precipitation was lower, perhaps by as much as a third (Alloway et al., 1992) and the prevailing westerly airflow meant that the rain shadow region east of the axial ranges became semiarid. The plains of the interior south-eastern South Island have been described as approximating a polar desert (McIntosh et al., 1990).
Last Glacial Maximum (LGM) pollen sequences (Figure 3) show a forested or partly forested northern third of the North Island (above c. latitude 38°). In Northland, although Nothofagaceae were the dominant tree cover (with abundant Lophozonia menziesii and Fuscospora truncata), tall conifers played an important role, particularly Dacrydium cupressinum (Newnham, 1992; Wright et al., 1995; Elliot, 1998; Newnham et al., 2017). From the Auckland Isthmus southwards, tall forest became sparser or confined to the coast while in the central districts of the North Island and the north of the South Island, conifer shrubland to low forest of Phyllocladus alpinus and Halocarpus bidwillii formed a mosaic with Nothofagaceae forest patches, broadleaved shrubland, and grassland. The western districts of the South Island, even those adjacent to the glacier fronts, had angiosperm shrubland-grassland cover, but also patches of low conifer forest, and sparse stands of tall conifers (Vandergoes et al., 2005). This vegetation type extended to coastal Fiordland in the far south of the mainland (Pickrill et al., 1992). In eastern lowland districts, grassland, low-growing angiosperm shrubland and sparse prostrate shrubs and herbfield were the main cover and conifers of any type were rare or absent over large areas although maintaining a regional presence (Moar, 1980; McGlone, 2002).
The Deglaciation (18 to 11.6 Ka)
A hemispheric warming and rapid retreat of glaciers began at about 17–18 ka following the last LGM advance at around 19 ka (Moreno et al., 2015; Darvill et al., 2016). Conifer-angiosperm forest spread in the central and northern North Island from 17 to 14 ka replacing previous forest-scrub-grassland mosaics (Figures 4–6). For instance, lowland forest expanded in the Auckland Isthmus between 15.5 and 14 ka (Sandiford et al., 2002, 2003; Newnham et al., 2007; Augustinus et al., 2011); at Kaipo Lagoon in the montane North Island, 16.5–14 ka (Newnham and Lowe, 2000), in lowland Taranaki at 15 ka (McGlone and Neall, 1994); and at Lake Rotoaira on the montane central Volcanic Plateau 16.5–15 ka (McGlone and Topping, 1977, 1983).
In the South Island, stands of forests expanded in what was still a largely grass and shrub covered landscape (Figures 4, 7–9). Northwest Nelson saw expansion of conifer forest at around 14.5 ka (Jara et al., 2015), and at Okarito on the west coast, between 15 and 14.5 ka (Vandergoes et al., 2005); at Cass Basin in inland Canterbury, 15.7–14.5 ka (McGlone et al., 2004) and at Clarks Junction, eastern South Island, 15.5–13.5 ka (McGlone et al., 2003). A minor reversal of this warming trend occurred between 14.5 and 12.9 ka with glacial readvances in the Southern Alps (Darvill et al., 2016). By this time, dense tall conifer forest had occupied all but the driest eastern districts of the North Island and extensive stands were present in the lowland South Island throughout. These early deglacial forest pollen spectra were dominated by Prumnopitys taxifolia (Figures 5–9) but with significant input from Phyllocladus, Libocedrus and, in places, the Nothofagaceous Lophozonia menzesii and the deciduous angiosperm tree Plagianthus regius were common. These trees are all frost-hardy (Bannister, 2007) and can tolerate a certain amount of drought, in sharp contrast to the angiosperms that became abundant in the early Holocene (e.g., Ascarina, Metrosideros) (Leathwick and Whitehead, 2001; Hall and McGlone, 2006).
The Holocene
The beginning of the Holocene period at 11.7 ka, marks the transition to true interglacial climates. Warming continued in New Zealand with increasing rainfall in the west, and the period between c. 11 and 8 ka was characterized by a greatly weakened westerly airflow (Shulmeister et al., 2004). The intensely oceanic climate promoted the spread of the small tree Ascarina lucida which cannot tolerate dry air or frost (McGlone and Moar, 1977; Martin and Ogden, 2005). A forest dynamic model was used to explore the climatic implications of a deglacial-Holocene pollen sequence from a montane rainfall spill-over area of the Southern Alps. Warmer than present winters, somewhat cooler summers, and less but more evenly spread rainfall were predicted for the early Holocene (McGlone et al., 2004).
In northern districts of the North Island, and western districts throughout, conifer forests with abundant Dacrydium cupressinum, Prumnopitys ferruginea, and Dacrycarpus dacrydioides and tree ferns dominated (Figures 4, 5). In rain-shadow eastern districts Prumnopitys taxifolia and Podocarpus spp. spread in lowland to montane locations (McGlone, 2002; McGlone et al., 2004), but low forest of Phyllocladus alpinus and Halocarpus bidwillii occupied the drier, frosty inland basins and hill slopes (McGlone and Moar, 1998). Stewart Island at the far south of the South Island was the last region where lowland conifers spread (Figures 4, 9, Toitoi). The early postglacial forests on Stewart Island were entirely dominated by broadleaved angiosperms and tree ferns. Although Stewart Island was connected to the mainland well into the early postglacial, neither Nothofagaceae nor Phyllocladus alpinus, both abundant on the adjacent mainland, are present on the island, suggesting climatic factors or climate-moderated competition prevented their establishment while land connections existed.
The Mid to Late Holocene
The intensely oceanic climates of the early Holocene gave way from 8 ka onwards to more seasonal regimes characterized by longer, cooler winters and shorter, but warmer, summers. Increased south-westerly wind flow over New Zealand brought increased winter rainfall (McGlone et al., 2004). This increased seasonality strongly favored some trees over others. In the far North, it is only post 8 ka that Agathis australis—the giant Araucarian forest dominant (Figure 1)—becomes universally common in the pollen rain (Ogden et al., 1992) at about the same time that Prumnopitys taxifolia, Podocarpus spp., Phyllocladus spp., and Libocedrus plumosa became also more prominent (Elliot, 1998; Newnham, 1999; Elliot et al., 2005) (Figure 5). Some caution is needed in interpreting Agathis fossil records as pollen and macrofossil occurrences may not match because of differential preservation, and thus fluctuations during the mid to late Holocene may simply reflect changing wetland watertables (D'Costa et al., 2009). In the very far southern Stewart Island, the conifers Dacrydium cupressinum, Prumnopitys ferruginea, Halocarpus biformis, and Lepidothamnus spp. began their spread into the previously dominant Metrosideros-Weinmannia broadleaf forests from about 6 ka onwards (McGlone and Wilson, 1996; Figure 9). On the adjacent south-eastern South Island mainland, Phyllocladus and Podocarpus low forest occupied the dry interior basin-and-range country only after 8 ka (McGlone et al., 1995, 1997).
The mid to late Holocene saw spread of Nothofagaceae in most districts (McGlone et al., 1996). There are two exceptions. The Northland Peninsula and the Auckland Isthmus had extensive Nothofagaceae forest, mostly Fuscospora truncata and Lophozonia menziesii during the LGM and early postglacial but this was replaced during the early Holocene by Agathis-podocarp-broadleaved communities (Elliot, 1998; Newnham et al., 2017). Lophozonia menziesii formed part of the lowland deglacial forests in the central North Island but was eliminated before the beginning of the Holocene by conifer-broadleaved forests (Newnham et al., 1989, 1999; Alloway et al., 1992). Nothofagaceae at the LGM occurred only in scattered patches in the far south but had a more substantial presence in coastal areas of the north-west of the South Island (Marra and Leschen, 2004). Fuscospora spread appears to have started more-or-less synchronously throughout the uplands of the central and southern North Island and northern South Island during the early Holocene (Figures 4, 6–9). Fuscospora forests are currently the most common cover of the uplands and axial ranges of the central and southern North Island and northern South Island, but only became treeline dominants from about 9 to 3 ka, depending on the site (Rogers and McGlone, 1989; McGlone et al., 1996; Jara et al., 2015). Fuscospora cliffortioides—the most abundant tree line forest tree—almost invariably spread into pre-existing alpine forests and shrubland of Libocedrus bidwillii, Phyllocladus alpinus, and Halocarpus spp.
Lophozonia spread after c. 7–6 ka across the south of the South Island in widely separated areas mainly upland treeline sites, but including montane-lowland conifer-broadleaved forest (McGlone et al., 2003). Where Lophozonia took part in these mid to late Holocene successions in lowland to lower montane settings, it mostly spread into conifer-broadleaved forest where Prumnopitys ferruginea or Dacrydium cupressinum were abundant (see Eweburn, Figure 8). In these lowland-montane sites, Fuscospora spp. follow the initial invasion or spread by Lophozonia.
Late Holocene and Polynesian Fire
Fire occurred frequently on the large, raised restiad bogs of northern New Zealand (Newnham, 1992; Battersby et al., 2017; Haenfling et al., 2017) but elsewhere was sporadic. Along the rain-shadow regions in the ranges to the east of the Southern Alps fires burnt from time to time, inducing a patchy landscape of conifer low forest, shrubland and grassland (Burrows et al., 1993; Burrows, 1996; Wardle, 2001b; Pugh and Shulmeister, 2010). Fire frequency may have increased at around 3 ka in some eastern parts of the North and South Islands but was still infrequent (McGlone and Moar, 1998; Ogden et al., 1998; Horrocks et al., 2001; Woodward et al., 2014). Few New Zealand woody plants have significant adaptations to fire (Perry et al., 2014) and conifers in particular appear to be highly vulnerable to fire. A notable exception is Halocarpus bidwillii, which has thick bark and can recover through basal resprouting after fire (Wardle, 1991). Polynesian fires beginning in the late thirteenth century, ultimately removed about 40% of the montane and lowland forest cover (McWethy et al., 2010; Perry et al., 2014). This forest loss was concentrated among conifer-rich lowland forests where c. 30% of this type was lost in the North Island, and nearly 90% in the South Island (Perry et al., 2012a). Some offshore islands were thought never to have had conifer forest, but pre-Polynesian pollen sequences have demonstrated that they did (Wilmshurst et al., 2014).
A Glacial-Interglacial Perspective on the Environmental Niche of the New Zealand Conifers
Ecological Niche
An outline of the ecological niche of the conifers has been given in Table 1, and in Table 2 we summarize how the changing climate and seasonality over a glacial-interglacial cycle has shifted the distribution of broadly defined conifer vegetation groupings. The loss of 80% of New Zealand's lowland forests since human settlement, along with nearly all the forest from rain shadow eastern districts, has left wet conifer-broadleaved forests and montane to alpine Nothofagaceae dominant forests as the most common forest types. Our understanding of their niches derives mainly from ecological observations made in dense, wet forests—which do not fully cover the environmental range of most of the species—and correlations between environmental variables and their abundance in these same forests. Nevertheless, a number of statements can be made about New Zealand conifer ecological niches (see Coomes and Bellingham, 2011) which are likely to be robust.
New Zealand conifers are slow growing and long-lived in comparison with competing angiosperms (Ogden and Stewart, 1995) and markedly taller. Despite conifers making up only 8% of the tree flora, 33% of the trees growing 20 m or more in height are conifers. As a group, the conifers are markedly frost-tolerant, most resisting frosts of −7°C or more, and the three most frost-tolerant trees and shrubs in the flora (Halocarpus bidwillii, Phyllocladus alpinus, and Podocarpus nivalis) are conifers (Bannister, 2007). With regard to low rainfall and drought, Prumnopitys taxifolia, Podocarpus laetus (formerly P. hallii), P. totara, and Dacrycarpus dacrydioides are among a small group of trees singled out as currently having their maximum abundance under wet climate regimes, but also being capable of tolerating dry, warm lowland sites (Leathwick and Whitehead, 2001). Agathis australis, grows best under drier summer conditions and can tolerate severe drought (Macinnis-Ng et al., 2016). In particular, these species can tolerate low atmospheric deficits. Dacrydium cupressinum and Prumnopitys ferruginea are, on the other hand, far less tolerant of both dry soils and atmospheric deficits. Some species have an ambiguous relationship to drought: Dacrycarpus dacrydioides can tolerate warm, dry lowland situations (Leathwick and Whitehead, 2001) but physiological measures show it has a very low tolerance of water deficit (Brodribb and Cochard, 2009) and remaining stands are often associated with wet soils (Figure 1).
New Zealand conifers are generally regarded as being tolerant of poor soils (Coomes and Bellingham, 2011; de Jonge et al., 2012), and have an affinity for leached, low nutrient, acid or poorly drained soils that form in ever-wet environments and some (Dacrydium cupressinum, Dacrycarpus dacrydioides, Lepidothamnus intermedius, Manoao colensoi, Libocedrus plumosa, Halocarpus bidwillii, H. biformis) are characteristic of such sites (Richardson et al., 2005b). Where the climate supports tall trees, conifers usually dominate the tree biomass as there are only three tall angiosperm trees that tolerate wetlands (Elaeocarpus hookerianus, Laurelia novae-zelandiae and Syzygium maire; McGlone, 2009). Pollen diagrams confirm this and peat sites usually show conifer sequences with Dacrycarpus dacrydiodes at the fertile, often swamp or lagoon beginning of the sequence, and Dacrydium cupressinum, Manaoa colensoi, Lepidothamnus intermedius, and Halocarpus bidwillii at the infertile bog later stages (McGlone, 2009). However, in some situations conifers are quick to colonize fertile soils after disturbance, losing ground to angiosperm broadleaves as the succession proceeds, and this is most apparent in the pollen record after large-scale volcanic disturbance (Wilmshurst and McGlone, 1996; Horrocks and Ogden, 1998) but it also occurs after smaller scale disturbances (Bray, 1989; Carswell et al., 2007; de Jonge et al., 2012).
This tolerance of frost, drought, dry air and low nutrient or water-saturated soils can to a certain extent be attributed to their narrow, embolism-resistant tracheids, conservative hydraulic systems and thick, narrow leaves which lead to slow growth relative to competing angiosperms but much greater stress tolerance of poor soils, cold and drought (Sperry et al., 2006). This combination of attributes is the key to conifer niche over both long and short timescales. Despite slow growth rates, longevity ensures that the crowns of New Zealand forest conifers eventually rise well above the continuous lower broadleaved canopy. They therefore spend most of their life span with their crowns exposed to high solar radiation, higher wind speeds and low humidity which induce a drying effect exacerbated by the physiological water transport stress that scales with height (Koch et al., 2004). For instance, tropical emergent trees transpire most of the water used in the forest they form part of (Kunert et al., 2017). A second, related fact is that New Zealand conifers within a conifer/broadleaf tract are often most abundant on ridges and steeper slopes exposing their canopies to windier, less humid conditions and drier soils. Just a handful of tall angiosperms compete in this supracanopy emergent space (e.g., Laurelia novae-zelandiae, Metrosideros robusta, Knightia excelsa, Fuscospora fusca, and F. truncata), and it has been argued that the angiosperm and conifer components of the forests they co-occur in are largely independent of each other (Ogden, 1985; Lusk, 2002).
Regeneration
New Zealand conifers have long been believed to face severe regeneration problems (Cockayne, 1928; Holloway, 1954; Robbins, 1962; Wardle, 1963; Veblen and Stewart, 1982; Smale et al., 2016). Most are bird-dispersed and dispersal seems not a critical issue. The wind-dispersed Agathis australis and Libocedrus spp. appear to have more limitations, and the montane to alpine Libocedrus bidiwillii has a markedly discontinuous distribution (Wardle, 2011), but even so they regenerate well after disturbance (Veblen and Stewart, 1982; Steward and Beveridge, 2010). The essential problem faced by the conifers in lowland forests is establishment in openings that quickly fill with tree ferns and fast-growing and/or vegetatively resprouting angiosperm trees. This difficulty is compounded by conifers rarely recruiting under closed canopies (Ogden and Stewart, 1995). A marked feature of most New Zealand conifers is distinct juvenile foliage or growth forms (Dorken and Parsons, 2016)—most strikingly with the divaricate branched juvenile Prumnopitys taxifolia, the drooping foliage of Dacrydium cupressinum and the pyramidal “ricker” juvenile of Agathis australis. It is at least plausible that these monopodial juvenile growth forms compensate for slow biomass accumulation by favoring a single stem axis while the often elongated, planar or dispersed leaves maximize photosynthesis in a complex light environment. Although the New Zealand conifers are generally considered to be shade-intolerant (Cameron, 1954; Ebbett and Ogden, 1998), experimental studies suggest that this intolerance varies between taxa. Lusk et al. (2009) report little relationship between light availability and seedling presence of Dacrydium cupressinum and Prumnopitys ferruginea in forest stands in the central North Island. Observations in a northern North Island conifer-broadleaved forest showed that while the conifers had much the same shade tolerance as their angiosperm competitors, they grew more slowly and it was only at forest edges that their greater stress tolerance allowed them to overcome this regeneration handicap (Lusk et al., 2015). Conifer regeneration in drier regions is poorly known. However, we can postulate that drier, relatively infertile sites have sparser understoreys and ground-layers, providing better opportunities for conifer regeneration (Wardle, 1963; Burns and Leathwick, 1996) and the greater stress tolerance of the adult trees permit them to dominate.
John Ogden proposed an influential model in which successive generations of conifers form a lesser proportion of a conifer-broadleaved forest due to recruitment difficulties until a large-scale disturbance resets the forest with thickly stocked conifer stands (Ogden, 1985). Some recent data suggests higher conifer mortality and slower replacement in central North Island forests affected by volcanic eruptions consistent with this model (Smale et al., 2016), but complexities of forest history make sweeping generalizations inadvisable. For instance, broad scale analyses of North Island forests have shown some of the largest conifers have lower mortality rates than angiosperm trees (Richardson et al., 2009), and selective logging of conifers from wide tracts of conifer-broadleaved communities has given rise to anomalous contemporary patterns with virtually no regeneration in some areas (driven by absence of conifer seed sources) vs. massive regeneration in others (Carswell et al., 2007).
The pollen record seems to only detect the very largest of disturbances because of its typically large spatial and temporal scales. Once these are factored in, there appears to be little overall trend in conifer-broadleaved forest toward angiosperm dominance. There is little signal in the deglacial and Holocene pollen record from extant conifer-broadleaved tracts that conifers have ever been reduced to low levels (Figures 4, 5–9). During the first few thousand years of conifer spread during the deglacial period, conifer pollen input appears to have been higher than subsequently: most sites show this period of great conifer abundance did not last. However, in those areas that have remained under conifer-broadleaved forests throughout the Holocene, the conifers appear to have always been abundant. There is one exception. During the late deglacial-early Holocene period, coastal southern South Island and Stewart Island appear to have had a broadleaved-tree fern community with Weinmannia racemosa and Metrosideros umbellata prominent members, and conifers all but excluded for an extended period (Pickrill et al., 1992; McGlone and Wilson, 1996). Conifer dominance was not established until well into the mid Holocene (Figure 9). This island has extremely oceanic climate by global standards (Meurk, 1984) which the low insolation, warm winter-cool summer regime of the early Holocene intensified. The conifer strategy, which relies, in the absence of landscape-level disturbance, on environmental conditions unfavorable to angiosperms to regenerate well, was negated. A recent parallel at a more local level may be found in some fertile, dark, moist gullies where tree ferns, palms and broadleaves appear to permanently exclude conifer regeneration.
Conifers and Drylands
New Zealand conifers extend into semi-arid areas (rainfall <500 mm a−1) and were dominant across a wider “dryland” (Penman deficit ≥ 270 mm a−1) region (Figure 10) mainly to the east of the axial ranges that made up some 19% of the land area of New Zealand prior to human settlement in the thirteenth century (Rogers et al., 2005). Because dryland conifer forest was largely destroyed by Polynesian fires (Perry et al., 2012b) little is known about its ecology. Figure 11 shows how human clearances have reduced the representation of current forests to vestigial levels in areas with less than about 900 mm a−1 rainfall, and how conifers dominated the pre-deforestation pollen rain in this dryland zone. The pre-deforestation extension of taxa, such as Dacrycarpus dacrydioides, Halocarpus, Podocarpus, Phyllocladus, and Prumnopitys taxifolia into the dryland zone is particularly clear and thus palynological investigations have focused much more on this zone than have ecological ones.
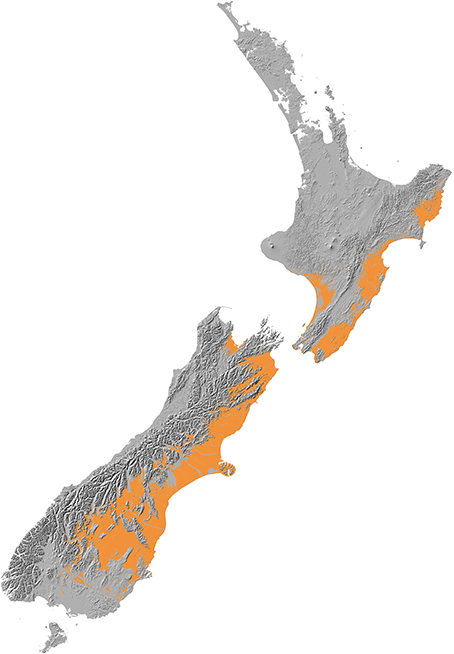
Figure 10. Dryland zone. Modified from Walker et al. (2009). Baseline data held by Landcare Research.
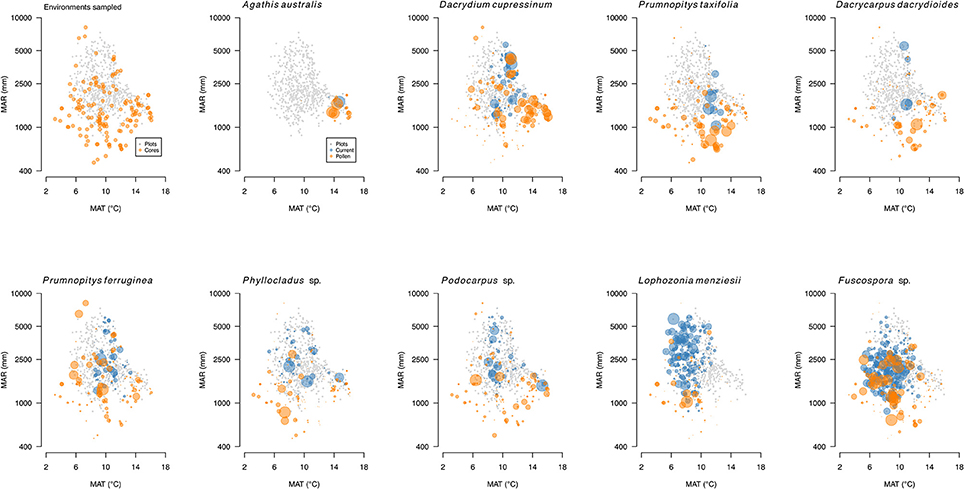
Figure 11. Pollen (orange) and basal area biplots (gray) for conifers and Nothofagaceae. Pollen percentages (orange) based on terrestrial sum excluding ferns and lycopods. Basal areas for trees (blue) from the LUCAS programme national Natural Forest plot (20 × 20 m) data. Data on individual panels scaled relative to largest value.
Conifer forests were more widespread during the early deglacial and Prumnopitys taxifolia and Podocarpus spp. dominated the transition from shrubland-grassland to closed forests (Mcglone and Bathgate, 1983; Mcglone and Topping, 1983; Newnham et al., 1989; Vandergoes et al., 1997; Sandiford et al., 2003; Augustinus et al., 2012; Jara et al., 2015). These early Prumnopitys and Podocarpus forests were not accompanied by an abundance of tree ferns, nor significant amounts of tall angiosperm trees, as the later Dacrydium dominated forests were and still are, and thus it seems that the early deglacial was drier than now. Dryland pollen sequences show that the forests existing just before deforestation strongly resembled the first forests to establish after the early deglacial Myrsine-Muehlenbeckia shrubland/grasslands, and then changed little during the Holocene (McGlone et al., 2003). In the driest areas of the southeastern South Island, conifer-broadleaved forest co-existed with patches of grassland and shrubland (McGlone, 2001b; Walker et al., 2004a; Rogers et al., 2005). It was only on the wet, western flanks of the Southern Alps (where Dacrydium cupressinum was abundant; Newnham et al., 2007) and Northland (where Fuscospora was abundant) that this classic tall dryland conifer forest failed to establish.
A generalized dryland pattern that prevailed just before the Polynesian deforestation can be reconstructed from macrofossil and pollen data (Molloy, 1968; McGlone et al., 2003). The pre-deforestation pollen rain of the drylands was largely dominated by conifers (Figure 11), and the drylands may have supported denser conifer stands than elsewhere (Hall and McGlone, 2006). Tall Prumnopitys taxifolia- and Podocarpus totara-dominated associations on the lowland, deeper, more fertile soils extended up into inland valleys in higher rainfall areas, with Dacrycarpus dacrydioides prominent on swamp or lagoon soils. Stonier, shallower soils throughout were dominated by Podocarpus laetus, especially on the midslopes of the inland valleys. Areas with cold winters and dry, droughty summers would have favored Phyllocladus alpinus dominance, with the most leached or acid soils carrying a cover of Halocarpus bidwillii shrubland. Prumnopitys taxifolia dominated the dryland pollen rain in most places, but there are only a few macrofossil or charcoal remains to corroborate this dominance. Podocarpus laetus (like the other Podocarpus species) is not well represented in the pollen rain, but occurs as a continuous component alongside Prumnopitys taxifolia. Preserved wood of Podocarpus laetus is widespread on tussock-clad hill slopes throughout eastern districts and is often accompanied by Phyllocladus alpinus charcoal (Molloy et al., 1963; Ogden et al., 1998; Wardle, 2001b). Pollen and charcoal show that the driest regions of the central southeastern South Island, and intermontane valley bottoms of the eastern central Southern Alps had Phyllocladus alpinus and Halocarpus bidwillii low forest to scrub cover.
A wide range of angiosperm shrubs and trees co-existed with the dryland conifers but only a handful of these were tall or capable of dominating forest tracts. The few remaining fragments of dryland forest suggest that they would have been heavily stocked with conifers over a low and subordinate canopy of small angiosperm trees, such as Melicytus ramiflorus, Griselinia littoralis, Elaeocarpus hookerianus, and Hoheria angustifolia. Most of these species are in the 10–15 m height range, with only Sophora microphylla, Kunzea spp., and Plagianthus regius taller (15–20 m), but even they rarely match the 20–50 m heights of the podocarps. The Kunzea species complex of Myrtaceaeous small leaved (leptophyll), wind-dispersed shrubs to tall trees formed extensive forest tracts: the taller Kunzea (K. serotina, K. robusta, and K. ericoides) range throughout the mainland islands and occupied the very driest sites but generally they do not form an integral part of mature conifer forest; rather they occur as ecotonal or early successional dominants (de Lange, 2014). Interestingly, their small, linear needle-like leaves, erect multi-branched form, ectomycorrhizal status, ability to occupy bare ground, relatively fast growth and resistance to stresses, and rapid recovery after disturbance, including fire, is strongly reminiscent of Northern Hemisphere Pinus. It is unlikely that the dryland conifers were as dependent for regeneration on large-scale disturbance as they are in denser, moist forests, as these dryland forests lacked a dense understory, ferns being particularly sparse.
Conifers and the Nothofagaceae
A striking feature of many pollen profiles from the New Zealand mainland south of the Northland Peninsula, and in particular along the axial ranges, is the rise to dominance over the postglacial period of the Nothofagaceae (Figures 4, 5–9). On the basis of their Southland pollen diagrams, Cranwell and von Post (1936) divided the New Zealand postglacial into three periods: (I) Grassland; (II) Podocarp forest; (III) Nothofagaceae forest and grassland mosaic period. Period I was regarded as the final stages of the glaciation, with cool, severe climates with little regional differentiation; Period II, wet with probably maximum warmth; and Period III, climatic deterioration (Cranwell, 1938). That cooling climates had driven the spread of Nothofagaceae was readily accepted at first because Fuscospora cliffortioides and Lophozonia menziesii favor cooler regions and climatic explanations for anomalous Nothofagaceae distributions became popular for a time (Holloway, 1954; Nicholls, 1956). However, it had also been long known that the Nothofagaceae have a number of broad gaps in their distribution (the Taranaki volcanoes, the central and highest section of the Southern Alps, central Southland and Stewart Island; Cockayne, 1928). Willett (1950) suggested that the forest cover is in a state of disequilibrium because of the lasting effects of exclusion of Nothofagaceae trees at the height of the LGM by ice or severe climates and thus attributed the long-term forest dynamics of glacial affected areas to the slow, but inexorable encroachment of Nothofagaceae trees into conifer-broadleaf dominated associations. The Nothofagaceae have winged, but poorly dispersed seeds and a requirement for ectomycorrhizal infection (Wardle, 1984) and thus dispersal limitation became the favored explanation for Nothofagaceae gaps at all scales (Molloy et al., 1963; Burrows, 1965; Moar, 1971). Palynological evidence suggests that neither of these explanations—glacial exclusion or deteriorating climates—is sufficient alone. To see why, we have to look at how the environmental niche of Nothofagaceae and conifers differ.
New Zealand Nothofagaceae, in general, occupy sites with cooler mean annual temperatures than the tall tree conifers (Leathwick, 1995) and dominate most current tree lines (Case and Duncan, 2014). While overlapping to a large extent with conifers in their environmental tolerances (Figure 11), and thus co-existing over wide areas, Nothofagaceae are mostly absent from the warmest locations—mainly the north of the North Island and southern North Island lowlands. Like conifers, they are vulnerable to having their regeneration supressed by fast-growing broadleaves under warm, moist climates but lack the slow-growing emergent strategy that permits conifers to persist in dense broadleaved forests (Lusk et al., 2013). They are also largely absent from the drier, more drought-prone, frostier regions where several tall conifer trees (most notably Podocarpus laetus and Prumnopitys taxifolia) are abundant. However, ecosystem models predict that Nothofagaceae should dominate over most of its range gaps (Hall and McGlone, 2001, 2006; Leathwick, 2001).
The absence of Nothofagaceae from the seasonally dry and frosty areas, such as the south-eastern South Island, even though model results project its presence, seems unproblematic once the limitations of the regeneration niche (which is not explicitly included in the models) is understood. The models project Nothofagaceae dominance because it is quite frost resistant but also fast-growing, and excellent at colonizing slips and clearings (Wardle, 1984; Richardson et al., 2011). However, they are poor at regenerating in grassland—where their sensitivity to late season dryness appears to be a factor—and are subject to local dispersal and mycorrhizal limitations. As Wardle (1984, pp. 381–382) comments, “Beech seedlings are poor competitors and have difficulty in establishing where the forest understory is dense, especially where turf or fern covers the ground. Young seedlings are prone to unseasonal frosts and both winter and summer desiccation. Even saplings over 2 m high can be killed by winter desiccation. Establishment success is therefore usually poor in the open, particularly where the climate tends to be cold and dry.”
If difficulties with establishment are overcome, Nothofagaceae have on average more rapid height growth and biomass accumulation than do competing conifers (Wardle, 1991). This advantage is particularly marked at tree line in relation to the competing conifers Podocarpus laetus, Phyllocladus alpinus, and Halocarpus biformis. In upland Nothofagaceae forest, Phyllocladus alpinus and Halocarpus spp. are often confined to the more stressed sites, such as frost-prone terraces and valley heads (Wardle, 1985) or on poorly drained soils. In broad terms, the Nothofagaceae have higher photosynthetic rates and therefore are well equipped to take advantage of high insolation days and short summers (Richardson et al., 2005a; Whitehead et al., 2011). The Nothofagaceae are also ectomycorrhizal and this brings with it the ability to directly use nutrients locked into organic complexes and also to lower the nutrient content of organic soils to such an extent that they are largely unsuitable for trees with arbuscular mycorrhizal infection (Dickie et al., 2014). However, these advantages over conifers are lessened under cloudy, low insolation summers (growth rate premium reduced) and long, mild winters (breakdown of soil organic matter and release of nutrients enhanced). When this is the climatic regime, other angiosperm broadleaved species prevail, dense fern groundcover forms restricting regeneration of the Nothofagaceae seedlings. We thus have the early Holocene conifer-dominant treeline associations of Phyllocladus alpinus, Halocarpus bidwillii and H. biformis, Podocarpus laetus and P. nivalis, and Libocedrus bidwilli (McGlone et al., 2011; McGlone and Basher, 2012) remnants of which persist throughout the axial ranges but most commonly in the central Southern Alps. There is a strong association of these conifer-dominant treelines with low insolation situations, such as in the southern Ruahine and northern Tararua Ranges (Rogers and McGlone, 1994), but limited Nothofagaceae spread across alpine-montane valley systems most likely contributed to this pattern (Wardle and Lee, 1990; McGlone et al., 1996; Hall and McGlone, 2006).
Mixed Nothofagaceae-conifer tall lowland forest is common in some regions, but most prevalent in the north-western South Island. Trade-offs in growth-rate and regeneration strategy can facilitate their long-term co-occurrence (Lusk and Smith, 1998). However, the propensity of Nothofagaceae to form monospecific stands means they are either segregated by site from conifers—with Nothofagaceae along river courses or ridges—or the tall conifers (mainly Dacrydium cupressinum, Prumnopitys ferruginea, P. taxifolia, and Podocarpus laetus) form a discontinuous, sparse overstratum (Wardle, 1984, 1991). Only rarely are isolated Nothofagaceae trees scattered in conifer-broadleaved forest (Wardle, 1984). Holocene pollen sequences from the wide Nothofagaceae gap in central western South Island show that these boundaries have been largely static over the mid to late Holocene both at the Fuscospora dominated northern edge (Pocknall, 1980) and in the Lophozonia menziesii dominated southern margin of the gap (Li et al., 2008). Ecosystem models also predict mixed conifer-Nothofagaceae associations in both regions (Hall and McGlone, 2006).
New Zealand Conifers from a Global Biogeographic Perspective
New Zealand Conifers: Relictual?
Extant New Zealand conifers are clearly not relic. They are ubiquitous within the New Zealand mainland, and their close relatives thrive in similar oceanic forest environments elsewhere (McGlone et al., 2016). Their ecological success should not be measured by their species richness, but by their dominance of the forest biomass (Bond, 1989) and on this measure they are the most successful plant group in New Zealand. The lineages from which they come mostly evolved during the Palaeogene-early Neogene (Pittermann et al., 2012; Yang et al., 2012; Lu et al., 2014). Some are quite recent: the alpine-upper montane shrub and small tree group of P. lawrencei (SE Australia), P. gnidioides (New Caledonia) and P. nivalis (New Zealand) is indicated by molecular clock methods to have originated <5 ma ago (Condamine et al., 2017) and this is consistent with the timing of the uplift of the Southern Alps of New Zealand (Heenan and McGlone, 2013). Prumnopitys taxifolia (New Zealand) and P. andina (southern South America) are a closely related two-species clade, and may have diverged only 10 ma ago. The New Zealand conifers are therefore not holdovers from pre-angiosperm or even pre Gondwana-separation times but represent continuing adaptation to changing environments and competitors. However, that said, rainforest conifers have had to compensate for the structural and physiological limitations imposed by their hydraulics based on narrow tracheids, narrow, simple veined leaves, sluggish stomatal responses and, overall, low photosynthetic and growth rates (Brodribb et al., 2012). They do this by leveraging the inbuilt stress tolerance these attributes bring with them, and the longevity that accompanies their slow growth rate [hence Bond's (1989) “tortoise” analogy].
Given conifer dominance in many different New Zealand forest types we suggest that, rather than having severe disadvantages, they have been extraordinarily successful in a range of niches characteristic of an oceanic temperate environment. The biology and biogeographic history of the New Zealand conifers therefore can only be understood against the constraints and opportunities presented by the uncommon climate and unusual geographic position of the New Zealand archipelago.
Adaptation and Historical Contingency
Despite sharing its highly oceanic, moist climate regime with restricted temperate coastal regions elsewhere on the margins of the major continents New Zealand is, from a biogeographical viewpoint, unique. The archipelago has the only large temperate islands that are remote from a continental mainland and therefore never connected during the course of a glacial-interglacial cycle. Small, oceanic landmasses experience muted climatic extremes—both seasonally and at glacial-interglacial scales. However, during the extreme cold and dry of a glacial maximum, the archipelago has to fall back on its own floristic resources as it has no access to permanent cold boreal, arctic or dry steppe zones. Even New Zealand treelines cannot fill the gap, as they have exceptionally mild climates (Cieraad et al., 2012; Cieraad and McGlone, 2014). The trees of New Zealand, therefore, are evolutionarily adjusted to a limited climatic range. The brief intervals of a few thousand years during which the southern third of the archipelago experiences glacial cold, dry climates merely punctuate an otherwise highly oceanic environment.
Given the double filter of oceanicity and isolation, do New Zealand conifers represent unique adaptations to the oceanic climate regime? And has historical contingency played a role in that some niches have not been filled? Globally, oceanic temperate forests are extraordinarily diverse in terms of their structural and taxonomic makeup (DellaSala, 2011). They include single canopy deciduous angiosperm forests of the western fringe of Europe, dense, conifer-dominant forests of north-western North America, tall evergreen Eucalyptus forests of eastern Australia, but also structurally and taxonomically similar southern conifer-evergreen angiosperm forest of the southern Atlantic coast of Brazil, southern Chile and the highlands of New Guinea. Near-identical oceanic climate regimes have therefore generated very different structural and plant functional trait solutions. We argue that the southern conifers have, by virtue of their continuous presence in oceanic environments, arrived at an evolutionary solution that takes advantage of their stress resistant physiology and morphology and minimizes the consequences of the accompanying slow growth. In an oceanic temperate, evergreen forest, supra-canopy space is potentially available, but only to trees with foliage that can endure winter conditions and survive episodic drying, wind, and high insolation in summer. The hydraulic physiology and leaf morphology of the conifers is clearly superior in this zone to that of all but a few angiosperms. The trade-off is the sacrifice of fast-seedling and sapling growth, which they partially offset with specialized juvenile growth forms. Once established, their longevity is the key to their long-term success as they can wait for the rare occasions which favor large-scale regeneration. Disturbance of a size that permits them to regenerate must be (at an evolutionary timescale) as certain as the alternation of seasons, and this is recognized in the conceptual models developed to explain their regeneration strategy (Ogden, 1985; Ogden and Stewart, 1995; Enright et al., 1999).
It is only under certain climatic conditions that the conifers fail to persist. The first is under hyperoceanic situations—such as that of Stewart Island during the early Holocene. Here, we suggest, a fern-rich ground layer, dense broadleaf shrub and canopy with abundant tree ferns can successfully resist conifer invasion. At a small scale, these conditions also prevail in shady gullies on rich soils where only sporadic conifer regeneration is possible (Coomes et al., 2005). The second situation in which the New Zealand conifers are at a disadvantage is in the presence of Nothofagaceae. Libocedrus bidwillii, Phyllocladus alpinus and Halocarpus dominated the subalpine and upper montane zone in the earlier part of the postglacial, but in nearly all locations have since lost this dominance during the Holocene. While the relatively slow spread rates of Nothofagaceae account for some of the early prevalence of conifer-dominated associations in alpine/montane environments, the underlying driver is almost certainly a climatic switch beginning around 9,000 years ago, from long, low insolation summers and mild winters—which favored slow-growing conifers—to short, high insolation summer and colder, longer winters—which favored the fast-growing mycorrhizal Nothofagaceae (Wilmshurst et al., 2002).
Vacant Tree Niches?
It is possible that there is a vacant tree niche in New Zealand currently represented by the winter cold dryland environments of the rain-shadow of the Southern Alps and a vacant conifer niche at tree line. During the full glacial, a very large area of New Zealand had only sparse, scattered forest, and the open, dry eastern lowlands something approaching open herb field with prostrate shrubs. In Northern Hemisphere temperate regions the LGM was characterized by open conifer dominated parklands unless the desert-steppe climates prevailed (McGlone et al., 2012). The current rapid spread of exotic fast-growing mycorrhizal wilding pines (in particular Pinus contorta and P. nigra) into lowland to montane fire-induced grassland/shrublands (Ledgard, 2001) indicates a vacant “conifer” niche in the drylands that is only partly filled by the Myrtaceous Kunzea. Evolution of such a tree type presumably requires a permanent, highly seasonal, winter-cold environment that is absent in the Southern Hemisphere from all but Antarctica. At some tree lines in the drier eastern side of the axial mountain ranges, exotic pines are spreading above the Nothofagaceae tree line and this likewise suggests a tree line “pine/fir/spruce” niche remains unfilled for similar reasons (Cieraad et al., 2014; Tomiolo et al., 2016).
Are Palynology and Ecology Mutually Supportive?
In New Zealand, history cannot be ignored. Besides the alternation of glacial-interglacial cycles, frequent, massive disruption caused by earthquakes, volcanic eruptions, cyclonic storms and the recent imposition of historically unprecedented human-lit fires have left major historical legacies. Therefore, in New Zealand, palaeoecological and neoecological researchers frequently collaborate as our exploration of conifer history shows. Not only that, neoecologists have often taken the lead in study of the past, as exemplified by Peter Wardle with his Quaternary investigations of soil charcoal (Wardle, 2001a), Colin Burrows and the palaeoecology of the South Island mountains (Burrows et al., 1993), Susan Walker, Bill Lee and Geoff Rogers on the history of the drylands of the southern South Island (Walker et al., 2004b), and the ground-breaking neo-palaeoresearch by ecologist John Ogden and colleagues on many aspects of northern plant successions and Quaternary history (for example: Ogden et al., 1992, 1997).
The answer then to the question posed by Bill Harris many years ago is that the unbroken legacy of Lennart von Post and Lucy Cranwell continues in New Zealand, palaeoecology and ecology combining in a productive relationship to document the past, understand the present, and anticipate the future.
Author Contributions
MM co-led the paleoecological section of the review and wrote the first draft; SR and OB undertook analyses of pollen and ecological data, and prepared the figures; SR and GP led the neoecological component of the review; JW organized underpinning pollen databases and co-led the paleoecological component. All authors contributed to draft revisions of the ms.
Conflict of Interest Statement
The authors declare that the research was conducted in the absence of any commercial or financial relationships that could be construed as a potential conflict of interest.
Acknowledgments
This research was supported by SSIF funding for Crown Research Institutes from the New Zealand Ministry of Business, Innovation and Employment's Science and Innovation Group. We thank Rewi Newnham and Marcus Vandergoes for permission to republish their Okarito pollen data and Susan Walker for access to the data Figure 10 and Jamie Wood for drafting Figure 2. We acknowledge the use of data drawn from the Natural Forest plot data collected between January 2002 and March 2007 by the LUCAS programme for the Ministry for the Environment.
References
Alloway, B. V., Stewart, R. B., Neall, V. E., and Vucetich, C. G. (1992). Climate of the Last Glaciation in New Zealand, based on aerosolic quartz influx in an andesitic terrain. Quaternary Res. 38, 170–179. doi: 10.1016/0033-5894(92)90054-M
Augustinus, P., Cochran, U., Kattel, G., D'costa, D., and Shane, P. (2012). Late quaternary paleolimnology of onepoto maar, Auckland, New Zealand: implications for the drivers of regional paleoclimate. Q. Int. 253, 18–31. doi: 10.1016/j.quaint.2011.02.028
Augustinus, P., D'costa, D., Deng, Y. B., Hagg, J., and Shane, P. (2011). A multi-proxy record of changing environments from ca. 30 000 to 9000 cal. a BP: onepoto maar palaeolake, Auckland, New Zealand. J. Quat. Sci. 26, 389–401. doi: 10.1002/jqs.1463
Bannister, P. (2007). A touch of frost? Cold hardiness of plants in the Southern Hemisphere. N. Z. J. Botany 45, 1–33. doi: 10.1080/00288250709509700
Battersby, P. F., Wilmshurst, J. M., Curran, T. J., Mcglone, M. S., and Perry, G. L. W. (2017). Exploring fire adaptation in a land with little fire: serotiny in Leptospermum scoparium (Myrtaceae). J. Biogeogr. 44, 1306–1318. doi: 10.1111/jbi.12950
Bond, W. (1989). The tortoise and the hare: ecology of angiosperm dominance and gymnosperm persistence. Biol. J. Linnean Soc. 36, 227–249. doi: 10.1111/j.1095-8312.1989.tb00492.x
Bray, J. R. (1989). The use of historical vegetation dynamics in interpreting prehistorical vegetation change. J. R. Soc. N. Z. 19, 151–160. doi: 10.1080/03036758.1989.10426444
Brodribb, T. J., and Cochard, H. (2009). Hydraulic failure defines the recovery and point of death in water-stressed conifers. Plant Physiol. 149, 575–584. doi: 10.1104/pp.108.129783
Brodribb, T. J., Pittermann, J., and Coomes, D. A. (2012). Elegance versus speed: examining the competition between conifer and angiosperm trees. Int. J. Plant Sci. 173, 673–694. doi: 10.1086/666005
Burns, B. R., and Leathwick, J. R. (1996). Vegetation-environment relationships at Waipoua Forest, Northland, New Zealand. N. Z. J. Bot. 34, 79–92. doi: 10.1080/0028825X.1996.10412695
Burrows, C. (1965). Some discontinuous distributions of plants within New Zealand and their ecological significance. 11: disjunctions between Otago-Southland and Nelson-Marlborough and related distribution patterns. Tuatara 13, 9–29.
Burrows, C. J. (1996). Germination behaviour of seeds of the New Zealand woody species Ascarina lucida, Coprosma grandifolia, Melicytus lanceolatus, and Solanum laciniatum. N. Z. J. Bot. 34, 509–515. doi: 10.1080/0028825X.1996.10410131
Burrows, C. J., Randall, P., Moar, N. T., and Butterfield, B. G. (1993). Aranuian vegetation history of the Arrowsmith Range, Canterbury, New Zealand. 3. Vegetation changes in the Cameron, upper south Ashburton and Paddle Hill Creek catchments. N. Z. J. Bot. 31, 147–174. doi: 10.1080/0028825X.1993.10419491
Cameron, E. K. (2000). Lucy May Cranwell, M. A., DSc, DSc(Hon), FLS(Lond.), FRSNZ, 1907-2000-Obituary. N. Z. J. Bot. 38, 527–535. doi: 10.1080/0028825X.2000.9512702
Cameron, R. (1954). Mosaic or cyclical regeneration in North Island podocarp forests. N. Z. J. For. 7, 55–64.
Carswell, F. E., Richardson, S. J., Doherty, J. E., Allen, R. B., and Wiser, S. K. (2007). Where do conifers regenerate after selective harvest? A case study from a New Zealand conifer-angio sperm forest. For. Ecol. Manage. 253, 138–147. doi: 10.1016/j.foreco.2007.07.011
Case, B. S., and Duncan, R. P. (2014). A novel framework for disentangling the scale-dependent influences of abiotic factors on alpine treeline position. Ecography 37, 838–851. doi: 10.1111/ecog.00280
Cieraad, E., and McGlone, M. S. (2014). Thermal environment of New Zealand's gradual and abrupt treeline ecotones. N. Z. J. Ecol. 38, 12–25.
Cieraad, E., McGlone, M. S., and Huntley, B. (2014). Southern Hemisphere temperate tree lines are not climatically depressed. J. Biogeogr. 41, 1456–1466. doi: 10.1111/jbi.12308
Cieraad, E., McGlone, M., Barbour, M. M., and Huntley, B. (2012). Seasonal Frost Tolerance of Trees in the New Zealand Treeline Ecotone. Arct. Antarct. Alp. Res. 44, 332–342. doi: 10.1657/1938-4246-44.3.332
Condamine, F. L., Leslie, A. B., and Antonelli, A. (2017). Ancient islands acted as refugia and pumps for conifer diversity. Cladistics 33, 69–92. doi: 10.1111/cla.12155
Coomes, D. A., Allen, R. B., Bentley, W. A., Burrows, L. E., Canham, C. D., Fagan, L., et al. (2005). The hare, the tortoise and the crocodile: the ecology of angiosperm dominance, conifer persistence and fern filtering. J. Ecol. 93, 918–935. doi: 10.1111/j.1365-2745.2005.01012.x
Coomes, D. A., and Bellingham, P. J. (2011). “Temperate and tropical podocarps: how ecologically alike are they?” in Smithsonian Contributions to Botany: Ecology of the Podocarpaceae in Tropical Forests, eds B. L. Turner and L. A. Cernusak (Washington, DC: Smithsonian Institution Scholarly Press), 119–140.
Cranwell, L. M. (1938). Fossil pollens: the key to the vegetation of the past. N. Z. J. Sci. Technol. 19, 628–645.
Cranwell, L. M., and von Post, L. (1936). Post-Pleistocene pollen diagrams from the southern hemisphere. I New Zealand. Geografiska Annaler. 18, 308–347.
Darvill, C. M., Bentley, M. J., Stokes, C. R., and Shulmeister, J. (2016). The timing and cause of glacial advances in the southern mid-latitudes during the last glacial cycle based on a synthesis of exposure ages from Patagonia and New Zealand. Quat. Sci. Rev. 149, 200–214. doi: 10.1016/j.quascirev.2016.07.024
D'Costa, D., Boswijk, G., and Ogden, J. (2009). Holocene vegetation and environmental reconstructions from swamp deposits in the Dargaville region of the North Island, New Zealand: implications for the history of kauri (Agathis australis). Holocene 19, 559–574. doi: 10.1177/0959683609104026
de Jonge, V. N., Pinto, R., and Turner, R. K. (2012). Integrating ecological, economic and social aspects to generate useful management information under the EU Directives' ‘ecosystem approach’. Ocean Coast. Manag. 68, 169–188. doi: 10.1016/j.ocecoaman.2012.05.017
de Lange, P. J. (2014). A revision of the New Zealand Kunzea ericoides (Myrtaceae) complex. PhytoKeys 40, 1–185. doi: 10.3897/phytokeys.40.7973
DellaSala, D. A. (2011). Temperate and Boreal Rainforests of the World: Ecology and Conservation. Washington, DC: Island Press.
Dickie, I. A., Koele, N., Blum, J. D., Gleason, J. D., and McGlone, M. S. (2014). Mycorrhizas in changing ecosystems. Botany 92, 149–160. doi: 10.1139/cjb-2013-0091
Dorken, V. M., and Parsons, R. F. (2016). Morpho-anatomical studies on the change in the foliage of two imbricate-leaved New Zealand podocarps: Dacrycarpus dacrydioides and Dacrydium cupressinum. Plant Syst. Evol. 302, 41–54. doi: 10.1007/s00606-015-1239-5
Ebbett, R., and Ogden, J. (1998). Comparative seedling growth of five endemic New Zealand podocarp species under different light regimes. N. Z. J. Bot. 36, 189–201. doi: 10.1080/0028825X.1998.9638781
Elliot, M. B. (1998). Late Quaternary pollen records of vegetation and climate change from Kaitaia Bog, far northern New Zealand. Rev. Palaeobot. Palynol. 99, 189–202. doi: 10.1016/S0034-6667(97)00048-1
Elliot, M., Neall, V., and Wallace, C. (2005). A Late Quaternary pollen record from Lake Tangonge, far northern New Zealand. Rev. Palaeobot. Palynol. 136, 143–158. doi: 10.1016/j.revpalbo.2005.05.003
Enright, N. J., Ogden, J., and Rigg, L. S. (1999). Dynamics of forests with Araucariaceae in the western Pacific. J. Veget. Sci. 10, 793–804. doi: 10.2307/3237304
Haenfling, C., Newnham, R., Rees, A., Jara, I., Homes, A., and Clarkson, B. (2017). Holocene history of a raised bog, northern New Zealand, based on plant cuticles. Holocene 27, 309–314. doi: 10.1177/0959683616658524
Hall, G. M. J., and McGlone, M. S. (2001). Forest reconstruction and past climatic estimates for a deforested region of south-eastern New Zealand. Landsc. Ecol. 16, 501–521. doi: 10.1023/A:1013199209388
Hall, G. M. J., and McGlone, M. S. (2006). Potential forest cover of New Zealand as determined by an ecosystem process model. N. Z. J. Bot. 44, 211–232. doi: 10.1080/0028825X.2006.9513019
Harris, W. (1963). Evidence for ecologically significant changes in climate during the post-glacial period in New Zealand: paleo-ecological evidence from pollen and spores. Proc. N. Z. Ecol. Soc. 10, 38–44.
Hawkins, B. J., and Sweet, G. B. (1989). Evolutionary interpretation of a high temperature growth response in 5 New Zealand forest tree species. N. Z. J. Bot. 27, 101–107. doi: 10.1080/0028825X.1989.10410148
Heenan, P. B., and McGlone, M. S. (2013). Evolution of New Zealand alpine and open-habitat plant species during the late Cenozoic. N. Z. J. Ecol. 37, 105–113.
Holloway, J. T. (1954). Forests and climate in the South Island of New Zealand. Trans. R. Soc. N. Z. 82, 329–410.
Horrocks, M., and Ogden, J. (1998). The Effects of the Taupo Tephra Eruption of c. 1718 BP on the Vegetation of Mt Hauhungatahi, Central North Island, New Zealand. J. Biogeogr. 25, 649–660. doi: 10.1046/j.1365-2699.1998.2540649.x
Horrocks, M., Deng, Y., Ogden, J., Alloway, B. V., Nichol, S. L., and Sutton, D. G. (2001). High spatial resolution of pollen and charcoal in relation to the c600-year-BP Kaharoa Tephra: implications for Polynesian settlement of Great Barrier Island, Northern New Zealand. J. Archaeol. Sci. 28, 153–168. doi: 10.1006/jasc.2000.0568
Jara, I. A., Newnham, R. M., Vandergoes, M. J., Foster, C. R., Lowe, D. J., Wilmshurst, J. M., et al. (2015). Pollen-climate reconstruction from northern South Island, New Zealand (41 degrees S), reveals varying high- and low-latitude teleconnections over the last 16,000 years. J. Quaternary Sci. 30, 817–829. doi: 10.1002/jqs.2818
Kirkpatrick, J. B., and DellaSala, D. A. (2011). “Temperate rainforests of Australasia,” in Temperate and Boreal Rainforests of the World: Ecology and Conservation, ed D. A. Dellasala (Washington, DC: Island Press), 195–212.
Koch, G. W., Sillett, S. C., Jennings, G. M., and Davis, S. D. (2004). The limits to tree height. Nature 428, 851–854. doi: 10.1038/nature02417
Kooyman, R. M., Wilf, P., Barreda, V. D., Carpenter, R. J., Jordan, G. J., Sniderman, J. M. K., et al. (2014). Paleo-Antarctic rainforest into the modern Old World tropics: the rich past and threatened future of the “southern wet forest survivors.” Am. J. Bot. 101, 2121–2135. doi: 10.3732/ajb.1400340
Kunert, N., Aparecido, L. M. T., Wolff, S., Higuchi, N., Dos Santos, J., De Araujo, A. C., et al. (2017). A revised hydrological model for the Central Amazon: the importance of emergent canopy trees in the forest water budget. Agric. For. Meteorol. 239, 47–57. doi: 10.1016/j.agrformet.2017.03.002
Leathwick, J. R. (1995). Climatic relationships of some New Zealand forest tree species. J. Vegetation Sci. 6, 237–248. doi: 10.2307/3236219
Leathwick, J. R. (2001). New Zealand's potential forest pattern as predicted from current species-environment relationships. N. Z. J. Bot. 39, 447–464. doi: 10.1080/0028825X.2001.9512748
Leathwick, J. R., and Whitehead, D. (2001). Soil and atmospheric water deficits and the distribution of New Zealand's indigenous tree species. Funct. Ecol. 15, 233–242. doi: 10.1046/j.1365-2435.2001.00504.x
Leathwick, J. R., Wilson, G., Rutledge, D., Wardle, P., Morgan, F., Johnston, K., et al. (2003). Land Environments of New Zealand. Auckland: David Bateman.
Ledgard, N. (2001). The spread of lodgepole pine (Pinus contorta, Dougl.) in New Zealand. For. Ecol. Manage. 141, 43–57. doi: 10.1016/S0378-1127(00)00488-6
Li, X., Rapson, G., and Flenley, J. R. (2008). Holocene vegetational and climatic history, Sponge Swamp, Haast, south-western New Zealand. Quaternary Int. 184, 129–138. doi: 10.1016/j.quaint.2007.09.011
Lorrey, A. M., Vandergoes, M., Almond, P., Renwick, J., Stephens, T., Bostock, H., et al. (2012). Palaeocirculation across New Zealand during the last glacial maximum at similar to 21 ka. Quat. Sci. Rev. 36, 189–213. doi: 10.1016/j.quascirev.2011.09.025
Lu, Y., Ran, J. H., Guo, D. M., Yang, Z. Y., and Wang, X. Q. (2014). Phylogeny and divergence times of gymnosperms inferred from single-copy nuclear genes. PLoS ONE 9:e107679. doi: 10.1371/journal.pone.0107679
Lusk, C. H. (2002). Basal area in a New Zealand podocarp-broadleaved forest: are coniferous and angiosperm components independent? N. Z. J. Bot. 40, 143–147. doi: 10.1080/0028825X.2002.9512778
Lusk, C. H., and Smith, B. (1998). Life history differences and tree species coexistence in an old-growth New Zealand rain forest. Ecology 79, 795–806. doi: 10.1890/0012-9658(1998)079[0795:LHDATS]2.0.CO;2
Lusk, C. H., Duncan, R. P., and Bellingham, P. J. (2009). Light environments occupied by conifer and angiosperm seedlings in a New Zealand podocarp-broadleaved forest. N. Z. J. Ecol. 33, 83–89.
Lusk, C. H., Jorgensen, M. A., and Bellingham, P. J. (2015). A conifer-angiosperm divergence in the growth vs. shade tolerance trade-off underlies the dynamics of a New Zealand warm-temperate rain forest. J. Ecol. 103, 479–488. doi: 10.1111/1365-2745.12368
Lusk, C. H., Kaneko, T., Grierson, E., and Clearwater, M. (2013). Correlates of tree species sorting along a temperature gradient in New Zealand rain forests: seedling functional traits, growth and shade tolerance. J. Ecol. 101, 1531–1541. doi: 10.1111/1365-2745.12152
Macinnis-Ng, C., Wyse, S., Veale, A., Schwendenmann, L., and Clearwater, M. (2016). Sap flow of the southern conifer, Agathis australis during wet and dry summers. Trees-Struct. Funct. 30, 19–33. doi: 10.1007/s00468-015-1164-9
Macphail, M. K., and McQueen, D. R. (1983). The value of New Zealand pollen and spores as indicators of Cenozoic vegetation and climates. Tuatara 26, 37–56.
Marra, M., and Leschen, R. A. B. (2004). Late Quaternary paleoecology from fossil beetle communities in the Awatere Valley, South Island, New zealand. J. Biogeogr. 31, 571–586. doi: 10.1046/j.1365-2699.2003.00998.x
Martin, T. J., and Ogden, J. (2005). Experimental studies on the drought, waterlogging, and frost tolerance of Ascarina lucida Hook. f (Chloranthaceae) seedlings. N. Z. J. Ecol. 29, 53–59.
McGlone, M. S. (2001a). A late Quaternary pollen record from marine core P69, southeastern North Island, New Zealand. N. Z. J. Geol. Geophys. 44, 69–77. doi: 10.1080/00288306.2001.9514923
McGlone, M. S. (2001b). The origin of the indigenous grasslands of southeastern South Island in relation to pre-human woody ecosystems. N. Z. J. Ecol. 25, 1–15.
McGlone, M. S. (2002). A Holocene and latest Pleistocene pollen record from Lake Poukawa, Hawke's Bay, New Zealand. Glob. Planet. Change 33, 283–299. doi: 10.1016/S0921-8181(02)00083-8
McGlone, M. S. (2009). Postglacial history of New Zealand wetlands and implications for their conservation. N. Z. J. Ecol. 33, 1–23.
McGlone, M. S., and Basher, L. (2012). “Holocene vegetation change at treeline, Cropp Valley, Southern Alps, New Zealand,” in Peopled Landscapes: Archaeological and Biogeographic Approaches to Landscape, eds S. Haberle and B. David (Canberra, ACT: ANU E Press), 343–358.
McGlone, M. S., and Bathgate, J. L. (1983). Vegetation and climate history of the Longwood Range, South Island, New Zealand, 12 000 B.P. to the present. N. Z. J. Bot. 21, 293–315. doi: 10.1080/0028825X.1983.10428560
McGlone, M. S., and Moar, N. T. (1977). Ascarina decline and postglacial climatic change in New Zealand. N. Z. J. Bot. 15, 485–489. doi: 10.1080/0028825X.1977.10432554
McGlone, M. S., and Moar, N. T. (1998). Dryland Holocene vegetation history, Central Otago and the Mackenzie Basin, South Island, New Zealand. N. Z. J. Bot. 36, 91–111. doi: 10.1080/0028825X.1998.9512549
McGlone, M. S., and Neall, V. E. (1994). The late Pleistocene and Holocene vegetation history of Taranaki, North Island, New Zealand. N. Z. J. Bot. 32, 251–269. doi: 10.1080/0028825X.1994.10410470
McGlone, M. S., and Topping, W. W. (1977). Aranuian (postglacial) pollen diagrams from Tongariro region, North Island, New Zealand. N. Z. J. Bot. 15, 749–760. doi: 10.1080/0028825X.1977.10429643
McGlone, M. S., and Topping, W. W. (1983). Late Quaternary vegetation, Tongariro region, central North Island, New Zealand. N. Z. J. Bot. 21, 53–76. doi: 10.1080/0028825X.1983.10428525
McGlone, M. S., and Wilson, H. D. (1996). Holocene vegetation and climate of Stewart Island, New Zealand. N. Z. J. Bot. 34, 369–388. doi: 10.1080/0028825X.1996.10410701
McGlone, M. S., Buitenwerf, R., and Richardson, S. J. (2016). The formation of the oceanic temperate forests of New Zealand. N. Z. J. Bot. 54, 128–155. doi: 10.1080/0028825X.2016.1158196
McGlone, M. S., Hall, G. M. J., and Wilmshurst, J. M. (2011). Seasonality in the early Holocene: extending fossil-based estimates with a forest ecosystem process model. Holocene 21, 517–526. doi: 10.1177/0959683610385717
McGlone, M. S., Mark, A. F., and Bell, D. (1995). Late Peistocene and Holocene vegetation history, central Otago, South Island, New Zealand. J. R. Soc. N. Z. 25, 1–22. doi: 10.1080/03014223.1995.9517480
McGlone, M. S., Mildenhall, D. C., and Pole, M. S. (1996). “History and palaeoecology of New Zealand Nothofagus forests,” in The Ecology and Biogeography of Nothofagus forest, eds T. T. Veblen, R. S. Hill, and J. Read (New Haven, CT: Yale University Press), 83–130.
McGlone, M. S., Moar, N. T., and Meurk, C. D. (1997). Growth and vegetation history of alpine mires on the Old Man Range, Central Otago, New Zealand. Arc. Alp. Res. 29, 32–44. doi: 10.2307/1551834
McGlone, M. S., Newnham, R. M., and Moar, N. T. (2010). “The vegetation cover of New Zealand during the Last Glacial Maximum: do pollen records under-represent woody vegetation?,” in Altered Fire Ecologies: Fire, Climate and Human Influences on Terrestrial Landscapes, eds S. Haberle, J. Stevenson, and M. Prebble (Canberra, ACT: ANU E Press), 49–68.
McGlone, M. S., Turney, C. S. M., and Wilmshurst, J. M. (2004). Late-glacial and Holocene vegetation and climatic history of the Cass basin, central south island, New Zealand. Quaternary Res. 62, 267–279. doi: 10.1016/j.yqres.2004.09.003
McGlone, M., Wardle, P., and Worthy, T. (2003). “Environmental change since the Last Glaciation,” in The Natural History of Southern New Zealand, eds D. John, R. E. Fordyce, A. Mark, K. Probert and C. Townshend (Dunedin: Otago University Press), 105–128.
McGlone, M., Wood, J., and Bartlein, P. J. (2012). “Environmental Change in the Temperate Forested Regions,” in The Sage Handbook of Environmental Change, eds J. A. Matthews, P. J. Bartlein, K. R. Briffa, A. G. Dawson, A. De Vernal, T. Denham, S. C. Fritz and F. Oldfield (Los Angeles, CA: Sage), 188–214.
McIntosh, P. D., Eden, D. N., and Burgham, S. J. (1990). Quaternary deposits and landscape evolution in northeast Southland, New Zealand. Palaeogeogr. Palaeoclimatol. Palaeoecol. 81, 95–113. doi: 10.1016/0031-0182(90)90042-6
McWethy, D. B., Whitlock, C., Wilmshurst, J. M., McGlone, M. S., Fromont, M., Li, X., et al. (2010). Rapid landscape transformation in South Island, New Zealand, following initial Polynesian settlement. Proc. Natl. Acad. Sci. U.S.A. 107, 21343–21348. doi: 10.1073/pnas.1011801107
Moar, N. (1973). Late Pleistocene vegetation and environment in southern New Zealand. Palaeoecol. Afr. Surround. Isl. Antarct. 8, 179–198.
Moar, N. T. (1971). Contributions to the Quaternary history of the New Zealand flora. 6. Aranuian pollen diagrams from Canterbury, Nelson, and north Westland, South Island. N. Z. J. Bot. 9, 80–145. doi: 10.1080/0028825X.1971.10430172
Moar, N. T. (1980). Late Otiran and early Aranuian grassland in central South Island. N. Z. J. Ecol. 3, 4–12.
Molloy, B. P. J. (1968). “Recent history of the vegetation,” in The Natural History of Canterbury, ed G. A. Knox (Wellington: A.H. & A.W. Reed), 340–360.
Molloy, B. P., Burrows, C., Cox, J., Johnston, J., and Wardle, P. (1963). Distribution of subfossil forest remains, eastern South Island, New Zealand. N. Z. J. Bot. 1, 68–77. doi: 10.1080/0028825X.1963.10429322
Moreno, P. I., Denton, G. H., Moreno, H., Lowell, T. V., Putnam, A. E., and Kaplan, M. R. (2015). Radiocarbon chronology of the last glacial maximum and its termination in northwestern Patagonia. Quat. Sci. Rev. 122, 233–249. doi: 10.1016/j.quascirev.2015.05.027
Newnham, R. (1999). Environmental change in Northland, New Zealand during the last glacial and Holocene. Quaternary Int. 57–58, 61–70. doi: 10.1016/S1040-6182(98)00050-0
Newnham, R. M. (1992). A 30,000 year pollen, vegetation and climate record from Otakairangi (Hikurangi), Northland, New Zealand. J. Biogeogr. 19, 541–554. doi: 10.2307/2845773
Newnham, R. M., Alloway, B. V., Holt, K. A., Butler, K., Rees, A. B. H., Wilmshurst, J. M., et al. (2017). Last Glacial pollen-climate reconstructions from Northland, New Zealand. J. Quaternary Sci. 32, 685–703. doi: 10.1002/jqs.2955
Newnham, R. M., and Lowe, D. J. (2000). Fine-resolution pollen record of late-glacial climate reversal from New Zealand. Geology 28, 759–762. doi: 10.1130/0091-7613(2000)28<759:FPROLC>2.0.CO;2
Newnham, R. M., Lowe, D. J., and Green, J. D. (1989). Palynology, vegetation and climate of the Waikato lowlands, North Island, New Zealand, since c. 18,000 years ago. J. R. Soc. N. Z. 19, 127–150. doi: 10.1080/03036758.1989.10426443
Newnham, R. M., Lowe, D. J., and Williams, P. W. (1999). Quaternary environmental change in New Zealand: a review. Prog. Phys. Geogr. 23, 567–610. doi: 10.1177/030913339902300406
Newnham, R. M., Vandergoes, M. J., Hendy, C. H., Lowe, D. J., and Preusser, F. (2007). A terrestrial palynological record for the last two glacial cycles from southwestern New Zealand. Quat. Sci. Rev. 26, 517–535. doi: 10.1016/j.quascirev.2006.05.005
Newnham, R., McGlone, M., Moar, N., Wilmshurst, J., and Vandergoes, M. (2013). The vegetation cover of New Zealand at the Last Glacial Maximum. Quat. Sci. Rev. 74, 202–214. doi: 10.1016/j.quascirev.2012.08.022
Nicholls, J. (1956). The historical ecology of the indigenous forest of the Taranaki upland. N. Z. J. For. 7, 17–34.
Ogden, J. (1985). An introduction to plant demography with special reference to New Zealand trees. N. Z. J. Bot. 23, 751–772. doi: 10.1080/0028825X.1985.10434241
Ogden, J., and Stewart, G. H. (1995). “Community dynamics of the New Zealand conifers,” in Ecology of the Southern Conifers, eds N. J. Enright and R. S. Hill (Melbourne, VIC: Melbourne University Press), 81–119.
Ogden, J., Basher, L., and McGlone, M. (1998). Fire, forest regeneration and links with early human habitation: evidence from New Zealand. Ann. Bot. 81, 687–696. doi: 10.1006/anbo.1998.0637
Ogden, J., Horrocks, M., Palmer, J. G., and Fordham, R. A. (1997). Structure and composition of the subalpine forest on Mount Hauhungatahi North Island, New Zealand, during the Holocene. Holocene 7, 13–23. doi: 10.1177/095968369700700102
Ogden, J., Wilson, A., Hendy, C., Newnham, R. M., and Hogg, A. G. (1992). The late Quaternary history of kauri (Agathis australis) in New Zealand and its climatic significance. J. Biogeogr. 19, 611–622. doi: 10.2307/2845704
Perry, G. L. W., Wilmshurst, J. M., and McGlone, M. S. (2014). Ecology and long-term history of fire in New Zealand. N. Z. J. Ecol. 38, 157–176.
Perry, G. L. W., Wilmshurst, J. M., McGlone, M. S., Mcwethy, D. B., and Whitlock, C. (2012a). Explaining fire-driven landscape transformation during the initial burning period of New Zealand's prehistory. Glob. Chang. Biol. 18, 1609–1621. doi: 10.1111/j.1365-2486.2011.02631.x
Perry, G. L. W., Wilmshurst, J. M., McGlone, M. S., and Napier, A. (2012b). Reconstructing spatial vulnerability to forest loss by fire in pre-historic New Zealand. Glob. Ecol. Biogeogr. 21, 1029–1041. doi: 10.1111/j.1466-8238.2011.00745.x
Pickrill, R. A., Fenner, J. M., and McGlone, M. S. (1992). Late Quaternary evolution of a fjord environment in Preservation Inlet, New Zealand. Quaternary Res. 38, 331–346. doi: 10.1016/0033-5894(92)90042-H
Pittermann, J., Stuart, S. A., Dawson, T. E., and Moreau, A. (2012). Cenozoic climate change shaped the evolutionary ecophysiology of the Cupressaceae conifers. Proc. Natl. Acad. Sci. U.S.A. 109, 9647–9652. doi: 10.1073/pnas.1114378109
Pocknall, D. T. (1980). Modern pollen rain and Aranuian vegetation from Lady Lake, North Westland, New Zealand. N. Z. J. Bot. 18, 275–284. doi: 10.1080/0028825X.1980.10426925
Pugh, J., and Shulmeister, J. (2010). “Holocene vegetation history of a high-elevation (1200 m) site in the Lake Heron Basin, inland Canterbury, New Zealand,” in Altered Ecologies: Fire, Climate and Human Influence on Terrestrial Landscapes. Terra Australis, Vol. 32, eds S. Haberle, J. Stevenson, and M. Prebble (Canberra, ACT: ANU E Press), 69–81.
Reitalu, T., Kuneš, P., and Giesecke, T. (2014). Closing the gap between plant ecology and Quaternary palaeoecology. J. Veget. Sci. 25, 1188–1194. doi: 10.1111/jvs.12187
Richardson, S. J., Allen, R. B., Whitehead, D., Carswell, F. E., Ruscoe, W. A., and Platt, K. H. (2005a). Climate and net carbon availability determine temporal patterns of seed production by Nothofagus. Ecology 86, 972–981. doi: 10.1890/04-0863
Richardson, S. J., Hurst, J. M., Easdale, T. A., Wiser, S. K., Griffiths, A. D., and Allen, R. B. (2011). Diameter growth rates of beech (Nothofagus) trees around New Zealand. N. Z. J. For. 56, 3–11.
Richardson, S. J., Peltzer, D. A., Allen, R. B., and McGlone, M. S. (2005b). Resorption proficiency along a chronosequence: responses among communities and within species. Ecology 86, 20–25. doi: 10.1890/04-0524
Richardson, S. J., Smale, M. C., Hurst, J. M., Fitzgerald, N. B., Peltzer, D. A., Allen, R. B., et al. (2009). Large-tree growth and mortality rates in forests of the central North Island, New Zealand. N. Z. J. Ecol. 33, 208–215.
Robbins, R. G. (1962). The podocarp-broadleaf forests of New Zealand. Trans. R. Soc. N. Z. Bot. 1, 33–75.
Rogers, G. M., and McGlone, M. S. (1989). A postglacial vegetation history of the southern-central uplands of North Island, New Zealand. J. R. Soc. N. Z. 19, 229–248. doi: 10.1080/03036758.1989.10427179
Rogers, G. M., and McGlone, M. S. (1994). A history of Kaiparoro clearing and the limits of Nothofagus in the northern Tararua Range, New Zealand. N. Z. J. Bot. 32, 463–482. doi: 10.1080/0028825X.1994.10412933
Rogers, G. M., Walker, S., and Lee, W. G. (2005). The Role of Disturbance in Dryland New Zealand: Past and Present. Department of Conservation, Wellington.
Rull, V. (2010). Ecology and palaeoecology: two approaches, one objective. Open Ecol. J. 3, 1–5. doi: 10.2174/1874213001003020001
Sandiford, A., Horrocks, M., Newnham, R., Ogden, J., and Alloway, B. (2002). Environmental change during the last glacial maximum (c. 25 000-c. 16 500 years BP) at Mt Richmond, Auckland Isthmus, New Zealand. J. R. Soc. N. Z. 32, 155–167. doi: 10.1080/03014223.2002.9517688
Sandiford, A., Newnham, R., Alloway, B., and Ogden, J. (2003). A 28 000-7600 cal yr BP pollen record of vegetation and climate change from Pukaki Crater, northern New Zealand. Palaeogeogr. Palaeoclimatol. Palaeoecol. 201, 235–247. doi: 10.1016/S0031-0182(03)00611-4
Shulmeister, J., Goodwin, I., Renwick, J., Harle, K., Armand, L., McGlone, M. S., et al. (2004). The Southern hemisphere westerlies in the Australasian sector over the last glacial cycle: a synthesis. Quaternary Int. 118, 23–53. doi: 10.1016/S1040-6182(03)00129-0
Smale, M. C., Coomes, D. A., Parfitt, R. L., Peltzer, D. A., Mason, N. W. H., and Fitzgerald, N. B. (2016). Post-volcanic forest succession on New Zealand's North Island: an appraisal from long-term plot data. N. Z. J. Bot. 54, 11–29. doi: 10.1080/0028825X.2015.1102747
Sperry, J. S., Hacke, U. G., and Pittermann, J. (2006). Size and function in conifer tracheids and angiosperm vessels. Am. J. Bot. 93, 1490–1500. doi: 10.3732/ajb.93.10.1490
Steward, G. A., and Beveridge, A. E. (2010). A review of New Zealand kauri (Agathis australis (D. Don) Lindl.): its ecology, history, growth and potential for management for timber. N. Z. J. For. Sci. 40, 33–59.
Tomiolo, S., Harsch, M. A., Duncan, R. P., and Hulme, P. E. (2016). Influence of climate and regeneration microsites on Pinus contorta invasion into an alpine ecosystem in New Zealand. Aims Environ. Sci. 3, 525–540. doi: 10.3934/environsci.2016.3.525
Turney, C., Wilmshurst, J., Jones, R., Wood, J., Palmer, J., Hogg, A., et al. (2017). Reconstructing atmospheric circulation over southern New Zealand: establishment of modern westerly airflow 5,500 years ago and implications for Southern Hemisphere Holocene climate change. Quat. Sci. Rev. 159, 77–87. doi: 10.1016/j.quascirev.2016.12.017
Vandergoes, M. J., Fitzsimons, S. J., and Newnham, R. M. (1997). Late glacial to Holocene vegetation and climate change in the eastern Takitimu Mountains, western Southland, New Zealand. J. R. Soc. N. Z. 27, 53–66. doi: 10.1080/03014223.1997.9517527
Vandergoes, M. J., Newnham, R. M., Preusser, F., Hendy, C. H., Lowell, T. V., Fitzsimons, S. J., et al. (2005). Regional insolation forcing of late Quaternary climate change in the Southern Hemisphere. Nature 436, 242–245. doi: 10.1038/nature03826
Veblen, T. T., and Stewart, G. H. (1982). On the conifer regeneration gap in New Zealand-the dynamics of Libocedrus bidwillii stands on South Island. J. Ecol. 70, 413–436.
Walker, S., King, N., Monks, A., Williams, S., Burrows, L., Cieraad, E., et al. (2009). Secondary woody vegetation patterns in New Zealand's South Island dryland zone. N. Z. J. Bot. 47, 367–393. doi: 10.1080/0028825x.2009.9672713
Walker, S., Lee, W. G., and Rogers, G. M. (2004a). Pre-settlement woody vegetation of Central Otago, New Zealand. N. Z. J. Bot. 42, 613–646. doi: 10.1080/0028825X.2004.9512915
Walker, S., Lee, W. G., and Rogers, G. M. (2004b). The woody vegetation of Central Otago, New Zealand. N. Z. J. Bot. 42, 589–612. doi: 10.1080/0028825X.2004.9512914
Wardle, J. A. (1984). The New Zealand Beeches: Ecology, Utilization and Management. Wellington: New Zealand Forest Service.
Wardle, J. A. (2011). Wardle's Native Trees of New Zealand and Their Story. Wellington: New Zealand Farm Forestry Association.
Wardle, P. (1963). The Regeneration gap of New Zealand gymnosperms. N. Z. J. Bot. 1, 301–315. doi: 10.1080/0028825X.1963.10429001
Wardle, P. (1985). New Zealand timberlines. 2. A study of forest limits in the Crow Valley near Arthur's Pass, Canterbury. N. Z. J. Bot. 23, 235–261. doi: 10.1080/0028825X.1985.10425329
Wardle, P. (2001a). Distribution of native forest in the upper Clutha district, Otago, New Zealand. N. Z. J. Bot. 39, 435–446. doi: 10.1080/0028825X.2001.9512747
Wardle, P. (2001b). Holocene forest fires in the upper Clutha district, Otago, New Zealand. N. Z. J. Bot. 39, 523–542. doi: 10.1080/0028825X.2001.9512755
Wardle, P., and Lee, W. G. (1990). Environmental and floristic gradients in Westland, New Zealand, and the discontinuous distribution of Nothofagus-comment. N. Z. J. Bot. 28, 479–481. doi: 10.1080/0028825X.1990.10412333
Whitehead, D., Barbour, M. M., Griffin, K. L., Turnbull, M. H., and Tissue, D. T. (2011). Effects of leaf age and tree size on stomatal and mesophyll limitations to photosynthesis in mountain beech (Nothofagus solandrii var. cliffortiodes). Tree Physiol. 31, 985–996. doi: 10.1093/treephys/tpr021
Willett, R. (1950). The New Zealand Pleistocene snow line, climatic conditions, and suggested biological effects. N. Z. J. Sci. Technol. 32, 18–48.
Wilmshurst, J. M., and McGlone, M. S. (1996). Forest disturbance in the central North Island, New Zealand, following the 1850 BP Taupo eruption. Holocene 6, 399–411. doi: 10.1177/095968369600600402
Wilmshurst, J. M., McGlone, M. S., and Charman, D. J. (2002). Holocene vegetation and climate change in southern New Zealand: linkages between forest composition and quantitative surface moisture reconstructions from an ombrogenous bog. J. Quaternary Sci. 17, 653–666. doi: 10.1002/jqs.689
Wilmshurst, J. M., Moar, N. T., Wood, J. R., Bellingham, P. J., Findlater, A. M., Robinson, J. J., et al. (2014). Use of Pollen and Ancient DNA as Conservation Baselines for Offshore Islands in New Zealand. Conserv. Biol. 28, 202–212. doi: 10.1111/cobi.12150
Woodward, C., Shulmeister, J., Zawadzki, A., and Jacobsen, G. (2014). Major disturbance to aquatic ecosystems in the South Island, New Zealand, following human settlement in the Late Holocene. Holocene 24, 668–678. doi: 10.1177/0959683614526935
Wright, I. C., McGlone, M. S., Nelson, C. S., and Pillans, B. J. (1995). An integrated latest Quaternary (Stage 3 to present) paleoclimatic and paleoceanographic record from offshore northern New Zealand. Quaternary Res. 44, 283–293. doi: 10.1006/qres.1995.1073
Keywords: conifer, history, New Zealand, glaciation, palynology, Holocene, ecology, niche
Citation: McGlone MS, Richardson SJ, Burge OR, Perry GLW and Wilmshurst JM (2017) Palynology and the Ecology of the New Zealand Conifers. Front. Earth Sci. 5:94. doi: 10.3389/feart.2017.00094
Received: 03 September 2017; Accepted: 03 November 2017;
Published: 16 November 2017.
Edited by:
Jesse L. Morris, University of Utah, United StatesReviewed by:
Li Wu, Anhui Normal University, ChinaThomas A. Minckley, University of Wyoming, United States
Copyright © 2017 McGlone, Richardson, Burge, Perry and Wilmshurst. This is an open-access article distributed under the terms of the Creative Commons Attribution License (CC BY). The use, distribution or reproduction in other forums is permitted, provided the original author(s) or licensor are credited and that the original publication in this journal is cited, in accordance with accepted academic practice. No use, distribution or reproduction is permitted which does not comply with these terms.
*Correspondence: Matt S. McGlone, mcglonem@landcareresearch.co.nz