Behavioral and Developmental Changes in Brown Trout After Exposure to the Antidepressant Venlafaxine
- 1Animal Physiological Ecology, University of Tübingen, Tübingen, Germany
- 2Effect-based Environmental Analysis, University of Tübingen, Tübingen, Germany
- 3Environmental Analytical Chemistry, University of Tübingen, Tübingen, Germany
- 4Steinbeis Transfer Center for Ecotoxicology and Ecophysiology, Rottenburg, Germany
During the last decades, depression has been diagnosed in increasing numbers, accompanied by rising prescription rates of antidepressants. Concomitantly, these pharmaceuticals are frequently detected in surface waters. Serotonin and noradrenalin reuptake inhibitors such as venlafaxine form the second largest group of antidepressants worldwide, and venlafaxine is the second most prescribed antidepressant in Germany. As drug targets are evolutionary highly conserved, venlafaxine can potentially change not only behavior and related physiological processes in humans but also in non-target species, especially aquatic organisms. In order to test this hypothesis for fish, we exposed brown trout larvae and juveniles to venlafaxine at concentrations ranging from 1 to 1,000 μg/L. Larvae were exposed for 5 months from the eyed ova stage until 8 weeks post yolk-sac consumption at 7 and 11 °C. Juveniles were exposed for 4 weeks at 7 °C. Mortality, weight, length, behavior during exposure and behavior in a stressful environment were recorded in both experiments. For larvae, additionally, hatching rate and heart rate were analyzed. In juvenile fish, tissue cortisol levels were determined. Our results clearly showed, that brown trout, irrespective of their life stage, change their behavior when being exposed to venlafaxine: During exposure, venlafaxine at 7 °C caused larvae to sojourn in the upper part of the aquaria for a longer time, with a lowest observed effect concentration of 100 μg/L. In a stressful environment with limited space, fish exposed to ≥10 μg/L venlafaxine were less active than controls. Furthermore, venlafaxine reduced the growth of larvae (length at ≥10 μg/L, weight at 1 mg/L) and their survival after 5 months (at 1 mg/L). Hatching rate and heart rate of larvae as well as tissue cortisol concentration of juveniles were not affected by venlafaxine treatment.
Introduction
The rising number of diagnosed depression in humans has led to continuously soaring prescription rates of antidepressant drugs during the last decades (Wittchen et al., 2011; Liu et al., 2020). Many of these pharmaceuticals resemble each other with respect to their mode of action: they inhibit the re-uptake of neurotransmitters such as serotonin, noradrenalin or dopamine into the pre-synapse. One of the most prescribed antidepressants worldwide is venlafaxine, a serotonin and noradrenalin reuptake inhibitor (SNRI). To a minor extent, it also inhibits the reuptake of dopamine (Burnett and Dinan, 1998). Since no notable effects of venlafaxine on other receptors were found, e.g., cholinergic, adrenergic and histaminergic receptors, it is regarded a rather specific anti-depressant (Burnett and Dinan, 1998). Venlafaxine is used as therapeutic drug against major depression but also generalized anxiety disorders and panic disorders (HEXAL, 2019).
In Germany, about 200 million defined daily doses (DDD) of venlafaxine were prescribed in 2018. This resulted in a market share of about 13.6% of all prescribed antidepressants (Schwabe et al., 2019). With an assumed defined daily dose (DDD) of 100 mg per patient (WHO, 2019), this leads to a minimum consumption rate of 20 t/year for Germany only. Due to increasing and still rising prescription rates of antidepressants, especially of SNRI during the last decade (Schwabe et al., 2019), and low elimination rates in wastewater treatment plants (Mackul'ak et al., 2015), venlafaxine was detected frequently in the water cycle (Ferrer and Thurman, 2012; Acuña et al., 2015; Schlüsener et al., 2015). Maximum concentrations reached up to 690 ng/L in wastewater-dominated streams, however, mean concentrations in surface waters were about 200 ng/L (Himmelsbach et al., 2006; Lajeunesse et al., 2008; Schultz et al., 2010; Ferrer and Thurman, 2012; Grabicova et al., 2017). In wastewater effluents, venlafaxine was detected in concentrations from 63.8 ng/L up to 2.5 μg/L (Lajeunesse et al., 2012; Schlüsener et al., 2015; Paíga et al., 2016). Its active metabolite, O-desmethylvenlafaxine, was found at two to three times higher concentrations ranging from 610 ng/L up to 4 μg/L in wastewater effluents (Lajeunesse et al., 2012; Schlüsener et al., 2015). Having in mind the continuously increasing prescription rates and the poor elimination rates, surface water concentrations of venlafaxine can be expected to rise in the future (Mackul'ak et al., 2015; Schwabe et al., 2019).
Most studies addressing the risk related to the exposure of aquatic organisms to antidepressants were conducted with selective serotonin reuptake inhibitors (SSRIs), especially with fluoxetine (Henry and Black, 2008; Mennigen et al., 2010; Martin et al., 2017). Less information is available for SNRI impact on fish, but, nevertheless, a number of studies were conducted in fathead minnow (Pimephales promelas) with a focus on venlafaxine impact on behavior. For this species, diminished escape behavior, increased mortality, increased nest defense and egg production were reported (Painter et al., 2009; Parrott and Metcalfe, 2017;, 2018; Schultz et al., 2010). Decreased hatching rates, deformities in fry and increased weight of males were shown in fathead minnows exposed to a mixture of the antidepressants venlafaxine (24 μg/L), citalopram (2.4 μg/L), fluoxetine (900 ng/L), sertraline (200 ng/L) and bupropion (900 ng/L). However, these effects could not be attributed to a single antidepressant (Parrott and Metcalfe, 2019). In white seabream (Diplodus sargus), an exposure of larvae to venlafaxine resulted in decreased survival, increased attack frequency and time on nauplii prey (Rodrigues et al., 2019). Marine juvenile meagre (Argyrosomus regios) exposed to 20 μg/L venlafaxine showed an increased sojourn time in the top part of the water column and a decreased activity in the novel tank diving test (Maulvault et al., 2018). Melvin (2017) showed a change in the circadian rhythm of male mosquitofish (Gambusia holbrooki) when exposed to at least 100 μg/L of the antidepressant. Also, in invertebrates, venlafaxine was shown to modulate behavior. In several studies, foot detachment from the surface and increased moving velocity were reported for snails (Fong and Hoy, 2012; Fong and Molnar, 2013; Fong et al., 2015). In common cuttlefish (Sepia officinalis) brain regional changes in cellular proliferation, a decrease in the norepinephrine level and a reduction of the relative number of glutamate N-methyl-D-aspartate (NMDA)-like receptor binding sites in response to 5 ng/L venlafaxine after 20 days exposure were reported. Additionally, 100 ng/L venlafaxine affected the camouflage ability of these animals (Bidel et al., 2016).
Up to now, there is still a lack of knowledge concerning the risk posed by antidepressants to fish native in Central European waters. We therefore conducted our study with two life stages of brown trout, a species which is supposed to react in a sensitive way to environmental chemicals (Schwarz et al., 2017). Brown trout eggs were exposed to venlafaxine concentrations ranging from environmentally relevant to explicitly higher concentrations (1, 10, 100, 1,000 μg/L) for up to 5 months. However, the highest concentration used is still far below LC50 values of >100 mg/L known for rainbow trout (Pfizer, 2011; Merck, 2017). During exposure, developmental parameters like hatching rate, malformations, heart rate and mortality were recorded. At the end of the experiment, also, weight and length of the fish were assessed. Since venlafaxine as an antidepressant could affect normal and anxiety related behavior, we assessed behavior under conditions of exposure and in a stressful environment. In the comparable novel tank diving test with zebrafish stay in the upper part is connected to reduced anxiety (Maximino et al., 2010a; Stewart et al., 2012) and this behavior could already be detected in fish exposed to venlafaxine (Maulvault et al., 2018). Furthermore, in artificial systems a reduced activity due to venlafaxine exposure could already be detected (Maulvault et al., 2018; Sehonova et al., 2019; Thompson and Vijayan, 2020). Therefore, an increased stay in the upper part as well as reduced activity in a stressful environment could be expected. In a second experiment, juvenile brown trout were exposed to the same venlafaxine concentrations for four weeks and, also here, the apical endpoints mortality, weight and length were determined at the end of the exposure time. Behavior was recorded in a similar way as for brown trout larvae, i.e. during exposure and at the end of the experiment in a stressful environment. As a proxy for their stress status, cortisol levels were determined in juvenile fish.
The aim of our study was to provide data for an environmental risk assessment of venlafaxine with particular emphasis on sublethal effects of this antidepressant, ranging from biochemical to behavioral endpoints, in a feral fish species.
Material and Methods
Fish
Brown trout eggs and juvenile fish were obtained from a commercial trout farm (Forellenzucht Lohmühle, Alpirsbach-Ehlenbogen, Germany). This commercial trout breeder is listed as disease-free Category I according to the EU council directive 2006 (EU, 2006). The eggs in the eyed ova stage were directly transferred into the test aquaria. Juvenile fish were kept in an acclimation tank for one week prior to the experiment and subsequently subjected to experimental exposure. The larvae were kept in climate chambers at 10:14 h light:dark cycle at either 7 °C or 11 °C. Exposure of juveniles was conducted at 7 °C only.
Test Substance
Venlafaxine is an antidepressant belonging to the group of SNRIs (serotonin and noradrenalin reuptake inhibitors). For our experiments we used venlafaxine hydrochloride (C17H27NO2 · HCl, CAS: 99,300-78-4) from Sigma Aldrich (Steinheim, Germany). The stock solutions contained 1 and 100 mg/L venlafaxine dissolved in distilled water. The nominal concentrations of venlafaxine in the experiments refer to the free base (C17H27NO2) of the pharmaceutical.
Experiment With brown Trout Eggs and Hatched Larvae
Brown trout eggs (3 × 10 per treatment) were exposed in a randomized semi-static three-block design to nominal 0, 1, 10, 100 and 1,000 μg/L venlafaxine in 25 L aquaria containing 10 L of the respective test solution. Prior to the exposure of eggs, each aquarium was saturated for seven days with the respective test solution. For each treatment, eggs in the eyed ova stage (32 days post fertilization) were exposed either at 7 °C or at 11 °C in a 10:14 h light:dark cycle in two climate chambers. The aquaria were covered with non-transparent black plastic foil to prevent direct illumination. Twice a week, in each aquarium, 50% of the test solution volume was renewed for sanitary reasons and in order to maintain the respective venlafaxine concentrations. After yolk sac consumption, fish were fed daily with a defined amount of trout feed (first 0.5 mm, later 0.8 mm in diameter, Inico Plus, Biomar, Brande, Denmark) equivalent to 3% of their body weight. During exposure, mortality and hatching rate were recorded on a daily basis. The heart rate of five individuals per treatment was recorded one week after hatching in the respective climate chamber. For heart rate determination, larvae were transferred into a Petri dish. After acclimatization for 5 min, the heart beat was counted for 20 s as a basis to calculate the heart rate (beats per minute). Simultaneously, the water temperature in the Petri dish was measured. Subsequent to this measurement, fish were transferred back into their exposure tank. From six days after yolk-sac consumption until the end of the experiment, three photos of each aquarium were taken daily with the aim to evaluate swimming behavior during exposure. One week before termination of exposure, five individuals of each tank were transferred into an artificial swimming measurement device (described below) aiming at quantifying swimming behavior under stressful conditions. Subsequently, these five fish were anesthetized with an overdose of MS222 (1 g/L buffered with NaHCO3) followed by a cervical spine cut to assure death. For each individual, weight and length were determined. Seven weeks after yolk-sac consumption, when the exposure ended, all remaining fish were killed as described before. Samples of the posterior body part were frozen in liquid nitrogen and stored at −80 °C for further analysis of venlafaxine tissue concentrations. At the beginning and the end of the experiment physico-chemical water parameters (temperature, oxygen concentration, pH, conductivity) were recorded. Additionally, the concentration of nitrite in water was measured at the end of the experiment and four weeks before. Detailed information is given in the Supplementary Material.
Experiment With Juvenile brown Trout
Juvenile brown trout were exposed to venlafaxine at 7 °C with a light:dark cycle of 10:14 h for 25 days. The 25 L tanks were arranged in randomized order following a semi-static three block design. Each aquarium contained 15 L of the respective test solution (0, 1, 10, 100 and 1,000 μg/L Venlafaxine). The top of the tanks were covered with non-transparent black plastic foil to prevent direct illumination. Twice a week, 50% of the test solution was replaced by freshly prepared test solution. Additionally, three tanks with solely 100 μg/L venlafaxine without fish served as a control for possible venlafaxine degradation and were treated equally to the other aquaria. Fish were fed daily with a defined amount of trout feed, equivalent to 3% of their body weight (grain size: 0.8 mm, Inico Plus, Biomar, Brande, Denmark). During the experiment, mortality was recorded daily. Additionally, three photos were taken daily from each tank as a basis to quantify behavior during exposure. At the end of the experiment, all fish were killed as described above except for three animals per aquarium which were used for behavioral analyses under stressful conditions prior to dissection. From each fish, samples were taken from the dorsal body portion, containing muscle and kidney tissue, to determine the cortisol level. Samples of the posterior body portion, containing mainly muscle tissue, were used for the chemical analysis of venlafaxine tissue residues. All samples were frozen in liquid nitrogen immediately after dissection and stored at −80 °C until further processing. At the beginning and the end of the experiments physico-chemical water parameters (temperature, oxygen concentration, pH, conductivity) were recorded. Detailed information is given in the Supplementary Material.
Venlafaxine Analysis
Water Samples
Water samples were taken at the beginning and the end of the experiment and once a month during the experiments. Venlafaxine concentrations were determined using LC-MS with a 1290 Infinity HPLC system (Agilent Technologies, Waldbronn, Germany) and a triple quadrupole mass spectrometer (6490 iFunnel Triple Quadrupole LC/MS, Agilent Technologies) in ESI(+) mode. An Agilent Poroshell-120-EC-C18 (2.7 µm, 2.1 × 100 mm) column at a flow rate of 0.4 ml/min was used for separation, and column temperature was maintained at 40 °C. Eluent A and B were water (+0.1% formic acid) and acetonitrile (+0.1% formic acid), respectively. For the samples taken from the experiment with juvenile brown trout, gradient elution was adapted as follows: 0–1 min 5% B, linear increase to 100% B within 7 min, hold for 7 min at 100% B. After switching back to the starting conditions, a reconditioning time of 3 min was set. Samples were kept in the autosampler at 10 °C and an injection volume of one or 10 µL (dilution factor 0–100). The detection limit of venlafaxine (mass transition m/z 278.2 → 260.2) for undiluted samples was 10 ng/L (10 µL injection volume). For the samples taken from the experiment with brown trout eggs and larvae, the gradient elution was adapted as follows: 0–1 min 5% B, linear increase to 100% B within 5 min, remain at 100% for 2 min, then return to starting conditions for 5 min to recondition the column. Samples were kept in an autosampler at 10 °C, and the injection volume was 5 µL. Further details on operating parameters of the triple quadrupole are provided in the Supplementary Material.
Tissue Samples
Venlafaxine tissue concentrations in brown trout larvae and juveniles were determined by liquid chromatography-mass spectrometry (LC-MS). Sample extraction was carried out with a miniaturized and optimized QuEChERS dispersive solid phase extraction method. Samples of each exposure group were either pooled if the sample quantity was too little (5 animals per pool) or treated individually. Since tailfin samples were expected to contain similar matrices (mainly muscle tissue), all samples were treated in the same way during extraction. The extraction method presented by Ziegler et al. (2020b) originally developed for citalopram, was adapted.
Homogenization of the frozen individual and pooled fish samples (−20 °C) was performed with mortar and pestle under liquid nitrogen. The homogenized samples were transferred into 2.0 ml tubes, weighed (Eppendorf, Hamburg, Germany) and subsequently spiked with deuterated venlafaxine-D6 (Merck KGaA, Darmstadt, Germany). Due to varying venlafaxine tissue concentrations and the consequent different dilution necessary after extraction, samples were spiked with adjusted concentrations. This ensured final concentrations in the established calibration range. Therefore, 10 µL of either a 10 mg/L venlafaxine-D6 solution in methanol for high exposure groups (100 μg/L, 1,000 μg/L venlafaxine in aquarium water) or a 1 mg/L solution for low exposure groups (0 μg/L, 1 μg/L, 10 μg/L) was used. Then, the solvent was evaporated at room temperature for 5 min under a gentle stream of nitrogen. In a next step, 0.25 ml acetonitrile (LC-MS grade, Merck KGaA, Darmstadt, Germany) and 0.75 ml water (LC-MS grade, Merck KGaA, Darmstadt, Germany) were added to the samples followed by mixing for 30 s. Then, 120 mg anhydrous magnesium sulfate (Sigma-Aldrich, Steinheim, Germany), which was dried overnight at 140 °C, and 30 mg sodium chloride (Sigma-Aldrich, Steinheim, Germany) were added to the samples which were immediately mixed for 30 s. After centrifugation for 15 min at 13,000 rpm, 0.1 ml of the acetonitrile phase were separated and evaporated carefully under a continuous stream of nitrogen. The dried residue was resolved in 0.5 ml methanol (LC-MS grade, Chemsolute, Renningen, Germany) and diluted to concentrations in the established calibration range for venlafaxine and venlafaxine-D6. At last, the methanol extract was filtered for LC-MS analysis with a 45 µm PTFE filter (pore size: 0.45 µm, Chromafil, Macherey-Nagel, Düren, Germany). Matrix-matched calibration was performed between 1 and 50 μg/L with venlafaxine-D6 and venlafaxine (>98%, Merck KGaA, Darmstadt, Germany). The limit of detection of the method was 5 ng/L. Recoveries of the extraction process were calculated by using standard addition after extraction. The extraction recovery of venlafaxine-D6 was 20%.
All analyses were performed using a 1,260 Infinity LC system coupled to a 6,550 iFunnel QTOF mass spectrometer (Agilent Technologies, Waldbronn, Germany and Santa Clara, CA, USA) with a jet stream electrospray ionization source. Aliquot samples with a volume of 10 µL were injected onto a Zorbax Eclipse Plus C18 column (2.1 × 150 mm; 3.5 µm particle size, narrow bore, Agilent Technologies, Waldbronn, Germany) at a column temperature of 40 °C. Details for analyses are summarized in the Supplementary Material. A gradient elution was performed with 0.3 ml/min using water and methanol, both containing 0.1% formic acid. Data analysis was performed with MassHunter Workstation 10.1 software (Agilent Technologies, Waldbronn, Germany).
Analyses of Behavior During the Exposure
To assess the influence of the antidepressant on the swimming behavior of fish during the exposure, three photos of each aquarium were taken daily. Five minutes after removal of the black foil from the aquaria three photos of each tank were taken with 5-min intervals in between, using a Panasonic DMC-TZ56 camera. Photos were taken between 1 and 4 p.m. before feeding. A white sheet of paper was placed behind the aquaria to obtain a bright background for better contrast, and all photos were taken from the aquarium´s front side. During the experiment with eggs and larvae, photos were taken every day within the period from one week after yolk sac consumption onwards until sampling. In the study with juvenile brown trout photos were taken every day from the second day of exposure onwards until the end of the experiment (01 August –23 August 2018). For each photograph, the number of fish in the upper and lower half of the aquarium was determined and. From the three photos per treatment and per day, a mean value was calculated.
Analyses of Behavior Under Stressful Conditions
Swimming behavior under stressful conditions was analyzed in an artificial swimming measurement device (ASMD) according to Ziegler et al. (2020b). Fish were transferred into four small aquaria (17 × 17 × 8.5 cm) which were surrounded by white polystyrene plates. Each aquarium was monitored by an individual camera (Basler acA 1,300–60 gm, 1.3 MP resolution, Basler AG, Ahrensburg, Germany, lens: 4.5–12.5 mm; 1:1–2: IR1/2”). To obtain optimal illumination, four lamps were adjusted to face the top of the polystyrene enclosure for indirect bright illumination. Five brown trout larvae were transferred into each of the four small aquaria containing 500 ml of the respective test solution, and their individual movement was recorded for 20 min. In the case of juvenile brown trout, three individuals per tank were assessed in 1 L of the respective solution. The respective first 2 min of each recording were neglected to account for the acclimation of fish to the test conditions. Video sequences of the remaining 18 min were analyzed for the parameters “total distance moved”, “mean velocity” and “time of no movement (inactivity)”. The individuals were centre-point tracked with the program EthoVision XT12 (Noldus Information Technology BV, Wageningen, The Netherlands). Due to occasional difficulties in automatic tracking, swimming tracks were manually corrected when necessary.
Cortisol Content
The cortisol content of juvenile fish exposed to venlafaxine was measured with the commercially available fish cortisol ELISA Kit by Cusabio Technology LCC (Houston, Texas, United States). The samples of the dorsal body portion were manually homogenized with a pestle in PBS buffer (pH 7.6, 0.1 M) (tissue/buffer ratio 1:11 w/v). The homogenates were frozen at −20 °C, thawn at room temperature, frozen and re-thawn again, and then centrifuged for 5 min at 5,000 rpm. Then, the supernatants were stored at −80 °C. Immediately before testing, the supernatants were thawn and diluted with the kit´s sample buffer (supernatant/buffer ratio 1:5 v/v). In the pre-coated 96-well plates provided, 50 µL of the respective standard solution or 50 µL sample as well as 50 µL assay buffer were pipetted into each well (except for the predetermined blank), followed by a 40 min incubation at 37 °C. After three washing cycles with washing buffer, 100 µL horseradish peroxidase (HRP)-conjugate was pipetted into each well (except for the blank) and incubated at 37 °C for 30 min again. After five further washing cycles, 90 µL of 3,3’,5,5’-Tetramethylbenzidine (TMB) solution was added into each well and the plate was incubated at 37 °C for 20 min. Finally, 50 µL of the stop solution were added, and the emission was determined photometrically at 405 nm and at 570 nm for wavelength correction. The concentrations were calculated using data corrected for the blank and wavelength fitted to a four parameter logistic standard curve. Results are expressed as ng/mL.
Statistical Analysis
Statistical analyses were performed with the programmes SAS JMP 14 (Cary, North Carolina, USA) and R 3.5.0 (Vienna, Austria) (packages: lme4, coxme). Mortality and hatching rates were analyzed with a cox mixed effect model (aquarium identity as random factor). Weight, length, heart rate of brown trout larvae and total distance moved in the ASMD were assessed with a nested ANOVA and subsequent post-hoc Dunnett’s test. Whenever data were not normally distributed, a non-parametric Kruskal-Wallis test was performed with a subsequent post-hoc Steel with control. If homogeneity of variance was not achieved, a Welch ANOVA with a subsequent post-hoc Dunnett’s test was used. Data for behavior during exposure were analyzed with a generalized linear mixed model (aquarium identity as random factor) and subsequent post-hoc Dunnett’s test. Cortisol content, time-dependant mean velocity and time of no movement in the ASMD were analyzed with a linear mixed model (aquarium identity as random factor).
CRED
Criteria for reporting and evaluating ecotoxicity data (CRED) are given in the Supplementary Material and aim to improve the evaluation of ecotoxicity studies (Moermond et al., 2016). CRED is used to improve the transparency, reproducibility and consistency of reliability with different reliability and relevance criteria to increase the chance, that an ecotoxicological study is considered for regulatory purposes (Moermond et al., 2016).
Results
Physico-Chemical Water Parameters
Physico-chemical water parameters were determined at the beginning and the end of the two experiments. Temperature, oxygen content, pH and conductivity were in an acceptable range in both experiments (brown trout larvae: mean temperature: 7 °C: 7.25 ± 0.26 °C; 11 °C: 11.37 ± 0.23 °C; mean oxygen content: 7 °C: 11.18 ± 0.33 mg/L; 11 °C: 9.92 ± 0.06 mg/L; mean pH: 7 °C: 8.26 ± 0.23; 11 °C: 7.90 ± 0.11; mean conductivity: 7 °C: 489.2 ± 28.3 μS/cm; 11 °C: 469.8 ± 2.3 μS/cm; juvenile brown trout: mean temperature: 7.01 ± 0.26°C; mean oxygen content: 11.24 ± 0.08 mg/L; mean pH: 7.43 ± 0.28; mean conductivity: 489.6 ± 6.75 μS/cm). In the experiment with eggs and larvae, nitrite levels were in an acceptable range (0.05–0.1 mg/L) during the experiment and at the end at 7 °C. Only at the very end of the experiment at 11 °C the nitrite level was elevated (0.6 mg/L). In the experiment with juvenile brown trout, the nitrite level was determined at the end of the experiment and was shown to be in an acceptable range (0.05 mg/L). Further details are given in the Supplementary Material.
Venlafaxine Analysis in Water and Tissue
Water Samples
Venlafaxine concentrations were below the limit of detection in all control treatments. Recovery rates of venlafaxine were at least 75% of the nominal concentrations. In most cases, the real water concentrations were 4–20% lower than the nominal concentrations, except for the highest concentration in the experiment with eggs and larvae (Table 1). Here, the concentrations were up to 6% higher than nominal concentrations. The water concentrations of the 100 μg/L venlafaxine degradation control in the experiment with juvenile brown trout (real water concentration: 75.79 ± 1.24 μg/L) was similar to the concentrations of the 100 μg/L venlafaxine treatment with fish (real water concentration: 75.80 ± 1.78 μg/L). Overall, the real venlafaxine concentrations in water were in good accordance the nominal concentrations (Table 1 and Table 2).
Tissue Samples
Results for venlafaxine residues in fish tissue samples made evident that tissue concentrations correlated with the respective concentrations in the exposure medium. Tissue concentrations in brown trout larvae exposed at 7 °C were similar to those in juvenile fish (Table 1 and Table 2). In control animals, venlafaxine concentrations were below the limit of detection of 5 ng/L. Bioconcentration factors ranged from 13.9 for the treatments with the lower venlafaxine concentrations to 3.5 for fish exposed to the highest test concentration. This could be shown for both life stages (Table 1 and Table 2).
Experiment With brown Trout Eggs and Larvae
Mortality of brown trout larvae was elevated in fish exposed to the highest venlafaxine concentration (1,000 μg/L) compared to the other exposure groups (Figure 1). This trend was observed at 7 °C (cox mixed-effects model: df = 4, χ2 = 9.4954, p = 0.04984, post-hoc Dunnett’s test [control|1,000 μg/L] p = 0.0563) with a prominent increase in the mortality from day 98 to day 109 to about 15% at the end of the experiment (Figure 1 A), the time of completion of the yolk sac consumption and the first food intake of the larvae. At 11 °C mortality of larvae exposed to 1,000 μg/L (28.89 ± 3.14%) was about 44% higher than control mortality (20 ± 7.2%) (Figure 1 B), but not significantly different (cox mixed-effects model: df = 4, χ2 = 4,3496, p = 0.3608). At 11 °C from day 62 onwards overall mortality increased resulting in an average mortality of about 21.33% at the end of the experiment (Figure 1 B). At 7 °C overall mortality of fish was 6.67% only.
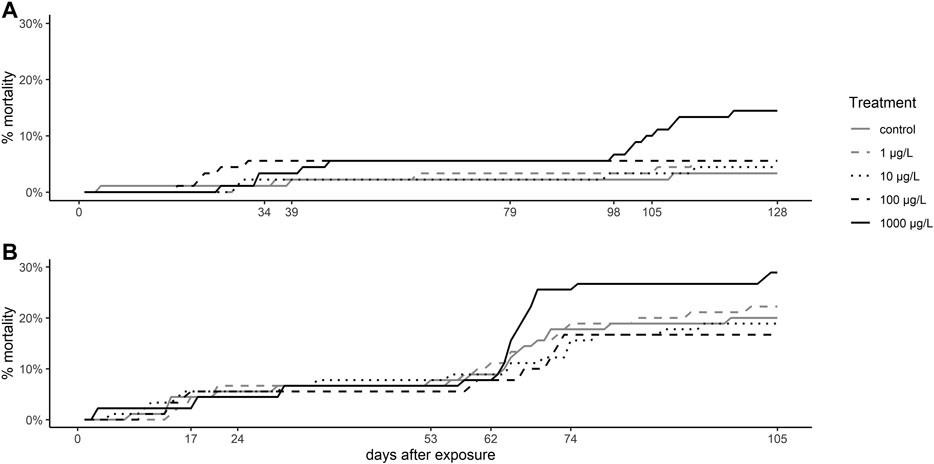
FIGURE 1. Mortality of brown trout larvae. Mortality rate of brown trout larvae exposed (A) at 7 °C, and (B) at 11 °C. Depicted is the cumulative mortality over time.
The hatching rate at both temperatures did not differ significantly between control and the respective venlafaxine treatments (cox mixed-effects model: 11 °C: df = 4, χ2 = 8.8912, p = 0.06388; 7 °C: df = 4, χ2 = 126.13, p < 0.0001, post-hoc Dunnett’s test revealed no difference to the control). Also, the heart rate was not affected by venlafaxine at both temperatures (nested ANOVA: 7 °C: df = 4.10, F = 3.6544, p = 0.0099, post-hoc Dunnett’s test revealed no difference to the control; 11 °C nested ANOVA: df = 4.10, F = 1.9067, p = 0.121). As it could be expected, fish exposed at 11 °C displayed an approximately 30 bpm higher heart rate than fish exposed at 7 °C.
The impact of venlafaxine on body length and weight became more evident in fish exposed at 7 °C rather than at 11 °C. Here, fish exposed to ≥10 μg/L venlafaxine were significantly smaller, and fish exposed to the highest venlafaxine concentration were also significantly lighter than control fish (length: Kruskal-Wallis test: df = 4, χ2 = 50.6227, p < 0.0001, post-hoc Steel with control (control|10 μg/L) p = 0.0093 (control|100 μg/L) p < 0.0001 (control|1,000 μg/L) p < 0.0001 μg/L; weight: Kruskal-Wallis test: df = 4, χ2 = 12.6183, p = 0.0133, post-hoc Steel with control (control|1,000 μg/L) p = 0.0053). At 11 °C, in particular fish exposed to 100 μg/L venlafaxine were significantly smaller than control fish, however no significant difference was observed (weight: Welch-ANOVA: df = 4,167.97, F = 1.4336, p = 0.225; length: nested ANOVA: df = 4.10; F = 3.5667; p = 0.0073; post-hoc Dunnett’s test [control|100 μg/L] p = 0.0064). Although not significantly different, fish exposed to either venlafaxine concentration were on the average smaller and lighter than control fish.
Analysis of Behavior During Exposure
Regarding behavior during exposure, larvae exposed to 100 and 1,000 μg/L venlafaxine at 7 °C stayed more often in the upper part of the respective aquarium than the control fish (Figure 2A) (generalized linear mixed model, df = 4, F = 29.6409, post-hoc Dunnett's test [control|100 μg/L] p = 0.0037 [control|1,000 μg/L] p < 0.001). Whereas this effect became obvious in larvae exposed to 1,000 μg/L right from the first photos taken, in fish exposed to 100 μg/L it could be observed first from day 114 onwards (Figure 2). Larvae exposed to 1,000 μg/L venlafaxine at 11 °C showed an increased sojourn frequency in the upper part of the aquaria during the entire experiment, whereas for fish exposed to 100 μg/L venlafaxine such behavior could not be observed (Figure 2 B) (generalized linear mixed model, df = 4, F = 15.2872, post-hoc Dunnett's test (control|100 μg/L) p = 0.278 (control|1,000 μg/L) p < 0.001).
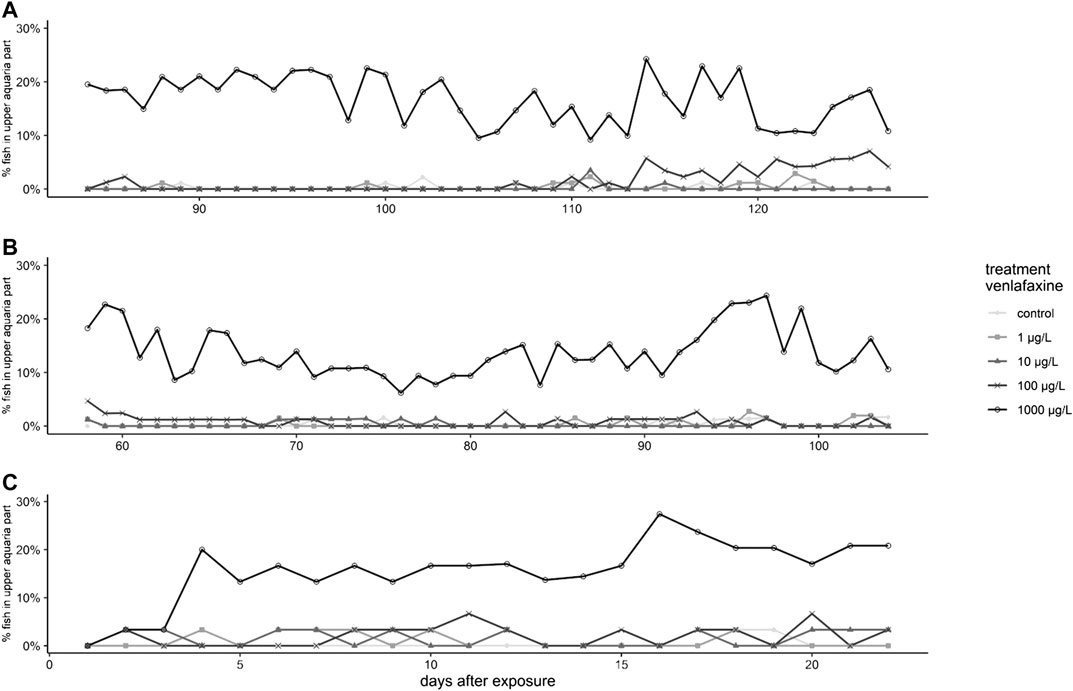
FIGURE 2. Results for the behavior of brown trout during the exposure. (A): Brown trout larvae exposed at 7 °C, (B): Brown trout larvae exposed at 11 °C, (C): Juvenile brown trout, depicted are daily mean percentage of fish observed in the upper half of the aquaria.
Analsyis of Behavior in Stressful Environment
Nominal venlafaxine concentrations affecting fish behavior under stressful conditions were lower than effective nominal concentrations affecting behavior during exposure. Larvae exposed to 100 and 1,000 μg/L venlafaxine at 7 °C swam less during the time of recording compared to control fish (Table 1, Figure 3 A) (Welch-ANOVA: df = 4.34.003, F = 14.5522, p < 0.0001, post-hoc Dunnett's test (control|100 μg/L) p = 0.0228 (control|1,000 μg/L) p < 0.0001). Likewise, fish exposed to 10 and 1,000 μg/L venlafaxine at 11 °C swam less than respective control fish. For fish exposed to 100 μg/L, data were more variable and not significantly different, however, a trend became visible (Table 1, Figure 3 D). (Welch-ANOVA: df = 4.29.399, F = 30.6773, p < 0.0001, post-hoc Dunnett's test (control|10 μg/L) p = 0.018 (control|100 μg/L) p = 0.0691 (control|1,000 μg/L) p < 0.0001). With regard to the temporal pattern during the recording time, control fish showed a low locomotor activity at the beginning of the recording but increased activity over time during the measurement (Figure 3 B + C + E + F). In contrast, fish exposed to 1,000 μg/L did not show such a temporal relationship with regard to mean velocity and inactivity (time of no movement). They constantly showed a low mean velocity and a constantly long time of inactivity during the recording time, with data being significantly different from controls (mean velocity: 7 °C: Linear mixed model: df = 4,10, F = 4.3551, p = 0.02694, post-hoc Dunnett’s test (control|1,000 μg/L) p = 0.00206; 11 °C: Linear mixed model: df = 4.10, F = 4.728, p = 0.02114, post-hoc Dunnett’s test (control|1,000 μg/L) p < 0.001; time of no movement: 7 °C: Linear mixed model: df = 4,10, F = 4.8471, p = 0.01961, post-hoc Dunnett’s test (control|1,000 μg/L) p = 0.00133; 11 °C: Linear mixed model: df = 4,10, F = 5.1766, p = 0.01601, post-hoc Dunnett’s test (control|1,000 μg/L) p < 0.001).
Experiment With Juvenile brown Trout
Exposure of juvenile brown trout to venlafaxine did not affect mortality independent of the exposure concentration (cox mixed-effects model: df = 4, χ2 = 13.215, p = 0.01027, post-hoc Dunnett’s test revealed no difference to control). Likewise, length and weight of juvenile brown trout exposed to venlafaxine did not differ significantly from control fish (weight: Welch-ANOVA: df = 4.66.624, F = 1.0271, p = 0.3999; length: Welch-ANOVA: df = 4.68.448, F = 0.7528, p = 0.5596).
Analysis of Behavior During Exposure
Only few control fish sojourned in the upper half of the respective aquarium (less than 1%). Significant differences occurred in fish exposed to the highest venlafaxine concentration with about 15% of fish staying in the upper half of the aquarium (Figure 2C, Table 2) [generalized linear mixed model, df = 4, F = 5.6349, post-hoc Dunnett's test (control|1,000 μg/L) p < 0.001].
Analysis of Behavior in Stressful Environment
Also, in the artificial swimming measurement device (ASMD), behavior of brown trout exposed to the highest concentration of venlafaxine was significantly different to the one of control fish. Exposed fish swam significantly less than controls (Table 2, Figure 3 G) [total distance moved: Welch-ANOVA: df = 4,14.735, F = 4.026, p = 0.021, post-hoc Dunnett's test (control|1,000 μg/L) p = 0.0174]. Similar to control brown trout larvae, juvenile fish swam slower at the beginning of the exposure and increased swimming velocity over recording time (Figure 3 H + I). Juvenile fish exposed to 1,000 μg/L venlafaxine showed a trend toward reduced velocity and longer inactivity, but the difference to the control was not as distinct as in larvae, and no significant differences could be revealed (linear mixed model: mean velocity: df = 4,10, F = 2.1353, p = 0.1508; time of no movement: df = 4,10, F = 2.3322, p = 0.1265).
Cortisol
Tissue cortisol levels in juvenile brown trout were not affected by exposure to venlafaxine (linear mixed model: df = 4,119.02, F = 1.4114, p = 0.234,370) (Table 2). However, there was a significant impact of the ASMD on cortisol levels of fish. Animals assessed in the ASMD had a significant higher tissue cortisol concentration (7.97 ng/ml) compared to fish not assessed in the measurement device (4.57 ng/ml) (linear mixed model: df = 1,119.37, F = 9.8669, p = 0.002122).
Discussion
As a basis for an evaluation of the risk posed by the antidepressant venlafaxine to aquatic ecosystems, the present study provides data for effects of this drug on two life stages of brown trout complemented by analytical data in water and exposed biota. Fish were exposed to four concentrations of venlafaxine with the lowest concentration representing an environmentally relevant exposure situation, e.g. in wastewater dominated surface waters (Lajeunesse et al., 2008; Lajeunesse et al., 2012; Schlüsener et al., 2015).
In both experiments, the real water concentrations were close to the nominal concentrations. Venlafaxine tissue concentrations correlated well with the respective water concentrations with low venlafaxine concentrations of the aqueous medium resulting in higher BCFs than for higher concentrations in the exposure medium (Table 1 and Table 2). When comparing the tissue concentrations between the two life stages, juvenile brown trout exposed to 1,000 μg/L venlafaxine for four weeks only accumulated equal or even slightly higher amounts of venlafaxine than larvae exposed to the same concentration for five months. Also, for the other concentrations, accumulation in juvenile and larval fish were in a comparable range, even though the larvae were exposed five times longer than juvenile fish. An explanation for this observation might be, that in larvae, the gastro-intestinal system and the gills are still under development and not yet fully functional which may have led to a lower uptake of the chemical (Wells and Pinder, 1996). It is also possible that, tissue concentrations may have also reached a saturation plateau in both life stages exposed (Meador et al., 2017). David et al. (2018) reported venlafaxine concentrations in brain tissue of roach, exposed for 15 days to wastewater (356 ± 39 ng/L venlafaxine), in the low ng/g range with a bioconcentration factor (BCF) of 0.7. In white sucker from a creek in iowa, U.S.A. (venlafaxine concentration 102 ng/L), venlafaxine brain tissue concentrations of 0.1 ± 0.1 ng/g were measured (Schultz et al., 2010). In rainbow trout exposed to wastewater (venlafaxine concentration 330 ± 20 ng/L), venlafaxine concentrations of 21 ± 11 ng/g were found in the liver (BCF = 63) and 3.1 ± 1.4 ng/g in the brain (BCF = 9) while no venlafaxine was detected in muscle tissue (Grabicova et al., 2014). In brown trout, concentrations of up to 3.5 ± 1.7 ng/g were found in liver (BCF = 15) and 16 ± 4 ng/g in kidney (BCF = 37) after exposure in a Czech river downstream a wastewater treatment plant (venlafaxine concentration 65 ± 34 ng/L) for 18 months. In the same study, no venlafaxine could be detected in brain and muscle tissue of exposed fish (Grabicova et al., 2017). Comparing our results with literature data, it becomes evident that venlafaxine can be accumulated also in muscle tissue, when fish are exposed to high concentrations of the antidepressant for longer time (up to 5 months). However, literature data indicate a higher accumulation in other organs, at least in liver and kidney, compared to muscular tissue. The comparatively low BCFs reported for brain may result from the rather low octanol-water partition coefficient log Pow of 2,74 (DrugBank, 2020) and thus low lipophilicity of venlafaxine.
The overall mortality of larvae was generally higher when exposed at 11 °C (21.33%) than at 7 °C (6.67%). The high nitrite levels at the end of the experiment at 11 °C can be excluded as mortality reason for this, since mortality increased between day 95 and 106 of exposure, and nitrite levels were still low (0.1 mg/L) and thus well acceptable at day 106 (Smith and Williams, 1974; Brown and McLeay, 1975; Molony, 2001). Furthermore, it is known that salmonid eggs and larvae are more resistant to nitrite than adult fish due to the larvae size, and therefore higher surface-area-to-volume ratio and the higher oxygen uptake through the skin (Bartlett and Neumann, 1998). A more likely reason for the higher mortality is the fact that fish developed quicker at 11 °C than at 7 °C and started earlier to feed on exogenous food. This transition to exogenous feeding represents an important bottleneck in the salmonid development and is generally associated with a higher mortality risk (Klemetsen et al., 2003). The increased mortality at 11 °C may be attributed to the smaller and lighter individuals at this temperature that reasonably did not survive the faster transition to exogenous feeding. Even though, at both temperatures, the increase in the mortality of larvae exposed to 1,000 μg/L venlafaxine was evidently higher than in controls, this difference was represented by a statistical trend at 7 °C only but was not significant at 11 °C. In fathead minnow, even lower concentrations of 305 ng/L venlafaxine led to an increased mortality (Schultz et al., 2011), and white-seabream larvae have reported to show a reduced survival rate when exposed to 100 μg/L venlafaxine (Rodrigues et al., 2019). Also other antidepressants are known to increase mortality as, e.g., fluoxetine with a 50% lethal concentration (LC50) of 546 μg/L in mosquitofish (Henry and Black, 2008). However, in other studies, meagre, zebrafish and fathead minnow mortality was not affected by venlafaxine (Parrott and Metcalfe, 2017; Maulvault et al., 2018; Sehonova et al., 2019) as it was the case for juvenile brown trout in our study.
Brown trout larvae exposed to 1,000 μg/L venlafaxine were generally smaller and lighter compared to the controls. This can be explained by the fact that antidepressants are known to alter the appetite of exposed organisms resulting in reduced food uptake and weight loss, as it was reported, e.g., in humans, rats and fish (Stahl, 1998; de Oliveira et al., 2004; Mennigen et al., 2010; Hedgespeth et al., 2014). We observed more food leftovers in all tanks containing 1,000 μg/L venlafaxine indicating less food uptake by fish in these exposure groups. Unfortunately, we did not have the possibility to quantify the amount of food ingested in our experiment. In general, however, the reduced food uptake may be confounded by the higher mortality of venlafaxine-exposed larvae. Regarding fish growth in general, it became evident that long-time exposure of hatched larvae to venlafaxine induced stronger effects than short-time exposure of juvenile fish, and effects became more evident at 7 °C than at 11 °C presumably due to the longer exposure time (105 days for larvae at 11°C, 128 days for larvae at 7°C, 24 days for juveniles at 7 °C). Due to co-variation and, possibly, concurrence of the test parameters, it cannot be decided which of the test parameters are more relevant to explain the observed effects. In previous studies, venlafaxine did not exert effects on growth, particularly weight and length of fish (Painter et al., 2009; Maulvault et al., 2018; Parrott and Metcalfe, 2019). The shorter exposure time in the studies of (Maulvault et al., 2018) and (Painter et al., 2009) which was below 28 days, and the lower exposure concentrations (max. 88 μg/L) used by Parrott and Metcalfe (2019) can likely account for these differences. Different species-specific sensitivities can also be of importance in this context. Several other antidepressants like citalopram, fluoxetine (SSRIs) and mianserin, a tetracyclic antidepressant, are known to affect food intake and growth in fish: In a previous study we showed that brown trout larvae and juveniles were smaller and lighter than controls when exposed to 1,000 μg/L citalopram (Ziegler et al., 2020b). Kellner et al. (2015) showed that sticklebacks exposed to at least 0.15 μg/L citalopram had a reduced food intake. Similarly, goldfish exposed to 54 μg/L fluoxetine showed a reduced food intake and lower weight gain (Mennigen et al., 2010), and zebrafish exposed to either 10 μg/L fluoxetine or 10 μg/L mianserin were shorter compared to control fish (Wu et al., 2017).
Generally, hatching time and the heart rate of fish seem not to be parameters sensitive to antidepressants. Regarding time to hatch, in our experiment with brown trout no impact of venlafaxine became evident. Also, in fathead minnow, the hatching rate was not impacted by venlafaxine applied in concentrations up to 88 μg/L (Parrott and Metcalfe, 2019). Likewise, zebrafish exposed to up to 3 mg/L venlafaxine did not show any difference in their hatching rate (Sehonova et al., 2019). Also other antidepressants did not alter the hatching rate of fish, like fluoxetine in medaka (Foran et al., 2004), sertraline in zebrafish (Sehonova et al., 2019) or citalopram in brown trout (Ziegler et al., 2020b). In addition, the heart rate, which was assessed one week after hatching was completed, did not show any differences between treated and control fish. Sehonova et al. (2019) exposed zebrafish and western-clawed frogs (Xenopus tropicalis) up to 3 mg/L venlafaxine or 1 mg/L sertraline and also did not observe changes in heart rate by either venlafaxine or sertraline. In a previous experiment, in which brown trout larvae were exposed to the antidepressant citalopram, similarly, the heart rate was not influenced by citalopram applied in concentrations up to 1 mg/L (Ziegler et al., 2020b).
Analysis of Behavior During Exposure
Both larvae of brown trout and juvenile fish showed higher preference for the upper half of the aquarium when exposed to venlafaxine. This was true for fish exposed to 1,000 μg/L venlafaxine during the entire experiment, but also larvae exposed to 100 μg/L at 7 °C exhibited such behavior at day 114 onwards presumably due to the longer exposure time compared to larvae at 11 °C. Juvenile brown trout started to sojourn more frequently in the upper part of the aquarium from exposure day 4 onwards. As previously pointed out by (Ziegler et al., 2020b), the interpretation of such behavior requires consideration of (anti)anxiety-modulated preference-associated movement such as geotaxis and scototaxis. Under control conditions, brown trout larvae and juveniles mostly stayed in the lower part of the water column or even close to the bottom of the tank (control, Table 1 and Table 2). Fish exposed to venlafaxine, however, stayed in the upper part of the aquarium, which was far better illuminated than the dark bottom of the aquarium, after removal of the black cover foil. In the novel tank test, where a single zebrafish is placed in a new environment, increased residence near the water surface (reduced geotaxis) was described to be due to reduced anxiety behavior and increased boldness (Maximino et al., 2010a; Stewart et al., 2012). Also Maximino et al. (2010b) reported the preference of fish for a lighter area (reduced scototaxis) to result from anti-anxiety. Even though, general toxicity cannot be excluded as a factor, based on the reduced geo- and scototaxis and the fact that venlafaxine acute toxicity (LC50) is higher than 100 mg/L in rainbow trout (Pfizer, 2011; Merck, 2017), the behavioral changes are most likely due to anti-anxiety effect of the substance. Furthermore, effects already appeared with a LOEC of 100 μg/L, while no mortality occurred within this exposure group. This is also supported by the findings of the histopathology of these fish, where no histopathological alterations in the liver where found and therefore no cytotoxicity appeared (Ziegler et al., 2020a). This decreased anxiety can alter natural behaviors, resulting in inter alia increased predation risk and can therefore have an effect on the population level (Saaristo et al., 2018; Ågerstrand et al., 2020).
In a previous experiment, brown trout exposed to the antidepressant citalopram, similarly, resided more often in the upper part of the aquarium (Ziegler et al., 2020b). Maulvault et al. (2018) showed, in the novel tank test, that juvenile meagre exposed to 20 μg/L venlafaxine spent more time in the upper aquaria part. Similar results from the novel tank test were also shown for other antidepressants like citalopram in sticklebacks, endler guppies and zebrafish (Sackerman et al., 2010; Olsén et al., 2014; Kellner et al., 2016), fluoxetine in mosquitofish (Henry and Black, 2008), and amitriptyline in zebrafish (Demin et al., 2017; Meshalkina et al., 2018). A consequential increase of the swimming activity as a result of the exposure to antidepressants was also reported by (Kellner et al., 2016; Martin et al., 2017). Despite this compelling evidence, however, occasionally also a decrease in the swimming activity was found after antidepressant exposure (Kellner et al., 2017; Maulvault et al., 2018). Ecological relevance of this behavioral endpoint is given, because anxiety in fish is related inter alia to predation risk and foraging efficacy (Kellner et al., 2016). Our results reveal an effect at 100 μg/L venlafaxine after five months exposure. Even though this is at least 100 fold higher than environmentally relevant concentrations, with regard to safety factors a no observed effect concentration (NOEC) of 10 μg/L for the behavior during the exposure, should not be ignored when assessing the environmental risk of venlafaxine.
Analysis of Behavior in Stressful Environment
In the ASMD, brown trout larvae and juveniles exposed to venlafaxine showed a decreased total distance moved during the 18 min recording time. For control juveniles and larvae, the mean velocity increased with recording time, and the time of inactivity decreased over time. This behavioral pattern is likely to be explained by freezing behavior at the beginning of confrontation with the new setting, shifting to escape behavior toward the end of the recordings. It disappeared in fish exposed to 1,000 μg/L of the drug: these fish constantly showed a low mean velocity and long times of inactivity. Also, in juvenile fish, the same effect became evident when exposed to 1,000 μg/L venlafaxine, although the difference to the controls was not significant. The cortisol concentrations measured in these individuals proved that a sudden translocation into an artificial new setting, the ASMD, is a stressful procedure for fish (Wendelaar Bonga, 1997). In this context, venlafaxine-exposed fish proved to be less stressed and more ‘relaxed' under these conditions compared to controls. This reflects the anxiolytic mode of action of venlafaxine in humans. Venlafaxine is a very receptor specific antidepressant (Holliday and Benfield, 1995) and to our knowledge no mutagenicity or teratogenicity was reported yet (Furu et al., 2015). Moreover, LC50-values of venlafaxine are regarded above 100 mg/L in rainbow trout (Pfizer, 2011; Merck, 2017), and our highest test concentrations are far below this LC50. In addition, effects already appeared at concentrations down to 10 μg/L, where no effects on mortality were apparent. Furthermore, the histopathology of these same fish did also not reflect general toxicity (Ziegler et al., 2020a). Therefore, it is unlikely that the behavioral changes are due to unspecific toxicity, but most likely the effects shown here are due to the anti-anxiety mode of action of venlafaxine. Furthermore, in larval brown trout, this anxiolytic effect was stronger and occurred at lower concentrations than in juveniles, which could be due to the much longer exposure time of the larvae. However, different sensitivities with respect to the life stage cannot be excluded as Schwarz et al. (2017) also showed differences in the sensitivity of different life stages of brown trout to the pharmaceutical diclofenac.
A retarded swimming in the same experimental setup under stressful conditions was already observed in brown trout exposed to citalopram (Ziegler et al., 2020b). Painter et al. (2009) showed reduced escape behavior in venlafaxine-exposed fathead minnow larvae. And also in other fish species, a reduced activity under stressful conditions after exposure to different antidepressants was reported frequently (Demin et al., 2017; Kellner et al., 2017; Saaristo et al., 2017; Maulvault et al., 2018; Meshalkina et al., 2018). But also in respect to this testing procedure, contradictory results do exist. Three-spined sticklebacks exposed to 1.5 μg/L citalopram for 21 days showed decreased freezing and increased swimming behavior in the novel tank test (Kellner et al., 2016).
Hazard Assessment
The effects we found in our study, in concentration ranges down to 10 μg/L, result in a NOEC of 1 μg/L for behavior under stressful conditions and length of brown trout larvae. This concentration range is comparable to results found in literature, including (Painter et al., 2009; Melnyk-Lamont et al., 2014; Maulvault et al., 2019; Sehonova et al., 2019). Fick et al. (2010) calculated the critical environmental concentration (CEC) for 500 pharmaceuticals and derived a CEC for venlafaxine of 6.112 μg/L. Taken together, results from literature and our NOEC are below this CEC and partly below measured environmental concentrations in surface waters and wastewater effluents (Schultz et al., 2010; Lajeunesse et al., 2012; Golovko et al., 2014). Accordingly, the environmental risk of venlafaxine cannot be excluded. Additionally, the antidepressant venlafaxine was already in focus of risk assessment studies, in which an environmental risk by venlafaxine could be deduced (Fernández-Rubio et al., 2019; Zhou et al., 2019). As a last consequence, venlafaxine and its main metabolite were incorporated to the surface water watch list of the water framework directive in August 2020 (EU, 2020).
Conclusion
We could demonstrate that, in both larvae and juveniles, venlafaxine exerts an anti-anxiety effect in brown trout as it does in humans, with a lowest observed effect concentration (LOEC) of 10 μg/L which is only a single decimal order of magnitude above environmentally relevant concentrations. The most sensitive parameters were the cautiousness in an unfamiliar surrounding area, together with decreased growth. In nature, the combination of these changes likely makes early life stages of fish to become an easier prey for predators. Due to such high potential relevance for the survival of fish offspring and the rather small difference between LOEC and environmentally relevant concentrations, the risk posed by venlafaxine in the environment should not be neglected, particularly when interacting in concert with other pharmaceuticals.
Data Availability Statement
The datasets presented in this study can be found in online repositories. The names of the repository/repositories and accession number(s) can be found below: https://effectnet-seek.bioquant.uni-heidelberg.de/investigations/9.
Ethics Statement
The animal study was reviewed and approved by Animal welfare committee of the Regional Council of Tübingen, Germany (authorisation ZO 2/16).
Author Contributions
MZ conceived, designed and performed the experiments, analysed the data and prepared the figures and tables and wrote the first draft of the paper and edited according to the coauthors. MB, RB, SS, and ST analysed part of the Data and contributed parts to the first draft of the paper as well as reviewed drafts of the paper and approved the final draft. H-RK, CH, and CZ contributed reagents/materials/analysis tools reviewed drafts of the paper and approved the final draft. RT conceived and designed the experiments and contributed reagents/materials/analysis tools as well as reviewed the first draft of the paper and approved the final draft.
Funding
We would like to thank the Ministry for Science, Research and Arts of Baden-Württemberg for funding the Effect-Net (Effect Network in Water Research) project, which is part of the Water Research Network Baden-Württemberg (Wassernetzwerk Baden-Württemberg). We acknowledge support by Open Access Publishing Fund of University of Tübingen.
Conflict of Interest
The authors declare that the research was conducted in the absence of any commercial or financial relationships that could be construed as a potential conflict of interest.
Acknowledgments
Further, we thank the Open Access Publishing Fund of University of Tübingen for support. Thanks go to Thomas Braunbeck for coordinating the project. Furthermore, the authors thank Stefanie Jacob, Stefanie Krais, Elisabeth May, Katharina Peschke, Hannah Schmieg and Sabrina Wilhelm for help in the laboratory and technical assistance, and Stefanie Dietz for comments on the manuscript.
Supplementary Material
The Supplementary Material for this article can be found online at: https://www.frontiersin.org/articles/10.3389/fenvs.2020.586584/full#supplementary-material.
References
Acuña, V., von Schiller, D., García-Galán, M. J., Rodríguez-Mozaz, S., Corominas, L., Petrovic, M., et al. (2015). Occurrence and in-stream attenuation of wastewater-derived pharmaceuticals in Iberian rivers. Sci. Total Environ. 503-504, 133–141. doi:10.1016/j.scitotenv.2014.05.067
Ågerstrand, M., Arnold, K., Balshine, S., Brodin, T., Brooks, B. W., Maack, G., et al. (2020). Emerging investigator series: use of behavioural endpoints in the regulation of chemicals. Environ. Sci.: Progress and Impacts 22 (1), 49–65. doi:10.1039/c9em00463g
Bartlett, F., and Neumann, D. (1998). Sensitivity of Brown trout alevins (Salmo trutta L.) to nitrite at different chloride concentrations. Bull. Environ. Contam. Toxicol. 60, 340–346. doi:10.1007/s001289900632
Bidel, F., Di Poi, C., Budzinski, H., Pardon, P., Callewaert, W., Arini, A., et al. (2016). The antidepressant venlafaxine may act as a neurodevelopmental toxicant in cuttlefish (Sepia officinalis). Neurotoxicology 55, 142–153. doi:10.1016/j.neuro.2016.05.023
Brown, D. A., and McLeay, D. J. (1975). Effect of nitrite on methemoglobin and total hemoglobin of juvenile rainbow trout. Prog. Fish-Cult. 37 (1), 36–38. doi:10.1577/1548-8659(1975)37[36:Eonoma]2.0.Co;2
Burnett, F., and Dinan, T. G. (1998). Venlafaxine. Pharmacology and therapeutic potential in the treatment of depression. Hum. Psychopharmacol. 13, 153–162. doi:10.1002/(SICI)1099-1077(199804)13:3<153::AID-HUP973>3.0.CO;2-S
David, A., Lange, A., Tyler, C. R., and Hill, E. M. (2018). Concentrating mixtures of neuroactive pharmaceuticals and altered neurotransmitter levels in the brain of fish exposed to a wastewater effluent. Sci. Total Environ. 621, 782–790. doi:10.1016/j.scitotenv.2017.11.265
de Oliveira, R. A., Cunha, G. M., Borges, K. D., de Bruin, G. S., dos Santos-Filho, E. A., Viana, G. S., et al. (2004). The effect of venlafaxine on behaviour, body weight and striatal monoamine levels on sleep-deprived female rats. Pharmacol. Biochem. Behav. 79 (3), 499–506. doi:10.1016/j.pbb.2004.09.001
Demin, K. A., Kolesnikova, T. O., Khatsko, S. L., Meshalkina, D. A., Efimova, E. V., Morzherin, Y. Y., et al. (2017). Acute effects of amitriptyline on adult zebrafish: potential relevance to antidepressant drug screening and modeling human toxidromes. Neurotoxicol. Teratol. 62, 27–33. doi:10.1016/j.ntt.2017.04.002
DrugBank (2020). Venlafaxine. Available at: https://www.drugbank.ca/drugs/DB00285 (Accessed June 18, 2020).
EU (2020). Commission implementing decision (EU) 2020/1161 of 4 August 2020 establishing a watch list of substances for Union-wide monitoring in the field of water policy pursuant to Directive 2008/105. Brussels: EC of the European Parliament and of the Council Official Journal of the European Union.
EU (2006). Richtlinie 2006/88/EG des Rates mit Gesundheits- und Hygienevorschriften für Tiere in Aquakultur und Aquakulturerzeignissen und zur Verhühtung und Bekämpfung bestimmter Wassertierkrankheiten, Amtsblatt der Europäischen Union. L 328/14. Luxemburg.
Fernández-Rubio, J., Rodríguez-Gil, J. L., Postigo, C., Mastroianni, N., López de Alda, M., Barceló, D., et al. (2019). Psychoactive pharmaceuticals and illicit drugs in coastal waters of North-Western Spain: environmental exposure and risk assessment. Chemosphere 224, 379–389. doi:10.1016/j.chemosphere.2019.02.041
Ferrer, I., and Thurman, E. M. (2012). Analysis of 100 pharmaceuticals and their degradates in water samples by liquid chromatography/quadrupole time-of-flight mass spectrometry. J. Chromatogr. A. 1259, 148–157. doi:10.1016/j.chroma.2012.03.059
Fick, J., Lindberg, R. H., Tysklind, M., and Larsson, D. G. (2010). Predicted critical environmental concentrations for 500 pharmaceuticals. Regul. Toxicol. Pharmacol. 58 (3), 516–523. doi:10.1016/j.yrtph.2010.08.025
Fong, P. P., Bury, T. B., Dworkin-Brodsky, A. D., Jasion, C. M., and Kell, R. C. (2015). The antidepressants venlafaxine ("Effexor") and fluoxetine ("Prozac") produce different effects on locomotion in two species of marine snail, the oyster drill (Urosalpinx cinerea) and the starsnail (Lithopoma americanum). Mar. Environ. Res. 103, 89–94. doi:10.1016/j.marenvres.2014.11.010
Fong, P. P., and Molnar, N. (2013). Antidepressants cause foot detachment from substrate in five species of marine snail. Mar. Environ. Res. 84, 24–30. doi:10.1016/j.marenvres.2012.11.004
Fong, P. P., and Hoy, C. M. (2012). Antidepressants (venlafaxine and citalopram) cause foot detachment from the substrate in freshwater snails at environmentally relevant concentrations. Mar. Freshw. Behav. Physiol. 45 (2), 145–153. doi:10.1080/10236244.2012.690579
Foran, C. M., Weston, J., Slattery, M., Brooks, B. W., and Huggett, D. B. (2004). Reproductive assessment of Japanese medaka (Oryzias latipes) following a four-week fluoxetine (SSRI) exposure. Arch. Environ. Contam. Toxicol. 46 (4), 511–517. doi:10.1007/s00244-003-3042-5
Furu, K., Kieler, H., Haglund, B., Engeland, A., Selmer, R., Stephansson, O., et al. (2015). Selective serotonin reuptake inhibitors and venlafaxine in early pregnancy and risk of birth defects: population based cohort study and sibling design. BMJ 350, h1798. doi:10.1136/bmj.h1798
Golovko, O., Kumar, V., Fedorova, G., Randak, T., and Grabic, R. (2014). Seasonal changes in antibiotics, antidepressants/psychiatric drugs, antihistamines and lipid regulators in a wastewater treatment plant. Chemosphere 111, 418–426. doi:10.1016/j.chemosphere.2014.03.132
Grabicova, K., Grabic, R., Fedorova, G., Fick, J., Cerveny, D., Kolarova, J., et al. (2017). Bioaccumulation of psychoactive pharmaceuticals in fish in an effluent dominated stream. Water Res. 124, 654–662. doi:10.1016/j.watres.2017.08.018
Grabicova, K., Lindberg, R. H., Ostman, M., Grabic, R., Randak, T., Larsson, D. G., et al. (2014). Tissue-specific bioconcentration of antidepressants in fish exposed to effluent from a municipal sewage treatment plant. Sci. Total Environ. 488-489, 46–50. doi:10.1016/j.scitotenv.2014.04.052
Hedgespeth, M. L., Nilsson, P. A., and Berglund, O. (2014). Ecological implications of altered fish foraging after exposure to an antidepressant pharmaceutical. Aquat. Toxicol. 151, 84–87. doi:10.1016/j.aquatox.2013.12.011
Henry, T. B., and Black, M. C. (2008). Acute and chronic toxicity of fluoxetine (selective serotonin reuptake inhibitor) in western mosquitofish. Arch. Environ. Contam. Toxicol. 54 (2), 325–330. doi:10.1007/s00244-007-9018-0
HEXAL (2019). Gebrauchsinformation: information für Patienten. Venlafaxin HEXAL® 37,5 mg Hartkapseln, retardiert. Venlafaxin HEXAL® 75 mg Hartkapseln, retardiert. Venlafaxin HEXAL® 150 mg Hartkapseln, retardiert, HEXAL AG, Holzkirchen. Available at: https://www.hexal.biz/praeparate/dokumente/gi/46259701_165x620_lf_it-1575531248.pdf (Accessed June 17, 2020).
Himmelsbach, M., Buchberger, W., and Klampfl, C. W. (2006). Determination of antidepressants in surface and waste water samples by capillary electrophoresis with electrospray ionization mass spectrometric detection after preconcentration using off-line solid-phase extraction. Electrophoresis 27 (5–6), 1220–1226. doi:10.1002/elps.200500693
Holliday, S. M., and Benfield, P. (1995). Venlafaxine. A review of its pharmacology and therapeutic potential in depression. Drugs 49 (2), 280–294. doi:10.2165/00003495-199549020-00010
Kellner, M., Porseryd, T., Hallgren, S., Porsch-Hällström, I., Hansen, S. H., and Olsén, K. H. (2016). Waterborne citalopram has anxiolytic effects and increases locomotor activity in the three-spine stickleback (Gasterosteus aculeatus). Aquat. Toxicol. 173, 19–28. doi:10.1016/j.aquatox.2015.12.026
Kellner, M., Porseryd, T., Porsch-Hällström, I., Borg, B., Roufidou, C., and Olsén, K. H. (2017). Developmental exposure to the SSRI citalopram causes long-lasting behavioural effects in the three-spined stickleback (Gasterosteus aculeatus). Ecotoxicology 27 (1), 12–22. doi:10.1007/s10646-017-1866-4
Kellner, M., Porseryd, T., Porsch-Hällström, I., Hansen, S. H., and Olsén, K. H. (2015). Environmentally relevant concentrations of citalopram partially inhibit feeding in the three-spine stickleback (Gasterosteus aculeatus). Aquat. Toxicol. 158, 165–170. doi:10.1016/j.aquatox.2014.11.003
Klemetsen, A., Amundsen, P.-A., Dempson, J. B., Jonsson, B., Jonsson, N., O'Conell, M. F., et al. (2003). Atlantic salmon Salmo salar L., brown trout Salmo trutta L. and Arctic charr Salvelinus alpinus (L.): a review of aspects of their life histories. Ecol. Freshw. Fish. 12, 1–59. doi:10.1034/j.1600-0633.2003.00010.x
Lajeunesse, A., Gagnon, C., and Sauvé, S. (2008). Determination of basic antidepressants and their N-desmethyl metabolites in raw sewage and wastewater using solid-phase extraction and liquid chromatography-tandem mass spectrometry. Anal. Chem. 80 (14), 5325–5333. doi:10.1021/ac800162q
Lajeunesse, A., Smyth, S. A., Barclay, K., Sauvé, S., and Gagnon, C. (2012). Distribution of antidepressant residues in wastewater and biosolids following different treatment processes by municipal wastewater treatment plants in Canada. Water Res. 46 (17), 5600–5612. doi:10.1016/j.watres.2012.07.042
Liu, Q., He, H., Yang, J., Feng, X., Zhao, F., and Lyu, J. (2020). Changes in the global burden of depression from 1990 to 2017: findings from the global burden of disease study. J. Psychiatr. Res. 126, 134–140. doi:10.1016/j.jpsychires.2019.08.002
Mackul'ak, T., Mosný, M., Škubák, J., Grabic, R., and Birošová, L. (2015). Fate of psychoactive compounds in wastewater treatment plant and the possibility of their degradation using aquatic plants. Environ. Toxicol. Pharmacol. 39 (2), 969–973. doi:10.1016/j.etap.2015.02.018
Martin, J. M., Saaristo, M., Bertram, M. G., Lewis, P. J., Coggan, T. L., Clarke, B. O., et al. (2017). The psychoactive pollutant fluoxetine compromises antipredator behaviour in fish. Environ. Pollut. 222, 592–599. doi:10.1016/j.envpol.2016.10.010
Maulvault, A. L., Camacho, C., Barbosa, V., Alves, R., Anacleto, P., Pousão-Ferreira, P., et al. (2019). Living in a multi-stressors environment: an integrated biomarker approach to assess the ecotoxicological response of meagre (Argyrosomus regius) to venlafaxine, warming and acidification. Environ. Res. 169, 7–25. doi:10.1016/j.envres.2018.10.021
Maulvault, A. L., Santos, L., Paula, J. R., Camacho, C., Pissarra, V., Fogaça, F., et al. (2018). Differential behavioural responses to venlafaxine exposure route, warming and acidification in juvenile fish (Argyrosomus regius). Sci. Total Environ. 634, 1136–1147. doi:10.1016/j.scitotenv.2018.04.015
Maximino, C., de Brito, T. M., da Silva Batista, A. W., Herculano, A. M., Morato, S., and Gouveia, A. (2010a). Measuring anxiety in zebrafish: a critical review. Behav. Brain Res. 214 (2), 157–171. doi:10.1016/j.bbr.2010.05.031
Maximino, C., Marques de Brito, T., Dias, C. A., Gouveia, A., and Morato, S. (2010b). Scototaxis as anxiety-like behavior in fish. Nat. Protoc. 5 (2), 209–216. doi:10.1038/nprot.2009.225
Meador, J. P., Yeh, A., and Gallagher, E. P. (2017). Determining potential adverse effects in marine fish exposed to pharmaceuticals and personal care products with the fish plasma model and whole-body tissue concentrations. Environ. Pollut. 230, 1018–1029. doi:10.1016/j.envpol.2017.07.047
Melnyk-Lamont, N., Best, C., Gesto, M., and Vijayan, M. M. (2014). The antidepressant venlafaxine disrupts brain monoamine levels and neuroendocrine responses to stress in rainbow trout. Environ. Sci. Technol. 48 (22), 13434–13442. doi:10.1021/es504331n
Melvin, S. D. (2017). Effect of antidepressants on circadian rhythms in fish: insights and implications regarding the design of behavioural toxicity tests. Aquat. Toxicol. 182, 20–30. doi:10.1016/j.aquatox.2016.11.007
Mennigen, J. A., Sassine, J., Trudeau, V. L., and Moon, T. W. (2010). Waterborne fluoxetine disrupts feeding and energy metabolism in the goldfish Carassius auratus. Aquat. Toxicol. 100 (1), 128–137. doi:10.1016/j.aquatox.2010.07.022
Merck, (2017). Safety data sheet according to regulation (EC) No. 07/2006, venlafaxine hydrochloride. Available at: https://www.merckmillipore.com/DE/de/product/msds/EMD_BIO-506135?Origin=accelerator-singlestep-msds (Accessed September 12, 2020).
Meshalkina, D. A., Kysil, E. V., Antonova, K. A., Demin, K. A., Kolesnikova, T. O., Khatsko, S. L., et al. (2018). The effects of chronic amitriptyline on zebrafish behavior and monoamine neurochemistry. Neurochem. Res. 43 (6), 1191–1199. doi:10.1007/s11064-018-2536-5
Moermond, C. T., Kase, R., Korkaric, M., and Ågerstrand, M. (2016). CRED: criteria for reporting and evaluating ecotoxicity data. Environ. Toxicol. Chem. 35 (5), 1297–1309. doi:10.1002/etc.3259
Molony, B. (2001). Environmental requirements and tolerances of Rainbow trout (Oncorhynchus mykiss) and Brown trout (Salmo trutta) with special reference to Western Australia: a review, Fisheries Research Report. Western Australia: Department of Fisheries, 130.
Olsén, K. H., Ask, K., Olsén, H., Porsch-Hällström, I., and Hallgren, S. (2014). Reprint of "Effects of the SSRI citalopram on behaviours connected to stress and reproduction in Endler guppy, Poecilia wingei. Aquat. Toxicol. 151, 97–104. doi:10.1016/j.aquatox.2014.02.011
Paíga, P., Santos, L., Ramos, S., Jorge, S., Silva, J. G., and Delerue-Matos, C. (2016). Presence of pharmaceuticals in the Lis river (Portugal): sources, fate and seasonal variation. Sci. Total Environ. 573, 164–177. doi:10.1016/j.scitotenv.2016.08.089
Painter, M. M., Buerkley, M. A., Julius, M. L., Vajda, A. M., Norris, D. O., Barber, L. B., et al. (2009). Antidepressants at environmentally relevant concentrations affect predator avoidance behavior of larval fathead minnows (Pimephales promelas). Environ. Toxicol. Chem. 28 (12), 2677–2684. doi:10.1897/08-556.1
Parrott, J. L., and Metcalfe, C. D. (2019). Assessing the effects of environmentally relevant concentrations of antidepressant mixtures to fathead minnows exposed over a full life cycle. Sci. Total Environ. 648, 1227–1236. doi:10.1016/j.scitotenv.2018.08.237
Parrott, J. L., and Metcalfe, C. D. (2017). Assessing the effects of the antidepressant venlafaxine to fathead minnows exposed to environmentally relevant concentrations over a full life cycle. Environ. Pollut. 229, 403–411. doi:10.1016/j.envpol.2017.06.009
Parrott, J. L., and Metcalfe, C. D. (2018). Nest-defense behaviors in fathead minnows after lifecycle exposure to the antidepressant venlafaxine. Environ. Pollut. 234, 223–230. doi:10.1016/j.envpol.2017.11.049
Pfizer (2011). Material safety data sheet, venlafaxine hydrochloride. Available at: https://pfe-pfizercom-d8-prod.s3.amazonaws.com/products/material_safety_data/PZ01391.pdf (Accessed September 12, 2020).
Rodrigues, A., Borges, F. O., Pissarra, V., Luísa Maulvault, A., Paula, J. R., Bispo, R., et al. (2019). First indication of deleterious impacts in white-seabream larvae (Diplodus sargus) survival and behaviour following acute venlafaxine exposure. Ecotoxicology 28 (6), 612–618. doi:10.1007/s10646-019-02057-7
Saaristo, M., McLennan, A., Johnstone, C. P., Clarke, B. O., and Wong, B. B. (2017). Impacts of the antidepressant fluoxetine on the anti-predator behaviours of wild guppies (Poecilia reticulata). Aquat. Toxicol. 183, 38–45. doi:10.1016/j.aquatox.2016.12.007
Saaristo, M., Brodin, T., Balshine, S., Bertram, M. G., Brooks, B. W., Ehlman, S. M., et al. (2018). Direct and indirect effects of chemical contaminants on the behaviour, ecology and evolution of wildlife. Proc. Roy. Soc. Biol. Sci. B. 285, 20181297. doi:10.1098/rspb.2018.1297.1885
Sackerman, J., Donegan, J. J., Cunningham, C. S., Nguyen, N. N., Lawless, K., Long, A., et al. (2010). Zebrafish behavior in novel environments: effects of acute exposure to anxiolytic compounds and choice of Danio rerio line. Int. J. Comp. Psychol. 23 (1), 43–61.
Schlüsener, M. P., Hardenbicker, P., Nilson, E., Schulz, M., Viergutz, C., and Ternes, T. A. (2015). Occurrence of venlafaxine, other antidepressants and selected metabolites in the Rhine catchment in the face of climate change. Environ. Pollut. 196, 247–256. doi:10.1016/j.envpol.2014.09.019
Schultz, M. M., Furlong, E. T., Kolpin, D. W., Werner, S. L., Schoenfuss, H. L., Barber, L. B., et al. (2010). Antidepressant pharmaceuticals in two U.S. Effluent-impacted streams: occurrence and fate in water and sediment, and selective uptake in fish neural tissue. Environ. Sci. Technol. 44, 1918–1925. doi:10.1021/es9022706
Schultz, M. M., Painter, M. M., Bartell, S. E., Logue, A., Furlong, E. T., Werner, S. L., et al. (2011). Selective uptake and biological consequences of environmentally relevant antidepressant pharmaceutical exposures on male fathead minnows. Aquat. Toxicol. 104 (1–2), 38–47. doi:10.1016/j.aquatox.2011.03.011
Schwabe, U., Paffrath, D., Ludwig, W.-D., and Klauber, J. (2019). Arzneiverordnungsreport. 2019. Berlin: Springer-Verlag GmbH.
Schwarz, S., Schmieg, H., Scheurer, M., Köhler, H. R., and Triebskorn, R. (2017). Impact of the NSAID diclofenac on survival, development, behaviour and health of embryonic and juvenile stages of brown trout, Salmo trutta f. fario. Sci. Total Environ. 607–608, 1026–1036. doi:10.1016/j.scitotenv.2017.07.042
Sehonova, P., Hodkovicova, N., Urbanova, M., Örn, S., Blahova, J., Svobodova, Z., et al. (2019). Effects of antidepressants with different modes of action on early life stages of fish and amphibians. Environ. Pollut. 254 (Pt A), 112999. doi:10.1016/j.envpol.2019.112999
Smith, C. E., and Williams, W. G. (1974). Experimental nitrite toxicity in rainbow trout and chinook salmon. Trans. Am. Fish. Soc. 103 (2), 389–390. doi:10.1577/1548-8659.1974103<389Entirt>2.0.Co2
Stahl, S. M. (1998). How to appease the appetite of psychotropic drugs. J. Clin. Psychiatr. 59 (10), 500–501. doi:10.4088/jcp.v59n1001
Stewart, A., Gaikwad, S., Kyzar, E., Green, J., Roth, A., and Kalueff, A. V. (2012). Modeling anxiety using adult zebrafish: a conceptual review. Neuropharmacology 62 (1), 135–143. doi:10.1016/j.neuropharm.2011.07.037
Thompson, W. A., and Vijayan, M. M. (2020). Environmental levels of venlafaxine impact larval behavioural performance in fathead minnows. Chemosphere 259, 127437. doi:10.1016/j.chemosphere.2020.127437
Wells, P., and Pinder, A. (1996). The respiratory development of atlantic salmon. I. Morphometry of gills, yolk sac and body surface. J. Exp. Biol. 199, 2725–2736.
Wendelaar Bonga, S. E. (1997). The stress response in fish. Physiol. Rev. 77 (3), 591–625. doi:10.1152/physrev.1997.77.3.591
WHO (2019). ATC/DDD index 2020. Available at: https://www.whocc.no/atc_ddd_index/ (Accessed June 18, 2020).
Wittchen, H. U., Jacobi, F., Rehm, J., Gustavsson, A., Svensson, M., Jönsson, B., et al. (2011). The size and burden of mental disorders and other disorders of the brain in Europe 2010. Eur. Neuropsychopharmacol. 21 (9), 655–679. doi:10.1016/j.euroneuro.2011.07.018
Wu, M., Liu, S., Hu, L., Qu, H., Pan, C., Lei, P., et al. (2017). Global transcriptomic analysis of zebrafish in response to embryonic exposure to three antidepressants, amitriptyline, fluoxetine and mianserin. Aquat. Toxicol. 192, 274–283. doi:10.1016/j.aquatox.2017.09.027
Zhou, S., Di Paolo, C., Wu, X., Shao, Y., Seiler, T. B., and Hollert, H. (2019). Optimization of screening-level risk assessment and priority selection of emerging pollutants - the case of pharmaceuticals in European surface waters. Environ. Int. 128, 1–10. doi:10.1016/j.envint.2019.04.034
Ziegler, M., Knoll, S., Köhler, H. R., Tisler, S., Huhn, C., Zwiener, C., et al. (2020b). Impact of the antidepressant citalopram on the behaviour of two different life stages of brown trout. PeerJ. 8, e8765. doi:10.7717/peerj.8765
Keywords: antidepressant, venlafaxine, brown trout, fish, behavior, development
Citation: Ziegler M, Banet M, Bauer R, Köhler H-R, Stepinski S, Tisler S, Huhn C, Zwiener C and Triebskorn R (2021) Behavioral and Developmental Changes in Brown Trout After Exposure to the Antidepressant Venlafaxine. Front. Environ. Sci. 8:586584. doi: 10.3389/fenvs.2020.586584
Received: 23 July 2020; Accepted: 17 December 2020;
Published: 21 January 2021.
Edited by:
Raul Perez Lejano, New York University, United StatesReviewed by:
Zhi Wang, Chinese Academy of Sciences, ChinaWing Shan Kan, Caritas Institute of Higher Education, Hong Kong
Copyright © 2021 Ziegler, Banet, Bauer, Köhler, Stepinski, Tisler, Huhn, Zwiener and Triebskorn. This is an open-access article distributed under the terms of the Creative Commons Attribution License (CC BY). The use, distribution or reproduction in other forums is permitted, provided the original author(s) and the copyright owner(s) are credited and that the original publication in this journal is cited, in accordance with accepted academic practice. No use, distribution or reproduction is permitted which does not comply with these terms.
*Correspondence: Michael Ziegler, michael.ziegler@student.uni-tuebingen.de