- 1 Department of Immunology, Weizmann Institute of Science, Rehovot, Israel
- 2 Department of Neurobiology, Weizmann Institute of Science, Rehovot, Israel
The brain has been commonly regarded as a “tissue behind walls.” Appearance of immune cells in the brain has been taken as a sign of pathology. Moreover, since infiltrating monocyte-derived macrophages and activated resident microglia were indistinguishable by conventional means, both populations were considered together as inflammatory cells that should be mitigated. Yet, because the microglia permanently reside in the brain, attributing to them negative properties evoked an ongoing debate; why cells that are supposed to be the brain guardians acquire only destructive potential? Studies over the last two decades in the immune arena in general, and in the context of central nervous system pathology in particular, have resulted in a paradigm shift toward a more balanced appreciation of the contributions of immune cells in the context of brain maintenance and repair, and toward the recognition of distinct roles of resident microglia and infiltrating monocyte-derived macrophages.
Differential Mononuclear Phagocyte Origins
The mononuclear phagocyte system – a term coined by van Furth et al. (1972) – is known today to comprise monocytes, dendritic cells (DCs), and macrophages, as well as their respective committed bone marrow (BM) progenitors. Mononuclear phagocytes can arise via two exclusive developmental routes (Orkin and Zon, 2008). Most peripheral mononuclear phagocytes are descended from BM-derived cells formed as part of ongoing definitive hematopoiesis. A major breakthrough in defining this developmental pathway was the identification of a clonotypic BM-resident founder cell, termed the macrophage-DC precursor (MDP), that can give rise to peripheral mononuclear phagocytes while having lost granulocyte potential (Fogg et al., 2006). MDPs differentiate within the BM into monocytes (Varol et al., 2007) and into dedicated DC precursors, the pre-DCs, that exit to the circulation to enable the repopulation of peripheral tissue macrophages and DCs, respectively. As opposed to the ephemeral DCs, peripheral macrophage populations are however heterogeneous with respect to their turnover rate, and thus, in their steady state, rely to a varying degree on renewal by BM-derived precursors, e.g., monocyte input (Landsman et al., 2007; Varol et al., 2007). Under inflammatory conditions, most resident microglia are complemented by recruited monocytes that differentiate in situ into macrophages (Ajami et al., 2011). However, the contribution of these cells is often transient (Ajami et al., 2011). Moreover, in certain scenarios, such as parasite-driven Th2 inflammation, tissue-resident macrophage populations were recently shown to even expand locally without any monocyte influx (Jenkins et al., 2011).
As exemplified by the brain microglia (Alliot et al., 1999; Ginhoux et al., 2010), mononuclear phagocytes can also arise during early development (around embryonic day 7.5) as a result of primitive hematopoiesis occurring in extra-embryonic tissue (Orkin and Zon, 2008). After seeding the tissue that is their final destination, these primitive macrophage-derived cells can – as is the case for the secluded microglia – maintain themselves through longevity coupled with limited self-renewal. Thus, these compartments remain independent of monocytic input throughout adult life (Ajami et al., 2007; Mildner et al., 2007; Shechter et al., 2009; Ginhoux et al., 2010). This alternative pathway might encode functional specialization. However, to what extent it applies to macrophage populations other than the brain microglia, remains currently unknown and will have to await future fate mapping studies.
The Mystery of the Role of Monocyte-Derived Macrophages Following Sterile CNS Injury
Extensive comparison of the responses of the peripheral and central nervous system (CNS) to axonal injury has revealed that recruitment of blood-derived cells to injured peripheral nerves is more pronounced than to the CNS (Perry et al., 1993). Opinions differed, however, regarding the interpretation of this finding (Rapalino et al., 1998; Schwartz et al., 1999; Shechter et al., 2009; London et al., 2011). Insight into “sterile” (non-infectious) injuries outside the CNS (DiPietro, 1995) and the assumption that recruited cells may be beneficial though their numbers might be insufficient for optimal repair, let to the suggestion that introduction of blood-derived macrophages with the correct phenotype, at the right time, and to the correct location following CNS injury could promote repair. Indeed, in a model of spinal cord injury, it was demonstrated that “alternatively activated” blood macrophages, when locally transplanted at the margin of a spinal cord lesion, resulted in improved recovery (Rapalino et al., 1998). The success of such macrophage transplantation was found to be dependent on the site of their injection (no effect was found when cells were administered at the center of the lesion or far from its margins), the number of injected cells, and the time elapsed between the injury and the injection (Schwartz and Yoles, 2006). The results of these manipulations were initially puzzling, primarily due to the lack of appreciation of macrophage heterogeneity (Gordon, 2003; Gordon and Taylor, 2005) by neuroscientists (Popovich et al., 1999; Mabon et al., 2000). Since the site of injury becomes spontaneously overwhelmed with locally activated microglia, the idea of deriving therapeutic benefit by adding additional blood-derived macrophages seemed contrary to common logic. Subsequent studies from several laboratories regarding the roles of various macrophage populations further complicated the picture, as the researchers primarily studied macrophages activated in vitro using products of bacteria or yeast, assuming that such inflammatory cells mimic strongly activated macrophages. However, activating agents differ not only in their strength of activation, but also in the functional state that they induce; thus, macrophages activated by yeast or bacterial cell wall components, such as zymosan or LPS, do not necessarily represent cells activated following “sterile” injury.
Additional missing pieces in the puzzle of the role of macrophages following CNS injury were the realization that distinct immune activities might be needed following the insult for tissue rescue/protection (Shechter et al., 2009) and restoration/regeneration (Benowitz and Yin, 2010; Benowitz and Popovich, 2011), and that although activated microglia and monocyte-derived macrophages share morphology and phenotypes, they might display distinct activities at critical time points following the insult (Shechter et al., 2009). These facts were belatedly accepted when standard histological or immuno-histochemical techniques of limited resolution were complemented by new genetic approaches enabling the tracking of cell origins and fates.
Differential Contributions of Activated Resident Microglia and Infiltrating Monocyte-Derived Macrophages to the Recovery from Acute Spinal Cord Injury
As outlined above, it is becoming clear that the resident microglia compartment is derived from primitive macrophages that enter the CNS during embryonic development; this compartment remains independent of monocytic input throughout life (Figure 1). The use of chimeric mice, in which monocytes, but not the microglia express a green fluorescent protein, GFP (CX3CR1gfp > wild type chimeras) allowed us to define the origins of the innate immune cell populations at a site of spinal cord injury. The chimeric mice were prepared by total body irradiation excluding the head, since although microglial cells are radio-resistant and remain strictly host-derived in BM chimeras, cells of donor marrow origin have been shown to enter irradiated brains but not of head protected brain. In the absence of injury, blood-derived macrophages were found to be excluded from the healthy host brain, as long as the chimeras were created while shielding the head during the conditioning irradiation. Following spinal cord injury, blood-derived GFP+ myeloid cells were found at the lesion site; yet, the majority of these recruited cells arrived with a delay relative to the initial injury-associated breach of the blood–brain barrier. Secondly and most critically, conditional ablation of the recruited blood-derived macrophages, by virtue of their expression of CD11c and use of the CD11c-DTR system (Jung et al., 2002), worsened the functional motor score of the injured animals and extended the lesion size, while elevating the inflammatory response of the local microglia (Shechter et al., 2009). Thus, the blood-derived macrophages, are recruited to the site of the injury only following insult, and facilitate the termination of the local immune response by displaying an anti-inflammatory activity required for the regulation of the locally activated microglia. Recovery from CNS insults hence seems to comprise consecutive discrete phases, as proposed for peripheral wound repair (Arnold et al., 2007; Nahrendorf et al., 2007). Accordingly, a first response primarily involves resident microglia and possibly also some infiltrating immune cells that “clean” the site of injury, removing dead cells, and debris. This phase is then followed by an active process of immune termination that involves resolving monocyte-derived macrophages, reminiscent of healing of tissues outside the CNS (Gordon and Taylor, 2005; Arnold et al., 2007). Indeed, we found that for blood-derived macrophages to be beneficial at the lesion site, they must express IL-10, the classical anti-inflammatory cytokine known to be associated with alternatively activated macrophages (also known as “M2” or “resolving macrophages”; Shechter et al., 2009). Ablation of the blood-derived macrophages followed by their replacement with macrophages that are defective in IL-10 expression preserves recruitment of cells, but results in impaired overall recovery (Shechter et al., 2009). Similarly, suppressing recruitment of innate immune cells at this stage is counterproductive, and is likely to result in chronic inflammation and insufficient repair (Shechter et al., 2009). Conversely, boosting of monocyte recruitment results in improved recovery (Shechter et al., 2009). Collectively, these results, argue in favor of both microglia and monocyte-derived macrophages as key players in the repair of spinal cord injuries, but with non-redundant activity. The monocyte-derived macrophages display in this scenario a local anti-inflammatory and beneficial role, which is critically dependent upon their expression of interleukin 10.
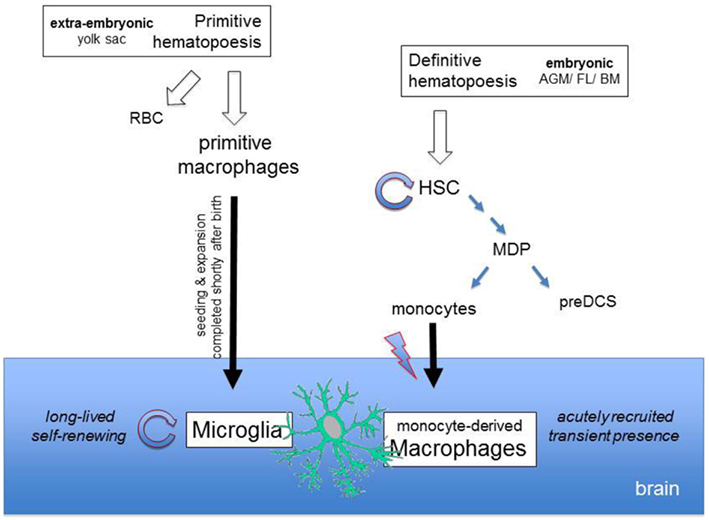
Figure 1. Differential origins of microglia and monocyte-derived brain macrophages. Microglia derive from macrophages that arise from primitive hematopoiesis in the yolk sac, The primitive macrophages seed the developing brain and expand to give rise to the microglia, which subsequently maintains itself through longevity and limited self-renewal. Upon injury, the brain recruits monocytes that differentiate locally into monocyte-derived macrophages and transiently complement the brain mononuclear phagocyte compartment. Monocytes are generated in the BM from macrophage-DC precursors (MDPs) that are constantly replenished from self-renewing hematopoietic stem cells (HSCs) which arose from definitive hematopoiesis.
Differential Contributions of Activated Resident Microglia and Infiltrating Monocyte-Derived Macrophages to Experimentally Induced Autoimmune Encephalitis
Multiple sclerosis and its established mouse model, experimentally induced autoimmune encephalitis (EAE), are characterized by extensive CNS infiltration of both lymphoid and myeloid inflammatory cells (Hickey, 1991). As with the acute injury model, specific contributions or resident microglia and recruited monocyte-derived macrophages have long remained a matter of debate due to the absence of suitable experimental systems. Using a combination of parabiosis and irradiation, Rossi and colleagues recently shed considerable light on this enigma (Ajami et al., 2011). Specifically, their strategy relied on reconstitution of an irradiated parabiont by its shielded parabiosis partner, thereby avoiding transfer of mechanically collected BM, which could contain precursors that are not released into the circulation under physiological conditions. This resulted in efficient replacement of BM and peripheral blood by donor cells, but no myeloid repopulation of the CNS; hence this system enabled monitoring of the dynamics of monocyte-derived macrophages and microglia during the course of active EAE. Interestingly, significant monocyte recruitment via a meningal route was found only in animals that already displayed considerable disease scores (>2). Progression to severe EAE, strongly correlated with the extent of this myelo-monocytic CNS infiltrate. Moreover, corroborating an earlier report (Mildner et al., 2009), the use of CCR2-deficient parabionts established monocyte-derived macrophages as active drivers of CNS inflammation.
The study of Ajami et al. (2011) also allowed a glimpse at the dynamic response of the resident microglia. Activation of microglia, as indicated by BrdU incorporation (a measure of proliferation), was observed prior to monocyte recruitment and irrespective of disease progression. During the acute severe EAE disease phase, the microglial compartment transiently expanded but is trimmed, presumably to its original density.
Collectively, also during setting of autoimmune inflammation, non-redundant activities of microglia and monocyte-derived macrophages emerge. Interestingly, the monocyte-derived cells display in this scenario as opposed to the injury model a pro-inflammatory role.
Concluding Remark
The results summarized above highlight important lessons:
a. That infiltrating monocyte-derived macrophages are not part of the microglia turnover.
b. The functional plasticity of monocyte-derived macrophages, which can adopt opposite fates depending on the context encountered. Thus, while they have a pivotal and beneficial role in sterile injury model, under inflammatory autoimmune disease conditions the recruited monocytes contribute to disease severity.
c. The intriguing and apparently distinct roles of resident microglia, the brain sentinels: on one hand, their essential role in the first phase of recovery from sterile injury, and, on the other hand, their key contribution in the initiation of inflammation autoimmune disease possibly through their interaction with autoreactive CD4+ T cells.
Conflict of Interest Statement
The authors declare that the research was conducted in the absence of any commercial or financial relationships that could be construed as a potential conflict of interest.
Acknowledgments
This work was supported in part by the Deutsche Forschungsgemeinschaft Research Unit (FOR) 1336 (Steffen Jung), and by ERC – European Research Council-Advanced grant (Michal Schwartz).
References
Ajami, B., Bennett, J. L., Krieger, C., McNagny, K. M., and Rossi, F. M. (2011). Infiltrating monocytes trigger EAE progression, but do not contribute to the resident microglia pool. Nat. Neurosci. 14, 1142–1149.
Ajami, B., Bennett, J. L., Krieger, C., Tetzlaff, W., and Rossi, F. M. (2007). Local self-renewal can sustain CNS microglia maintenance and function throughout adult life. Nat. Neurosci. 10, 1538–1543.
Alliot, F., Godin, I., and Pessac, B. (1999). Microglia derive from progenitors, originating from the yolk sac, and which proliferate in the brain. Brain Res. Dev. Brain Res. 117, 145–152.
Arnold, L., Henry, A., Poron, F., Baba-Amer, Y., van Rooijen, N., Plonquet, A., Gherardi, R. K., and Chazaud, B. (2007). Inflammatory monocytes recruited after skeletal muscle injury switch into antiinflammatory macrophages to support myogenesis. J. Exp. Med. 204, 1057–1069.
Benowitz, L. I., and Popovich, P. G. (2011). Inflammation and axon regeneration. Curr. Opin. Neurol. 24, 577–583.
DiPietro, L. A. (1995). Wound healing: the role of the macrophage and other immune cells. Shock 4, 233–240.
Fogg, D. K., Sibon, C., Miled, C., Jung, S., Aucouturier, P., Littman, D. R., Cumano, A., and Geissmann, F. (2006). A clonogenic bone marrow progenitor specific for macrophages and dendritic cells. Science 311, 83–87.
Ginhoux, F., Greter, M., Leboeuf, M., Nandi, S., See, P., Gokhan, S., Mehler, M. F., Conway, S. J., Ng, L. G., Stanley, E. R., Samokhvalov, I. M., and Merad, M. (2010). Fate mapping analysis reveals that adult microglia derive from primitive macrophages. Science 330, 841–845.
Gordon, S., and Taylor, P. R. (2005). Monocyte and macrophage heterogeneity. Nat. Rev. Immunol. 5, 953–964.
Hickey, W. F. (1991). Migration of hematogenous cells through the blood-brain barrier and the initiation of CNS inflammation. Brain Pathol. 1, 97–105.
Jenkins, S. J., Ruckerl, D., Cook, P. C., Jones, L. H., Finkelman, F. D., van Rooijen, N., MacDonald, A. S., and Allen, J. E. (2011). Local macrophage proliferation, rather than recruitment from the blood, is a signature of TH2 inflammation. Science 332, 1284–1288.
Jung, S., Unutmaz, D., Wong, P., Sano, G., De los Santos, K., Sparwasser, T., Wu, S., Vuthoori, S., Ko, K., Zavala, F., Pamer, E. G., Littman, D. R., and Lang, R. A. (2002). In vivo depletion of CD11c(+) dendritic cells abrogates priming of CD8(+) T cells by exogenous cell-associated antigens. Immunity 17, 211–20.
Landsman, L., Varol, C., and Jung, S. (2007). Distinct differentiation potential of blood monocyte subsets in the lung. J. Immunol. 178, 2000–2007.
London, A., Itskovich, E., Benhar, I., Kalchenko, V., Mack, M., Jung, S., and Schwartz, M. (2011). Neuroprotection and progenitor cell renewal in the injured adult murine retina requires healing monocyte-derived macrophages. J. Exp. Med. 208, 23–39.
Mabon, P. J., Weaver, L. C., and Dekaban, G. A. (2000). Inhibition of monocyte/macrophage migration to a spinal cord injury site by an antibody to the integrin alphaD: a potential new anti-inflammatory treatment. Exp. Neurol. 166, 52–64.
Mildner, A., Mack, M., Schmidt, H., Bruck, W., Djukic, M., Zabel, M. D., Hille, A., Priller, J., and Prinz, M. (2009). CCR2+Ly-6Chi monocytes are crucial for the effector phase of autoimmunity in the central nervous system. Brain 132, 2487–2500.
Mildner, A., Schmidt, H., Nitsche, M., Merkler, D., Hanisch, U. K., Mack, M., Heikenwalder, M., Bruck, W., Priller, J., and Prinz, M. (2007). Microglia in the adult brain arise from Ly-6ChiCCR2+ monocytes only under defined host conditions. Nat. Neurosci. 10, 1544–1553.
Nahrendorf, M., Swirski, F. K., Aikawa, E., Stangenberg, L., Wurdinger, T., Figueiredo, J. L., Libby, P., Weissleder, R., and Pittet, M. J. (2007). The healing myocardium sequentially mobilizes two monocyte subsets with divergent and complementary functions. J. Exp. Med. 204, 3037–3047.
Orkin, S. H., and Zon, L. I. (2008). Hematopoiesis: an evolving paradigm for stem cell biology. Cell 132, 631–644.
Perry, V. H., Andersson, P. B., and Gordon, S. (1993). Macrophages and inflammation in the central nervous system. Trends Neurosci. 16, 268–273.
Popovich, P. G., Guan, Z., Wei, P., Huitinga, I., van Rooijen, N., and Stokes, B. T. (1999). Depletion of hematogenous macrophages promotes partial hindlimb recovery and neuroanatomical repair after experimental spinal cord injury. Exp. Neurol. 158, 351–365.
Rapalino, O., Lazarov-Spiegler, O., Agranov, E., Velan, G. J., Yoles, E., Fraidakis, M., Solomon, A., Gepstein, R., Katz, A., Belkin, M., Hadani, M., and Schwartz, M. (1998). Implantation of stimulated homologous macrophages results in partial recovery of paraplegic rats. Nat. Med. 4, 814–821.
Schwartz, M., Moalem, G., Leibowitz-Amit, R., and Cohen, I. R. (1999). Innate and adaptive immune responses can be beneficial for CNS repair. Trends Neurosci. 22, 295–299.
Schwartz, M., and Yoles, E. (2006). Immune-based therapy for spinal cord repair: autologous macrophages and beyond. J. Neurotrauma 23, 360–370.
Shechter, R., London, A., Varol, C., Raposo, C., Cusimano, M., Yovel, G., Rolls, A., Mack, M., Pluchino, S., Martino, G., Jung, S., and Schwartz, M. (2009). Infiltrating blood-derived macrophages are vital cells playing an anti-inflammatory role in recovery from spinal cord injury in mice. PLoS Med. 6, e1000113. doi: 10.1371/journal.pmed.1000113
van Furth, R., Cohn, Z. A., Hirsch, J. G., Humphrey, J. H., Spector, W. G., and Langevoort, H. L. (1972). The mononuclear phagocyte system: a new classification of macrophages, monocytes, and their precursor cells. Bull. World Health Organ. 46, 845–852.
Keywords: microglia, monocyte-derived macrophages, central nervous system, neurodegeneration, neuroinflammation
Citation: Jung S and Schwartz M (2012) Non-identical twins – microglia and monocyte-derived macrophages in acute injury and autoimmune inflammation. Front. Immun. 3:89. doi: 10.3389/fimmu.2012.00089
Received: 10 November 2011; Paper pending published: 28 November 2011;
Accepted: 06 April 2012; Published online: 07 May 2012.
Edited by:
Amiram Ariel, University of Haifa, IsraelReviewed by:
Marco Prinz, University Medical Centre Freiburg, GermanyFabio Rossi, University of British Columbia, Canada
Copyright: © 2012 Jung and Schwartz. This is an open-access article distributed under the terms of the Creative Commons Attribution Non Commercial License, which permits non-commercial use, distribution, and reproduction in other forums, provided the original authors and source are credited.
*Correspondence: Michal Schwartz, Department of Immunology, Weizmann Institute of Science, 76100 Rehovot, Israel. e-mail: michal.schwartz@weizmann.ac.il