Activity level and aggregation behavior in the crustacean gammarid Gammarus insensibilis parasitized by the manipulative trematode Microphallus papillorobustus
- 1Maladies Infectieuses et Vecteurs: Ecologie, Génétique, Evolution et Contrôle, UMR (IRD/CNRS/UM) 5290, Montpellier, France
- 2Department of Wetland Ecology, Estacion Biológica de Doñana, Sevilla, Spain
- 3Département Biologie et Écologie des Vertébrés, Centre de Recherche de la Tour du Valat, Arles, France
- 4Department of Biology, John Abbott College, Sainte-Anne-de-Bellevue, QC, Canada
Hosts manipulated by parasites are profoundly altered organisms exhibiting a broad range of potential modifications. Exploring this multidimensionality is an emerging field. Previous studies have shown that the bird trematode Microphallus papillorobustus induces several behavioral changes in the gammarid Gammarus insensibilis. Knowing that aggregation behavior and reduced activity levels are strategies that limit predation in other species of amphipods, we explored in this study these behavioral responses for infected and uninfected G. insensibilis in the presence of host and non-host predator olfactory cues (bird feces and fish mucus). While uninfected individuals reduced their activity level in the presence of predator cues, infected individuals did not change their activity level in presence of aquatic bird feces. We also studied the gammarid aggregation behavior. Uninfected gammarids in clean water spent significantly more time in aggregates than did infected individuals. Among the uninfected individuals, the aggregation level tended to increase when bird feces and fish mucus were added, but the difference was not significant. Among infected individuals, the level of aggregation was significantly increased only with the bird feces. We discussed our results in the context of the literature on multidimensional manipulations, acknowledging that subtle differences between unparasitized and parasitized gammarids can also be by-products of manipulation on other traits.
Introduction
Altered host phenotypes (e.g., behavior, morphology, physiology) that enhance the probability of parasite transmission have been well documented over the last few decades (reviewed by Moore, 2002; Thomas et al., 2005). These phenotypic alterations can vary greatly in their magnitude, from slight shifts in the percentage of time spent in performing a given activity to the production of complex and spectacular behaviors (Poulin and Thomas, 1999; Moore, 2002; Hughes et al., 2012). While much of the research on host manipulation by parasites has focused on a single or only a few phenotypic traits in the parasitised hosts (usually the most altered ones; Moore, 2002), an important aspect of recent studies has been the recognition that these phenomena usually affect numerous phenotypic traits (i.e., multidimensional manipulation), either simultaneously or sequentially (Benesh et al., 2008; Thomas et al., 2010; Cézilly et al., 2013). For example, trophically transmitted parasites can greatly enhance their probability of transmission to their definitive hosts by simultaneously altering the color and several behavioral aspects of their intermediate hosts (e.g., Yanoviak et al., 2008; see Thomas et al., 2010, for review). In addition to promoting parasite transmission, certain phenotypic changes can also limit the risk of maladaptive host death (Levri, 1998; Kullmann et al., 2008; Parker et al., 2009; Dianne et al., 2011). For example, in the presence of non-host predators, gammarids Gammarus pulex and Gammarus roeseli, respectively parasitised by Pomphorhynchus laevis and Polymorphus minutus, were shown to increase their refuge use (Kaldonski et al., 2007; Médoc and Beisel, 2009) and/or have enhanced escape performance (increased swimming speed; Medoc and Beisel, 2008). Although multidimensionality in host manipulation has been increasingly addressed (Cézilly and Perrot-Minnot, 2005, 2010; Thomas et al., 2010), little is known regarding its extent and how it evolves, even among the most studied models (Thomas et al., 2010).
Here we explored possible novel dimensions in the manipulation exerted by the trematode Microphallus papillorobustus (trematode; Rankin, 1940) in the crustacean gammarid, Gammarus insensibilis (amphipod; Stock, 1966). This salt marsh trematode has a complex life cycle that includes snails from the genus Hydrobia as the first intermediate host, gammaridean amphipods as the second intermediate host, and various aquatic birds as definitive hosts (order Charadriiformes; Rebecq, 1964). M. papillorobustus metacercariae are always encysted in the brain (protocerebrum) of G. insensibilis and induce strong behavioral and physiological alterations in this host, i.e., positive phototaxis, negative geotaxis, aberrant evasive behavior, and higher lipid content (Helluy, 1981, 1984; Ponton et al., 2005). Infected gammarids typically live at the surface of the water while uninfected ones inhabit the bottom (Helluy, 1981; Ponton et al., 2005). It is thought that these multiple behavioral changes increase the vulnerability of gammarids to predation by aquatic birds and therefore promote parasite transmission (Helluy, 1984).
Knowing that aggregation behavior and reduced activity levels are strategies that limit predation in other species of amphipods (Andersson et al., 1986; Krause and Godin, 1994; Wooster, 1998; Dezfuli et al., 2003; Wellnitz et al., 2003), we explored in this study whether M. papillorobustus affects these behavioral responses in G. insensibilis when exposed to either host or non-host predator olfactory cues in the water (i.e., bird feces and fish mucus, respectively). In lagoon ecosystems of southern France, hundreds to thousands aquatic birds frequently aggregate in rest areas (Cramp and Simmons, 1983 and personal observations). As a result of this high density, fecal concentrations are high in these areas compared to other localities. For infected gammarids living in the surface microhabitat, as well as for uninfected ones which live few centimeters above the mud, the choice is given between aggregating or conversely avoiding areas yet occupied by conspecifics. Starting from this postulate and taking into account the manipulation hypothesis, we predicted that infected gammarids should show increased activity levels and reduced aggregation behavior compared to uninfected gammarids in the presence of bird (definitive host) feces. Also, if aggregation behavior of amphipods can override their propensity to swim to the surface, it could be adaptive for the parasite to manipulate the aggregation behavior of its host. Conversely, in the presence of fish cues, we predicted that there would be a reduction in the activity level and increased aggregation for both infected and uninfected individuals.
Materials and Methods
Gammarid Sampling
From January to May 2012, G. insensibilis were randomly collected following the methodology described in Thomas et al. (1996) from the brackish lagoon of Thau (southern France; 43°25′N, 3°35′E). Infected individuals were identified in the field through the aberrant surface behavior induced by M. papillorobustus (Helluy, 1981; Ponton et al., 2005). From our long field experience (20 years), we know that all gammarids collected at the surface with an aberrant behavior harbor at least one mature cyst of M. papillorobustus, while those collected at the bottom are either non-parasitized or infected by immature metacercariae. In any case, by dissecting all the gammarids at the end of the experiment (see below), we can verify their parasitological status. Because experiments were performed from naturally and not experimentally parasitized individuals, we cannot, in theory, exclude the possibility that aberrant surfacing behaviors are the cause rather than the consequence of the infection. However, previous studies (e.g., Helluy, 1981) clearly demonstrated that the behavioral changes displayed by G. insensibilis harboring metacercariae of M. papillorobustus are induced by the parasite. Infected and uninfected gammarids were placed separately in tanks (30 × 20 × 20 cm) filled with lagoon water; tanks were continuously oxygenated with a bubbler pump (ambient temperature: 20°C) and dwarf eelgrass (Zostera noltii) was added as a food source. In the laboratory, amphipods were maintained (1–7 days) under natural photoperiod conditions (14 h Light: 10 h Darkness). To ensure that observations were not biased by mate choice (Thomas et al., 1996), and because female behavior may vary depending on their reproductive state, only males (distinguished by sexual dimorphism) were used in the experiments.
Odor Clue Sampling
Aquatic bird fecal matter served as the definitive host stimulus. Aquatic bird fecal matter was collected with swab from the Thau's lagoon embankments adjacent to the gammarid sampling areas. This area is almost exclusively frequented by various aquatic birds (e.g., Larus michahellis, Larus ridibundus, Actitis hypoleucos), all of which are potential definitive hosts of the parasite (Alvarez et al., 2006).
Fish conditioned water from the predatory fish Dicentrarchus labrax (about 30 cm) served as the non-definitive host stimulus. Fish-conditioned water was directly sampled in the rearing tank of the Station Méditerranéenne de l'Environnement Littoral to Sète. The sample water was performed the same day as the experiment.
Experimental Design
We set out to determine whether the activity level and aggregation behavior of G. insensibilis were altered by M. papillorobustus in the presence of host-predator cues (aquatic bird feces), non-host predator cues (fish mucus), and lagoon water (control treatment). To do so, we used the experimental set-up described in Thünken et al. (2010). Using a permanent marker, we divided test aquaria (30 × 20 × 20 cm) into three equal compartments (10 × 20 × 20 cm) (see Figure 1). Tea balls (3.5 cm spheres made of fine metal mesh) were hung in the center of the left and right compartments of the test aquaria, 1 cm above the bottom. The central compartment was left empty. The two outer compartments containing the tea balls served as aggregation zones and the middle compartment served as a neutral zone. Since G. insensiblis is active during the day (Helluy, 1981), all experiments were performed between 09:00 and 15:00 (taking into account that amphipod's behavior is significantly similar throughout the day) at ambient temperature (approximately 20°C) and in daylight. For all experiments, aquaria were initially filled with 4.5 L of filtered lagoon water. After each experiment, aquaria were rinsed with lagoon water.
For each of the three conditions tested in parallel (bird feces, fish mucus, or lagoon water only), 10 uninfected male gammarids were randomly selected and placed into one of the tea balls. The second tea ball remained empty. The position of the tea balls (with gammarids vs. without) in the aquaria was alternated between experiments. The 118 gammarids studied were used only once. Ten minutes after the tea balls were introduced, a randomly selected male gammarid was introduced into the aquarium. Following a 30 s acclimatization period, the position of the individual within the tank was recorded every second for a period of 10 min. By recording its location in the aquarium, we were able to ascertain its (i) aggregation behavior (total time spent in close contact with the conspecific group found within the tea ball) and (ii) its activity level (number of times that it left a compartment). The tank was rinsed with lagoon water after each test. In our experiment, we assume that the chemical signals produced by gammarids in the tea ball did not change during the course of the experiment.
Dissection
Following each experiment, gammarids (including those in the tea ball) were dissected to determine parasite load. Each individual was placed in a petri dish containing lagoon water. Under a dissecting microscope, the fourth coxal plate (right or left) was carefully removed using tweezers and measured using an ocular micrometer (2 × magnification). The head was removed with a scalpel to count cerebral metacercariae of M. papillorobustus (Rebecq, 1964; Helluy, 1983).
Statistical Analyses
Activity level was measured by the number of passages from one compartment to another one. This number was log-transformed. Taking into account the manipulation hypothesis, we predicted that infected gammarids should show increased activity levels compared to uninfected gammarids in the presence of bird (definitive host) feces. Standard linear regression was used to study the effects of infection status (IS) and water treatment (WT) and their interaction on the activity level.
Aggregation was measured as the proportion of time spent in the compartment with the tea ball containing gammarids. Uninfected amphipods are predicted to increase their aggregation in the presence of predator cues. On the contrary, infected amphipods are predicted to reduce aggregation behavior in the presence of bird (definitive host) feces. Then, a significant effect of the interaction term between IS and WT is expected. To study the effect of IS, WT and their interaction, we used Beta regression (Ferrari and Cribari-Neto, 2004).
For both models, the significance of each term in the model was assessed via likelihood ratio tests, and partial Wald tests were used to test the nullity of each estimated parameter. Post-hoc analysis was performed using single-step adjustment of P-values.
All computations were carried on with the R software (R Core Team, 2015) and especially the betareg package (Cribari-Neto and Zeileis, 2010) and the multcomp package (Hothorn et al., 2008).
Results
Activity
Log-likelihood tests showed that IS (P = 0.0084) and the interaction IS*WT (P = 0.00016) were significantly associated to the activity level of gammarids.
In Table 1A, parameters which were estimated using the log-transformed data, have been transformed back to activity scale. They are to be interpreted as ratios of activity levels, or relative risk (RR) for a gammarid of being active at a given instant.
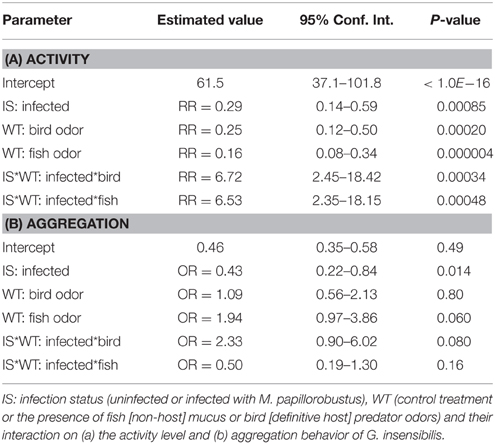
Table 1. Effects of explanatory variables on activity level (relative risks) and aggregation behavior (odds ratios).
There was a significant decrease in the activity level of uninfected individuals in the presence of predator cues compared to control treatment (bird feces, RR = 0.25, P = 0.0013; fish mucus, RR = 0.16, P < 0.001). Infected individuals showed a slight but not significant increase in activity level in the presence of the definitive host cue (RR = 1.68, P = 0.62) and in the presence of fish mucus (RR = 1.04, P = 0.999). In control treatment there was a significant difference in activity level between uninfected and infected gammarids (RR = 0.29, P = 0.0055), with infected individuals being less active than uninfected ones (Figure 2). This result was reversed in the presence of predator cues, i.e., infected individuals were more active than uninfected ones although these differences were not significant (bird feces, RR = 1.95, P = 0.34; fish mucus, RR = 1.89, P = 0.42).
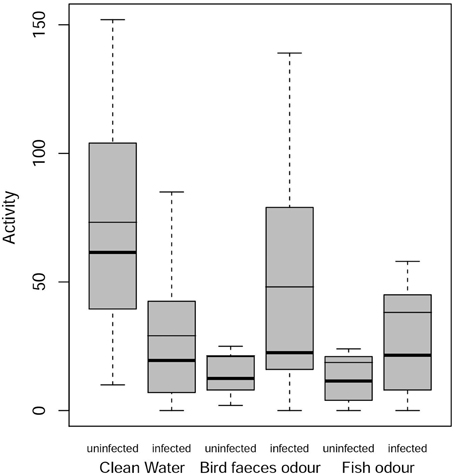
Figure 2. Activity levels of infected and uninfected G. insensibilis. Activity levels (number of times that it left a compartment) as a function of gammarid infection status (uninfected or infected with M. papillorobustus) and water condition (control or the presence of fish [non-host] or bird [definitive host] predator odors). Boxes extend from the 1st to the 3rd quartiles, whiskers extend from the boxes 1.5 times the interquartile range, dots represent extreme data, bold lines inside the boxes correspond to the median, slim lines to the mean. Notice that in the third box, the mean is equal to the third quartile.
Aggregation
As in the activity analysis, likelihood ratio tests showed that infectious status (P = 0.00027) and the interaction IS*WT (P = 0.0085) were significantly associated to the aggregation behavior of gammarids.
In Table 1B, exponentials of the estimated parameters have been reported, and they are to be interpreted as odds-ratios.
In this analysis, the only contrast significantly different from 1 is the difference between uninfected and infected gammarids, with uninfected individuals being overall more aggregated than infected ones (Figure 3). In the presence of definitive host cues, infected and uninfected individuals displayed similar level of aggregation (OR = 1.002, P = 1.0).
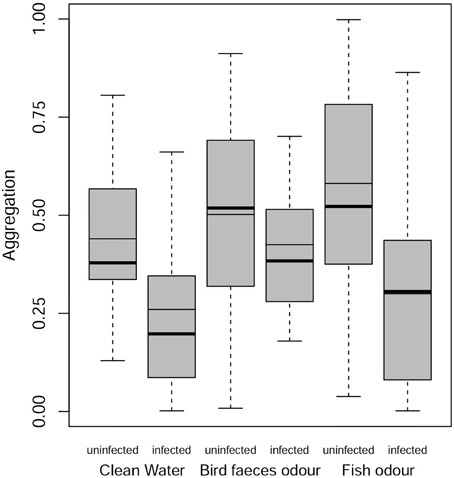
Figure 3. Aggregation behavior of infected and uninfected G. insensibilis. Aggregation behavior (total time spent in close contact with the conspecific group found within the tea ball) as a function of the gammarid infection status (uninfected or infected with M. papillorobustus) and water condition (control or the presence of fish [non-host] or bird [definitive host] predator odors). The meaning of the graphical elements is the same as in Figure 2. Notice that in the last box, the mean is equal to the median.
Overall, the aggregation behavior of uninfected individuals was not altered by the presence of predatory cues (bird feces: OR = 1.09, P = 0.99; fish mucus: OR = 1.94, P = 0.29). In contrast, infected individuals showed an increased aggregation behavior in the presence of definitive host cues compared to the control treatment (OR = 2.54, P = 0.041). No difference in aggregation behavior was observed in presence of fish mucus (OR = 0.97, P = 1.0).
Discussion
As recalled by Poulin (2010), no one expects a sick animal to behave normally and the simplest, most parsimonious, explanation for a difference in behavior between parasitized and non-parasitized animals need only involve side-effects of pathology (Poulin, 1995; Thomas et al., 2005). However, if a parasite-induced change in host behavior leads to improved transmission of the parasite, then, as long as there is a genetic basis for this effect, and whether it is fortuitous or not, it can be considered as a case of adaptive manipulation (Poulin, 2010). Applying these considerations in the context of multidimensional manipulations is currently a challenging task for which speculation until now has proven more attractive than data collection.
Activity Level
Several invertebrate species, particularly amphipods, have evolved the ability to reduce activity levels and increase their use of refuges once they have detected a predator's presence by chemical means (Andersson et al., 1986; Wooster, 1998; Dezfuli et al., 2003; Wellnitz et al., 2003; Bollache et al., 2006; Perrot-Minnot et al., 2007; Thünken et al., 2010). In agreement with these findings, we found that uninfected individuals were significantly less active in the presence of bird and fish cues than in control treatment. This suggests that activity level is plastic in G. insensibilis and can be adjusted in response to the presence of olfactory cues associated with predation risk (Médoc and Beisel, 2009; Lewis et al., 2012). Therefore, this behavior constitutes a potential target for natural selection acting on parasites to increase trophic transmission to the final host predators.
Conversely to uninfected individuals that reduced their activity in the presence of predator cues, the difference was not significant for the infected individuals. Infected amphipods tended to have higher activity levels than uninfected individuals in the bird feces treatment, while the reverse was observed in the control treatment. These results could support the manipulation hypothesis since active gammarids are presumably more likely to be eaten by definitive hosts (birds) than passive individuals. Alternatively, these findings could also indicate that parasitized gammarids do not anymore respond to the predatory cues, without the need to invoke an active manipulation. Further studies would also be needed to clarify the links that exist between activity, geotaxis and phototaxis. Indeed, we cannot exclude either that alteration in the activity level could be a by-product of infected amphipods' tendency to swim toward the surface.
Aggregation Behavior
Assuming that aggregation is an anti-predator behavior (Krause and Godin, 1994; Mooring and Hart, 2008), we predicted that uninfected individuals would display a higher aggregation in response to predators (both bird and fish), while infected ones would display this protective behavior only when confronted with non-host predators (i.e., fish only). Contrary to these predictions, uninfected individuals did not significantly increase their aggregation behavior in the presence of either bird or fish cues. These results contrast with those of Kullmann et al. (Kullmann et al., 2008), obtained using the same protocol, but on a different species. This suggests that increased aggregation in response to predation risk may not be a general phenomenon among gammarid species. One possible explanation for this result is that in our system the risk of bird predation is not associated with the presence of bird feces at the bottom of the water column where uninfected individuals spend most of their time (Helluy, 1983). It is also possible that for uninfected gammarids, which are yet aggregated naturally, increasing aggregation further only marginally decrease predation risk, so that it is not optimal for uninfected amphipods to increase it.
Contrary to our predictions, infected gammarids did not decrease, instead increase, their aggregation behavior when exposed to predatory final host cues. It is possible that the transmission benefits for the parasite remain low relative to the costs associated with inducing an olfactory manipulation (Thomas et al., 2010). In addition, this cost increased if there was partial resistance from the host. Indeed, Daoust et al. (2015) proposed that natural selection could, under certain circumstances, favor hosts that resist to certain manipulative dimensions, especially those that contribute least to parasite transmission. In so doing, parasitised hosts could substantially postpone their death by predation and increase their mating opportunities without severely impairing the chance of parasite transmission. Natural selection on the host could favor partial resistance to manipulation because it allows reproduction. On the contrary, depending on the costs/benefits for the parasite transmission to keeping these dimensions, selection could conversely be low to prevent the host from displaying partial resistance to manipulation. In this experiment, infected gammarids would respond to the manipulation exerted by M. papillorobustus by displaying additional behaviors (in this case aggregation) that limit their predation risk by aquatic birds, the definitive host. Alternatively, we cannot exclude the possibility that aggregation at the water surface (coupled with higher activity; see previous paragraph) instead of conferring protection enhances predation risk, perhaps by attracting more predators (Rode et al., 2013). In presence of fish cues infected individuals do not increase their aggregation. This result was surprising given that M. papillorobustus should presumably benefit from an increased aggregation in response to predation risk by inappropriate definitive hosts. One possible explanation is that infected amphipods are less responsive to non-host predator cues as previously suggested for other gammarid species (e.g., Baldauf et al., 2007; Perrot-Minnot et al., 2007; Lewis et al., 2012). However, this would not necessarily be detrimental for parasite fitness given that fish are not frequent to the water surface. Alternatively, if we consider that aggregation behaviors attract, rather than protect from predators (Milinski, 1977), this decreased aggregation might be adaptive for the parasite. Finally, it remains possible that infected amphipods were generally less aggregated than uninfected ones because they were simply not paying attention to the group and were just trying to reach the water surface due to manipulation on geotaxis and phototaxis.
Conclusion
It is interesting to note that activity and aggregation behaviors of infected and uninfected individuals were different in the control treatment, with infected gammarids being less active and less aggregated than uninfected individuals. One possible explanation for this finding is that the energy required by the host to accomplish a parasite-induced behavior such as the maintenance of aberrant surfacing behaviors represents a key energetic constraint (Maure et al., 2013). Manipulative parasites therefore have to “budget” for such costs when optimizing the exploitation of host resources as they develop (Thomas et al., 2005; Lefèvre et al., 2008; Poulin, 2010). Following the theoretical model of Parker et al. (2009), a parasite's strategy that induces a switch from “predation suppression” (during non-infective phase) to “predation enhancement” (as soon as the infective stage is reached) in the intermediate host is selectively advantageous.
Among the limitations in our study, it remains difficult to disentangle in our experimental apparatus the effect of manipulation on phototaxis/geotaxis and manipulation on other traits, such as aggregation and activity. Indeed, the first manipulations bring the parasitized gammarid in a habitat where ecological conditions, and hence decisions to take, are potentially different. Differences may be adaptive responses from the host which accommodates with the novelty, not necessarily adaptations for the parasitic transmission (see for instance Poulin and Thomas, 1999). Different aggregation/activity levels could also be by products of the first manipulations with no adaptive value. Said differently, traits studied here, even though they can be different between unparasitized and parasitized individuals, would not be under parasite manipulation. Beyond this study, this suggests that evaluating fitness benefits for the parasite of different changes in manipulated organisms is a difficult task because any change is likely to subsequently generate by-products.
This study suggests that even in well-known models of host manipulation (the system of G. insensibilis—M. papillorobustus has been studied since the 1980s), multidimensional aspects of manipulation deserve to be further investigated. Additional studies, especially ecological and mechanistic ones, are necessary to improve our understanding of the conditions that govern multidimensionality in manipulative strategies.
Author Contributions
AD, MS, MB, EE, HD, DM, MV, SD, and FT contributed to the design of this work and the acquisition of data. AA, MV, EE, SD, and FT were involved in the drafting of the article. All the co-authors revised the content of the paper and approved this version for publication.
Conflict of Interest Statement
The authors declare that the research was conducted in the absence of any commercial or financial relationships that could be construed as a potential conflict of interest.
Acknowledgments
We thank the Station Méditerranéenne de l'Environnement Littoral to Sète (France) and especially François Bonhomme. We also thank the reviewers for their helpful comments.
References
Alvarez, M. F., Cordeiro, J. A., Leiro, J. M., and Sanmartin, M. L. (2006). Influence of host age and sex on the helminth fauna of the yellow-legged gull (Larus michahellis) in Galicia (northwestern Spain). J. Parasitol. 92, 454–458. doi: 10.1645/GE-3546.1
Andersson, K. G., Bronmark, C., Herrmann, J., Malmqvist, B., Otto, C., and Sjorstrom, P. (1986). Presence of sculpins (Cottus-Gobio) reduces drift and activity of gammarus-pulex (Amphipoda). Hydrobiologia 133, 209–215. doi: 10.1007/BF00005592
Baldauf, S. A., Thünken, T., Frommen, J. G., Bakker, T. C. M., Heupel, O., and Kullmann, H. (2007). Infection with an acanthocephalan manipulates an amphipod's reaction to a fish predator's odours. Int. J. Parasitol. 37, 61–65. doi: 10.1016/j.ijpara.2006.09.003
Benesh, D. P., Valtonen, E. T., and Seppälä, O. (2008). Multidimensionality and intra-individual variation in host manipulation by an acanthocephalan. Parasitology 135, 617–626. doi: 10.1017/S0031182008004216
Bollache, L., Kaldonski, N., Troussard, J. P., Lagrue, C., and Rigaud, T. (2006). Spines and behaviour as defences against fish predators in an invasive freshwater amphipod. Anim. Behav. 72, 627–633. doi: 10.1016/j.anbehav.2005.11.020
Cézilly, F., Favrat, A., and Perrot-Minnot, M.-J. (2013). Multidimensionality in parasite-induced phenotypic alterations: ultimate versus proximate aspects. J. Exp. Biol. 216, 27–35. doi: 10.1242/jeb.074005
Cézilly, F., and Perrot-Minnot, M. J. (2005). Studying adaptive changes in the behaviour of infected hosts: a long and winding road. Behav. Processes 68, 223–228. doi: 10.1016/j.beproc.2004.08.013
Cézilly, F., and Perrot-Minnot, M. J. (2010). Interpreting multidimensionality in parasite-induced phenotypic alterations: panselectionism versus parsimony. Oikos 119, 1224–1229. doi: 10.1111/j.1600-0706.2010.18579.x
Cramp, S., and Simmons, K. E. L. (1983). “The birds of the Western Palearctic,” in Handbook of the Birds of Europe, The Middle East and North Africa, 1st Edn. (New York, NY: Oxford University Press), 130–150.
Cribari-Neto, F., and Zeileis, A. (2010). Beta Regression in R. J. Stat. Soft. 34, 1–24. Available online at: http://www.jstatsoft.org/v34/i02/paper
Daoust, S. P., King, K. C., Roche, B., and Thomas, F. (2015). Making the best of a bad situation: host partial resistance and by pass of behavioral manipulation by parasites? Trends Parasitol. 31, 413–418. doi: 10.1016/j.pt.2015.05.007
Dezfuli, B. S., Maynard, B. J., and Wellnitz, T. A. (2003). Activity levels and predator detection by amphipods infected with an acanthocephalan parasite, Pomphorhynchus laevis. Folia Parasitol. (Praha) 50, 129–134. doi: 10.14411/fp.2003.023
Dianne, L., Perrot-Minnot, M. J., Bauer, A., Gaillard, M., Léger, E., and Rigaud, T. (2011). Protection first then facilitation: a manipulative parasite modulates the vulnerability to predation of its intermediate host according to its own developmental stage. Evolution 65, 2692–2698. doi: 10.1111/j.1558-5646.2011.01330.x
Ferrari, S. L. P., and Cribari-Neto, F. (2004). Beta regression for modelling rates and proportions. J. Appl. Stat. 31, 799–815. doi: 10.1080/0266476042000214501
Helluy, S. (1981). Parasitisme et comportement. Etude de la Métacercaire de Microphallus papillorobustus (Rankin 1940) et de son influence sur les gammares. Ph.D. thesis, U.S.T.L. Montpellier.
Helluy, S. (1983). Relations hôtes-parasite du trématode Microphallus papillorobustus (Rankin, 1940). II. Modifications du comportement des Gammarus hôtes intermédiaires et localisation des métacercaires. Ann. Parasitol. Hum. Comparée 58, 1–17.
Helluy, S. (1984). Relations hôtes-parasites du trématode Microphallus papillorobustus (Rankin 1940). III. Facteurs impliqués dans les modifications du comportement des Gammarus hôtes intermediaires et tests de prédation. Ann. Parasitol. Hum. Comparée 59, 41–56.
Hothorn, T., Bretz, F., and Westfall, P. (2008). Simultaneous inference in general parametric models. Biom. J. 50, 346–363. doi: 10.1002/bimj.200810425
Hughes, D. P., Brodeur, J., and Thomas, F. (2012). Host Manipulation by Parasites. New York, NY: Oxford University Press.
Kaldonski, N., Perrot-Minnot, M. J., and Cézilly, F. (2007). Differential influence of two acanthocephalan parasites on the antipredator behaviour of their common intermediate host. Anim. Behav. 74, 1311–1317. doi: 10.1016/j.anbehav.2007.02.027
Krause, J., and Godin, J.-G. J. (1994). Shoal choice in the banded killifish (Fundulus diaphanus, Teleostei, Cyprinodontidae): effectsofpredation risk, fishsize species composition and size of shoals. Ethology 98, 128–136. doi: 10.1111/j.1439-0310.1994.tb01063.x
Kullmann, H., Thünken, T., Baldauf, S. A., Bakker, T. C. M., and Frommen, J. G. (2008). Fish odour triggers conspecific attraction behaviour in an aquatic invertebrate. Biol. Lett. 4, 458–460. doi: 10.1098/rsbl.2008.0246
Lefèvre, T., Roche, B., Poulin, R., Hurd, H., Renaud, F., and Thomas, F. (2008). Exploiting host compensatory responses: the ‘must’ of manipulation? Trends Parasitol. 24, 435–439. doi: 10.1016/j.pt.2008.06.006
Levri, E. P. (1998). The influence of non-host predators on parasite-induced behavioural changes in a freshwater snail. Oikos 119, 393–400.
Lewis, S. E., Hodel, A., Sturdy, T., Todd, R., and Weigl, C. (2012). Impact of acanthocephalan parasites on aggregation behavior of amphipods (Gammarus pseudolimnaeus). Behav. Processes 91, 159–163. doi: 10.1016/j.beproc.2012.07.009
Maure, F., Brodeur, J., Hughes, D., and Thomas, F. (2013). How much energy should manipulative parasites leave to their hosts to ensure altered behaviours? J. Exp. Biol. 216, 43–46. doi: 10.1242/jeb.073163
Medoc, V., and Beisel, J.-N. (2008). An acanthocephalan parasite boosts the escape performance of its intermediate host facing non-host predators. Parasitology 135, 977–984. doi: 10.1017/S0031182008004447
Médoc, V., and Beisel, J. N. (2009). Field evidence for non-host predator avoidance in a manipulated amphipod. Naturwissenschaften 96, 513–523. doi: 10.1007/s00114-008-0503-8
Milinski, M. (1977). Experiments on the selection by predators against spatial oddity of their prey. Z. Tierpsychol. 43, 311–325. doi: 10.1111/j.1439-0310.1977.tb00078.x
Mooring, M., and Hart, B. (2008). Animal grouping for protection from parasites: selfish herd and encounter-dilution effects. Behaviour 123, 173–193. doi: 10.1163/156853992X00011
Parker, G. A., Ball, M. A., Chubb, J. C., Hammerschmidt, K., and Milinski, M. (2009). When should a trophically transmitted parasite manipulate its host? Evolution 63, 448–458. doi: 10.1111/j.1558-5646.2008.00565.x
Perrot-Minnot, M. J., Kaldonski, N., and Cézilly, F. (2007). Increased susceptibility to predation and altered anti-predator behaviour in an acanthocephalan-infected amphipod. Int. J. Parasitol. 37, 645–651. doi: 10.1016/j.ijpara.2006.12.005
Ponton, F., Biron, D., Joly, C., Duneau, D., and Thomas, F. (2005). Ecology of populations parasitically modified: a case study from a gammarid (Gammarus insensibilis)- trematode (Micrrophalus papillorobustus) system. Mar. Ecol. Prog. Ser. 299, 205–215. doi: 10.3354/meps299205
Poulin, R. (1995). “Adaptive” change in the behaviour of parasitized animals: a critical review. Int. J. Parasitol. 25, 1371–1383 doi: 10.1016/0020-7519(95)00100-x
Poulin, R. (2010). Parasite manipulation of host behavior: an update and frequently asked questions. Adv. Study Behav. 41, 151–186. doi: 10.1016/S0065-3454(10)41005-0
Poulin, R., and Thomas, F. (1999). Phenotypic variability induced by parasites: extent and evolutionary implications. Parasitol. Today 15, 28–32. doi: 10.1016/S.0169-4758(98)01357-X
R Core Team (2015). R: A Language and Environment for Statistical Computing. Vienna: R Foundation for Statistical Computing. Available online at: http://www.R-project.org/
Rebecq, J. (1964). Recherches Systématiques, Biologiques et Écologiques sur les Formes Larvaires de Quelques Trématodes de Camargue. Ph.D. thesis, University of Marseille, Marseille.
Rode, N. O., Lievens, E. J. P., Flaven, E., Segard, A., Jabbour-Zahab, R., Sanchez, M. I., et al. (2013). Why join groups? Lessons from parasite-manipulated Artemia. Ecol. Lett. 16, 493–501. doi: 10.1111/ele.12074
Thomas, F., Adamo, S., and Moore, J. (2005). Parasitic manipulation: where are we and where should we go? Behav. Processes 68, 185–199. doi: 10.1016/j.beproc.2004.06.010
Thomas, F., Poulin, R., and Brodeur, J. (2010). Host manipulation by parasites: a multidimensional phenomenon. Oikos 119, 1217–1223. doi: 10.1111/j.1600-0706.2009.18077.x
Thomas, F., Renaud, F., and Cezilly, F. (1996). Assortative pairing by parasitic prevalence in Gammarus insensibilis (Amphipoda): patterns and processes. Anim. Behav. 52, 683–690. doi: 10.1006/anbe.1996.0213
Thünken, T., Baldauf, S. A., Bersau, N., Bakker, T. C. M., Kullmann, H., and Frommen, J. G. (2010). Impact of olfactory non-host predator cues on aggregation behaviour and activity in Polymorphus minutus infected Gammarus pulex. Hydrobiologia 654, 137–145. doi: 10.1007/s10750-010-0377-6
Wellnitz, T., Giari, L., Maynard, B., and Dezfuli, B. S. (2003). A parasite spatially structures its host population. Oikos 100, 263–268. doi: 10.1034/j.1600-0706.2003.12153.x
Wooster, D. E. (1998). Amphipod (Gammarus minus) responses to predators and predator impact on amphipod density. Oecologia 115, 253–259. doi: 10.1007/s004420050514
Keywords: activity level, aggregation behavior, Gammarus insensibilis, Microphallus papillorobustus, Parasitic manipulation
Citation: Arnal A, Droit A, Elguero E, Ducasse H, Sánchez MI, Lefevre T, Misse D, Bédèrina M, Vittecoq M, Daoust S and Thomas F (2015) Activity level and aggregation behavior in the crustacean gammarid Gammarus insensibilis parasitized by the manipulative trematode Microphallus papillorobustus. Front. Ecol. Evol. 3:109. doi: 10.3389/fevo.2015.00109
Received: 27 May 2015; Accepted: 08 September 2015;
Published: 29 September 2015.
Edited by:
Oliver Yves Martin, ETH Zurich, SwitzerlandReviewed by:
Bronwyn Heather Bleakley, Stonehill College, USAMatthias Galipaud, Bielefeld University, Germany
Copyright © 2015 Arnal, Droit, Elguero, Ducasse, Sánchez, Lefevre, Misse, Bédèrina, Vittecoq, Daoust and Thomas. This is an open-access article distributed under the terms of the Creative Commons Attribution License (CC BY). The use, distribution or reproduction in other forums is permitted, provided the original author(s) or licensor are credited and that the original publication in this journal is cited, in accordance with accepted academic practice. No use, distribution or reproduction is permitted which does not comply with these terms.
*Correspondence: Frédéric Thomas, Maladies Infectieuses et Vecteurs: Ecologie, Génétique, Evolution et Contrôle, UMR (IRD/CNRS/UM) 5290, 911 Avenue Agropolis, BP 64501, 34394 Montpellier, France, frederic.thomas2@ird.fr
†These authors have contributed equally to this work.