- Dipartimento per l’Innovazione nei sistemi biologici, agroalimentari e forestali, Università degli Studi della Tuscia, Viterbo, Italy
Lymphocytes are the responsible of adaptive responses, as they are classically described, but evidence shows that subpopulations of mammalian lymphocytes may behave as innate-like cells, engaging non-self rapidly and without antigen presentation. The innate-like lymphocytes of mammals have been mainly identified as γδT cells and B1-B cells, exert their activities principally in mucosal tissues, may be involved in human pathologies and their functions and tissue(s) of origin are not fully understood. Due to similarities in the morphology and immunobiology of immune system between fish and mammals, and to the uniqueness of having free-living larval stages where the development can be precisely monitored and engineered, teleost fish are proposed as an experimental model to investigate human immunity. However, the homology between fish lymphocytes and mammalian innate-like lymphocytes is an issue poorly considered in comparative immunology. Increasing experimental evidence suggests that fish lymphocytes could have developmental, morphological, and functional features in common with innate-like lymphocytes of mammals. Despite such similarities, information on possible links between conventional fish lymphocytes and mammalian innate-like lymphocytes is missing. The aim of this review is to summarize and describe available findings about the similarities between fish lymphocytes and mammalian innate-like lymphocytes, supporting the hypothesis that mammalian γδT cells and B1-B cells could be evolutionarily related to fish lymphocytes.
Introduction
Vertebrate-type adaptive responses with MHC, RAG, memory, are present in only 2% of metazoans, but invertebrates can live very long protected by their innate immune defenses. Indeed, invertebrates classically defined as relying only on innate responses may live for centuries and have been found to respond to reinfection, suggesting that innate immunity mechanisms need more investigation. In a comparative immunology view, it is conceivable to speculate that leukocytes populations that emerged early in vertebrates evolution inherited and retained some invertebrate features related to antigen recognition and elimination. During evolution, genes coding for immune activities accumulated toward mammals in a form of “layers.” This hypothesis proposes that evolution produced a layered immune system in which following descendants obtain predominance during development, giving rise to cell populations responsible for progressively more complex immune activities. As it is commonly thought that “ontogeny resembles phylogeny,” a “layered immune system” hypothesis may give clues to understand cell functionality in vertebrates and provide knowledge for a better understanding of human pathologies.
Immune innate responses are a first-level of protection against infection and damage, exerted by cells reacting fast to non-self/injury with their germline-encoded receptors. In mammals, there are different types of innate immune cells, besides macrophages/dendritic cells/neutrophils, also innate lymphoid cells (ILC) have been described. ILC are classified in three groups for the expression of defined transcription factors, functional characteristics, and phenotype. Another group of mammalian unconventional or innate-like lymphocytes (mILL) has been identified with properties and functions as a bridge between innate and adaptive responses. Populations of mILL might thus rerepresent an “immune lower layer,” with activities involved in maintaining gut homeostasis, in early response to intestinal infections, in autoimmune diseases and cancer, in a fast unprimed fight against infection and damage, in producing germline natural polyreactive antibodies and typical cytokine patterns. The mILL have been mainly identified as γδT cells and B1-B cells, are mainly located in mucosal tissues, and their functions and origin are still under scrutiny.
Of note, increasing evidence suggests that conventional fish lymphocytes display some developmental, morphological, and functional features in common with mILL and, very recently, these similarities have attracted attention among immunologists (1). However, studies aimed to clarify the links between fish lymphocytes and mIL are at their infancy.
This review is proposed to summarize the current knowledge on possible similarities between fish lymphocytes and mILL, and use the knowledge to raise the hypothesis that most of fish lymphocytes behave like subpopulations of mILL and, consequently, that mILL subpopulations (γδT cells, B1-B cells) could represent a “lower layer” of extant, evolutionary-related, analogs of fish lymphocytes.
Mammalian Innate-Like Lymphocytes
Important players of innate immune activities are the mammalian ILC that derive from a common lymphoid precursor and play a role with effector and regulatory functions in innate immunity and tissue remodeling. The ILC do not have TcR or Ig rearranged receptors in their surface and are classified into three groups on the base of the patterns of cytokines they produce, and of transcription factors necessary to their functions. Namely, ILC1 produce IFNγ and depend on Tbet, ILC2 produce type 2 cytokines (IL-5/IL-13) and require GATA3, ILC3 depend on RORγt and produce IL-17 and/or IL-22 (2). Also natural killer (NK) cells belong to innate lymphocytes are involved in fast innate responses and do not express CD3 or lymphocyte receptors on their surface. However, aside from the classical description of lymphocytes as cells responsible of adaptive responses, subpopulations of recently discovered mILL behave as innate immune cells with respect to the historical innate-adaptive classification (3).
The mILL are involved in maintaining gut homeostasis and in early response to intestinal infections (4, 5), in autoimmune diseases and cancer (6, 7), are able to combat non-self in an MHC-independent fashion (8, 9), produce unbiased natural polyreactive antibodies (10, 11) and typical cytokine patterns (12, 13).
The main lymphocyte subpopulations displaying innate-like activities in mammals have been identified as γδT cells (5), mucosa-associated invariant T cells (MAIT) (14), natural killer T cells (NKT) (15), B1-B cells (16), and spleen marginal zone B cells (17).
Innate-Like T Cells
The γδT lymphocytes are non-conventional T lymphocytes, comprising a minor T cell subset in blood and a major population of intestinal intraepithelial lymphocytes (IELs) having typical morphological features of lymphocytes with a surface germline TCR phenotype of γ+δ+ (mostly displaying repertoires Vδ1/Cγ1 and Vγ9/Vδ2) and showing a potent phagocytic ability to both soluble and particulate antigens (18, 19). With respect to immunoglobulins and αβTcR molecules, the γδTcR displays the highest spontaneous diversity in the CDR3 region produced by VDJ recombination by using the V-chain gene. The γδT cells can develop extrathymically and independently from an antigen encounter and are active players in adaptive and innate-like immune responses such as the direct killing of infected cells, are involved in tumor immunosurveillance (20), produce molecules required for pathogen clearance (21), are spontaneously cytotoxic (22), release immunomodulatory cytokines (23), and can be activated by stress-induced molecules (MIC-A/B, ULBPs) to produce pro-inflammatory cytokines and lytic enzymes. In summary, evidence suggests that γδT cells act either as effectors and regulators (24), and represent an evolutionarily primitive T cell subset characterized by innate and adaptive immune functions. Supporting these findings, recent data also showed the presence of γδT cells subsets for which innate stimuli are more important than TcR ligation, as in the case of IL-17-producing (γδT-17) and IFNγ-producing (γδT-IFNγ) cells (25).
Other subpopulations of recently discovered mammalian innate-like T lymphocytes are the MAIT and NKT. MAIT are an innate T cell subpopulation (14), principally involved in antibacterial immunity at mucosal surfaces, and mainly present in man than in mouse (26), they display a germline TcRαβ phenotype (Vα7.2-Jα33/12/20 in humans, Vα19-Jα33 in mice) and variable but restricted TcRβ chains (5, 27). Upon stimulation, MAIT produce the regulatory cytokines IFNγ, TNFα, and IL-17, and express the receptors for IL-7, IL-12, and IL-18 (26).
The NKT are a subpopulation of αβ- and γδ-T cells differing from NK cells for the presence of CD3 and TcR, characterized by CD1d restriction and limited TcR diversity (15, 28). They are principally present in non-mucosal tissues, are involved in antitumor activity, and are of help for B cell proliferation and antibody production (29). The NKT can be further divided into two distinct subpopulations, namely, type I and type II NKT cells (30) that are preferentially located in the liver. Type I display a semi-invariant TcR (Vα14Jα18/Vβ2, 7, 8) in mice and (Vα24Jα18/Vβ11) in humans, whereas type II NKT cells exhibit a more diverse TcR repertoire.
Innate-Like B Cells
The B lymphocytes of mammals are now cataloged as B2, or classic, and B1, or innate. These two major sets of B cells are defined by differential presence of CD5 in their surface. The B1-B cells are further subdivided in B1a (B1) having a phenotype CD5+/IgMhigh/IgDlow, and B-1b cells, which are CD5-negative (31). The B1-B cells produce large amounts of natural polyreactive antibody in a T cell-independent manner, are actively phagocytic and microbicidal (32), may be involved in autoimmunity (33), and are present as IgA-secreting plasma cells in the intestinal mucosa where they migrate during infections (16). Natural polyreactive antibodies produced by CD5+ B cells are germline-encoded antigen recognition molecules (class IgM, IgA, and IgG3) (11) with a limited repertoire of V-region genes, play an important role in early host defense, in autophagy/tissue remodeling and immune regulation, in recognition of pathogens and activation of the innate immune system via the classical pathway of complement activation (10). The B1-B cells are considered to have no memory, are present in mouse liver at fetal stages (34), whereas in adults are present in the spleen and peritoneal cavity (35, 36), where they undergo self-renewal with mechanisms that are poorly understood.
Being involved in innate activities, B1-B cells respond to stimulation in vitro through TLRs (from TLR1 to TLR8) (37, 38) inducing B1-B cell proliferation and differentiation into immunoglobulin-secreting cells. Also, B1-B cells show a rapid capacity to produce high amounts of the immunomodulatory cytokine IL-10 after innate activation (13).
An additional subpopulation of B cells having innate-like activities is located in the spleen pulp marginal zone and involved in producing IgM antibodies in a T cell-independent manner against pathogens circulating in blood (17).
Of particular interest is the tissue localization of innate-like B cells, which exert their activities principally in mucosal surfaces and mainly in the intestine, where the IgA produced by B1-plasma cells can be spontaneously present, reacting with the intestinal microflora (39). The mucosal intestine is also the richest site of γδT lymphocytes in adult mice and man (40), followed by the respiratory epithelium (24), and the epidermis (41). In mucosal tissues, during a possible infection the mILL displaying germline receptors can respond quickly, thus providing protection independently from adaptive responses and in the absence of antigen exposure as, for instance, in newborns (5).
Fish Lymphocytes
The features of mILL, very briefly summarized above, appear to be remarkably similar to the features of conventional lymphocytes as they are known in teleost fish, where experimental data accumulated in decades of investigation showed the presence of T cells possessing surface αβ- and γδ-TcR, of B cells expressing three immunoglobulin types (IgM, IgT, and IgD), of lymphocyte subpopulations, and a complete set of master genes coding for lymphocyte-associated molecules (42–45). The fish lymphocytes have been shown to be functionally active in vitro and in vivo (46–52), and to produce and/or be affected by families of lymphocyte-related cytokines (53, 54).
Features of Fish T Cells
Two classes of T cells are present in teleost fish, displaying on their cell surface αβ- and γδ-TcR, together with TcR coreceptors, and expressing patterns of genes that clearly indicate the presence of T cell subpopulations as they are known in mammals, namely, cytotoxic (CD8), helper (CD4), and regulatory (Treg, Th17) (45, 55–57). The immunobiology of fish T cells has been the subject of extensive research addressed to investigate regulation mechanisms, expression of surface markers, and in vitro/vivo studies, that have been reassumed in recent reviews (42, 53, 54, 58–60). In relation with the present work, available data have shown that the distribution of T cells in fish is principally located in mucosal tissues of intestine and gills (60–66), and that activities of T cells are diverse in these tissues. In the intestine, IEL displays an in vitro spontaneous cytotoxic activity (65), proliferate poorly (unpublished), and perform in vivo RAG-driven spontaneous somatic rearrangement of a given V/C combination in the CDR3 junction length of TcRβ-chain/TcRγ-chain in the absence of antigen stimulation (64, 67). On the other hand, T cells from the gills are able to proliferate in vitro in response to lectins, but RAG expression is negligible (45). These observations suggest that the teleost intestine could be a site of production of T cells, whereas the gills could be a site where T cells are more committed as effectors/helper. A support to the hypothesis that the fish intestine can be a primary producer of T cells comes from data on the development of sea bass immune system, where first antibody-positive T cells are detected in the developing gut before, or at the same time, than in thymus (68, 69). However, definitive knowledge establishing precise timing and tissue of appearance of T cell subpopulations in fish is still missing (70).
The intestine of sea bass displays a high homogeneous expression of TcRα and TcRγ, a low expression of CD4, and differential expression of CD8α and of MHCII showing an increment and a decrease, respectively, toward the terminal part (65). Considering the number of T cells present in the intestinal mucosa, and that purified T cells from the intestine with a pan-T mAb showed enriched expression of RAG-1, TcRα, TcRγ, CD8α, and CD4, it appears evident that the gut can be considered the main lymphoid tissue for T cells in adult fish (43).
Data obtained on in vitro activity of fish leukocytes suggest the presence of an IL-2 modulated proliferation of T cells during a mixed-leukocyte reaction (48) and of a MHC-restricted CTL activity (52), suggesting that fish immune cells also display activities comparable to classical T lymphocytes of mammals.
Finally, fish do have memory T cells, identified by the IL-10 modulation of CD8- and CD4-populations responses and proliferation in immunized carp (71). Interestingly, it should be noted that mutant zebrafish engineered for lacking somatic recombination (RAG-1−/−) are still able to mount a specific protection after bacterial re-exposure (72), and that Atlantic cod lacks CD4 and MHCII in the genome but is protected during immune challenges with pathogens (73). These latter observations suggest that further research is needed in fish to better elucidate functional features of T cells, such as the phagocytic capability of γδT cells (18).
Features of Fish B Cells
Production of antigen-specific antibody in fish is known since almost 70 years, and research has shown that fish have B cells expressing three heavy Ig chain classes, namely, IgM, IgT/Z, and IgD, as defined by the expressed genes μ, τ, and δ, respectively (74–76), and of some Ig light chains (e.g., two in catfish, three in zebrafish, MW 25–28 kDa) (77). The IgM are tetrameric in fish (MW 450 kDa) and present systemically in body fluids, where they may be present in serum at high concentration. The IgT/IgZ are mucosal immunoglobulins produced in a monomeric form (MW 170 kDa), although a non-covalent polymeric IgT association has been observed in trout mucus. The IgD has been studied at molecular level, it is expressed in a monomeric form with a putative MW of 150 kDa, but little is known on its physiological role in fish (78). Likewise T cells, the B cells of fish have been the subject of much research, with results reassumed in comprehensive reviews (44, 79, 80). With respect to the present work, main activities of fish B cells can be summarized as follows: (i) high content of natural serum IgM in unimmunized fish (81–83); (ii) poor increase in IgM affinity after secondary immunization (84, 85); (iii) presence of memory B cells (85); (iv) spontaneous phagocytosis (86); (v) production of pathogen-induced mucosal secretory IgT (evolutionary orthologs of IgA) (49); (vi) presence of kidney lymphocyte precursors similar to mouse spleen B1-B cells (34, 87); and (vii) presence of proliferating B cells in the peritoneal cavity (88); possible expression of TLRs (89). Interestingly, intriguing features regarding B cells have been observed in some fish species as, for instance, the lacking of pathogen-specific IgM in gadoids after successful immunization against the pathogen (82), and a lack of the whole IgM gene in a coelacanth species (90).
Similarities Between Mammalian Innate-Like and Fish Lymphocytes
The principal features of mILL and of fish conventional lymphocytes have been briefly summarized above, and a comparison of possible similarities is shown in Figure 1 for T cells and in Figure 2 for B cells. A point of great importance to better understand the evolution of lymphocytes among vertebrates is the definition of the primary tissue(s) of origin and of tissue localization during development. Experimental evidence suggests that the fish intestine may be a primary lymphoid tissue for T cells, which can be detected there even before their appearance in thymus (68, 91–93), and where a T cell selection might be present that differs from thymic T cell selection. The possible thymus-independent origin of T cell subpopulations appears to be conserved until mammals, where γδT cells may derive from human fetal liver and the primitive intestine between 6 and 9 weeks of gestation, as proposed by investigating expression of the δTcR repertoire during human development (94, 95). Indeed, γδT cells play a pivotal role during human intestine development, since preterm infants with intestinal barrier immaturity, and thus with a reduction in the number of IEL, may develop severe enterocolitis (96).
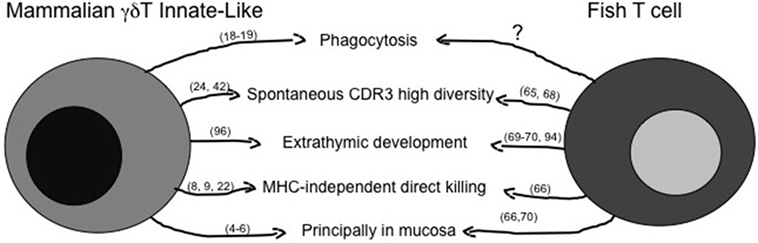
Figure 1. Similarities between mammalian innate-like T lymphocytes and fish T cells. The figure reassumes similar immune activities of γδT innate-like T cells as they are known in mammals and in fish. The question mark represents activities likely present, but not fully demonstrated yet. Main references for the indicated activities are reported in brackets.
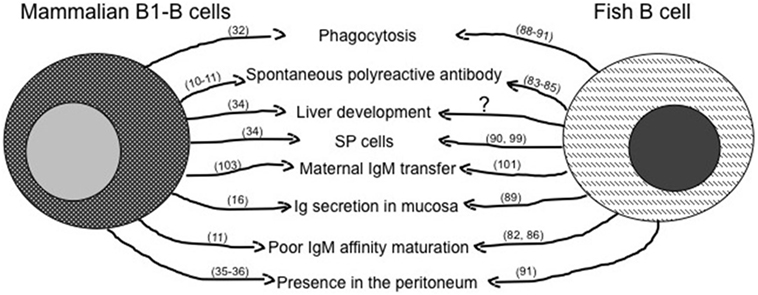
Figure 2. Similarities between mammalian innate-like B lymphocytes and fish B cells. The figure reassumes similar immune activities of B1-B cells as they are known in mammals and in fish. The question mark represents activities likely present, but not fully demonstrated yet. Main references for the indicated activities are reported in brackets.
The importance of liver as a site of possible lymphocytes development emerges from data on the origin of B cells in mammals, where B1a cells have been found to develop in mouse fetal liver, from which they migrate in the spleen, but not in the bone marrow (34). Interestingly, in the mouse spleen, a lymphocyte subpopulation shows flow cytometric morphological features (SP cells) remarkably similar to that of lymphoid SP cells from adult goldfish and zebrafish kidney (87, 97).
The origin of B cells in fish is not clearly defined, in zebrafish the pancreas has been supposed to be a primary site on the base of B cell receptors genes rearrangement (98), whereas in the sea bass kidney a presence of IgM-producing cells has been established by IHC at 55 days post hatching (69). Although it is evident in all fish species investigated that the development of T cells precedes development of B cells, a definitive clarification of a primary site of B cell origin is missing (69).
Another similarity could be found in the transmission of immunity between the female and the developing embryo. In fish, a possible precursor process of the maternal antibody transfer to the fetus through placenta has been observed by the presence of IgM molecules and IgM gene expression in unfertilized eggs and during first embryonic stages (99). In mammals, the B1-B cells are already present at early stages in the extraembryonic yolk sac and continue their development in the fetal liver (100), with the IgM being the predominant class during late gestation and infancy (101).
Other experimental evidence suggests striking similarities between low affinity polyreactive serum natural IgM antibodies produced by mammalian B1-B cells (13) with IgM presence/responses in fish (80). As in mammals and other investigated vertebrate species, the kinetic of primary antibody response in fish involves IgM but, at variance with mammals, in fish there is neither a class-switch secondary response nor a substantial increase in serum IgM affinity, although specific antibody titers can be observed after immunization. Of note, the protection mechanisms and specificity of antibody responses in fish are far to be fully understood, since some fish species result protected after immunization without producing specific IgM antibody (82). In addition, the IgM can be even totally absent, as discovered in a species lacking completely of IgM genes (90). The possible importance of natural IgM in fish as players in innate immunity emerges from their amount in serum, since the mean concentration of IgM in unimmunized fish (sera from five species, 7.7 mg/ml) (81) is much higher than the mean concentration of IgM in humans (1.3 mg/ml) (102). Considering that fish lack IgG, the higher concentration of natural IgM could contribute to immunity against pathogens in not yet completely understood ways, suggesting that research on natural IgM contribution in innate immunity, and the kinetic of production of specific IgM by B cells upon immunization, may give some clues to understand the physiology of natural antibodies in mammals.
The Ig secreted in/by mucosal tissues are particularly important for pathogen clearance at the boundary with external environment, and teleost fish have a mucosa-associated IgT class whose features, like the coating of intestinal commensal microbiota, precede that of mammalian-specific secretory IgA (49). Although IgT is not homologous to IgA, it is evident a convergent evolution of the two molecules, both are multimeric, predominantly produced in the mucosa, and induced by mucosal immunization (103).
Fish leukocytes express TLRs (90), show strong in vitro response to LPS, and respond to flagellin with TLR5 (104), and to viruses and poly I:C with TLR3 (105). Although these responses have been measured in leukocytes, it should be reasonable to speculate that fish B cells should express pathogen-specific conserved TLRs on the base of nucleotide sequences obtained from a transcriptome of head kidney, a B cell lymphopoietic tissue in fish that revealed the presence of several TLRs’ gene expression (106). Given the presence of TLRs on fish B cells, a similarity becomes evident with TLRs on mammalian B1-B cells (37, 38).
Another population of fish IgM-B cells is located in the peritoneal cavity, capable of proliferate very soon after antigenic stimulation, produces polyreative antibodies, and is responsible of pathogen clearance (88). Similarly, in mammals the peritoneal B1-B cells can proliferate rapidly after antigen stimulation and can migrate in the periphery, including the intestine, to fight the pathogen (107).
Summary
The immune defense system of vertebrates in its molecular and cellular components is remarkably conserved from teleost fish, the more ancient extant representatives of the evolutive lineage that directly brings to mammals. The knowledge on the similarities between morphological and physiological processes of vertebrates led to the use of teleost fish as an additional animal model for investigations in pathology and physiology of immune recognition, with the goal of applying results in translational research for modeling human diseases, as can be easily appreciated with the zebrafish model. Therefore, teleost fish play a fundamental role in understanding the evolution of immune responses of vertebrates, and experimental evidence suggests that some features of mammalian innate-like lymphocytes related to pathogenic conditions, such as chronic lymphocytic leukemia and inflammation could benefit from knowledge in fish lymphocytes.
The hypothesis described in this review is that younger species (mammals) retain immune defense features of ancestors (fish) that have been enriched by evolution with new “layers” of genes coding for cells and molecules, with a “lower” immune layer that in mammals might be composed of cells with innate activities, among which innate-like lymphocytes.
The experimental evidence considered in this review suggests similarities in morphology, gene expression, and functional signatures of fish lymphocytes with mammalian innate-like lymphocyte subpopulations, although much remains to be learned on the immunobiology of fish lymphocytes such as the origin/functions of intestinal T cells/γδT cells and of B1-B cells.
Considering that MAIT are restricted to mammals, it remains to elucidate the possible presence of NKT in fish, where a clear surface phenotype identification of spontaneously cytotoxic T cells is missing. It also remains to investigate in more details in fish a precise timing and tissues of origin of αβ/γδT cells, of IgM/IgT B cells, their transcriptomic signatures, and some functional activities like production and kinetic properties of natural polyspecific IgM and phagocytic capability of γδT cells.
Importantly, the hypothesis that subpopulations of mammalian innate-like lymphocytes, namely, γδT cells and B1-B cells could be an extant-like counterpart of fish lymphocytes has been already proposed (32) and supported by a recent publication (1). These works suggest that the origin of lymphocytes, possibly including innate-like lymphocytes, goes back to the origin of all vertebrates (1).
In conclusion, investigations on the development and immunobiology of fish lymphocytes is of great importance in comparative immunology, and possibly important for a better understanding of mammalian innate-like lymphocytes immunobiology and their involvement in human diseases.
Author Contributions
GS was responsible of organizing and supervising the review work. AF was responsible for manuscript writing. SP was responsible for checking the literature references and databases and organizes figures.
Conflict of Interest Statement
The authors declare that the research was conducted in the absence of any commercial or financial relationships that could be construed as a potential conflict of interest.
Funding
Experimental data from authors are from EU-funded projects: 5FP project QLK2-CT-2000-01076 FISHAID, 6FP project CT-2005-007103 IMAQUANIM, 7FP project 311993 TARGETFISH.
References
1. Flajnik MF. A cold-blooded view of adaptive immunity. Nat Rev Immunol (2018). doi:10.1038/s41577-018-0003-9
2. Hazenberg MD, Spits H. Human innate lymphoid cells. Blood (2014) 124:700–9. doi:10.1182/blood-2013-11-427781
3. Verykokakis M, Zook EC, Kee BL. ID’ing innate and innate-like lymphoid cells. Immunol Rev (2014) 261:177–97. doi:10.1111/imr.12203
4. Meresse B, Cerf-Bensussan N. Innate T cell responses in human gut. Semin Immunol (2009) 21:121–9. doi:10.1016/j.smim.2009.01.002
5. Bennett MS, Round JL, Leung DT. Innate-like lymphocytes in intestinal infections. Curr Opin Infect Dis (2015) 28:457–63. doi:10.1097/QCO.0000000000000189
6. Exley MA, Tsokos GC, Mills KH, Elewaut D, Mulhearn B. What rheumatologists need to know about innate lymphocytes. Nat Rev Rheumatol (2016) 12:658–68. doi:10.1038/nrrheum.2016.140
7. Zou C, Zhao P, Xiao Z, Han X, Fu F, Fu L. γδ T cells in cancer immunotherapy. Oncotarget (2017) 8:8900–9. doi:10.18632/oncotarget.13051
8. Sugita M, Brenner MB. T lymphocyte recognition of human group 1 CD1 molecules: implications for innate and acquired immunity. Semin Immunol (2000) 12:511–6. doi:10.1006/smim.2000.0277
9. de Oliveira Henriques MD, Penido C. γδ T lymphocytes coordinate eosinophil influx during allergic responses. Front Pharmacol (2012) 3:200. doi:10.3389/fphar.2012.00200
10. Carroll MC, Prodeus AP. Linkages of innate and adaptive immunity. Curr Opin Immunol (1998) 10:36–40. doi:10.1016/S0952-7915(98)80028-9
11. Panda S, Ding JL. Natural antibodies bridge innate and adaptive immunity. J Immunol (2015) 194:13–20. doi:10.4049/jimmunol.1400844
12. Kabelitz D, Wesch D. Features and functions of gamma delta T lymphocytes: focus on chemokines and their receptors. Crit Rev Immunol (2003) 23:339–70. doi:10.1615/CritRevImmunol.v23.i56.10
13. Zhang X. Regulatory functions of innate-like B cells. Cell Mol Immunol (2013) 10:113–21. doi:10.1038/cmi.2012.63
14. Ussher JE, Klenerman P, Willberg CB. Mucosal-associated invariant T-cells: new players in anti-bacterial immunity. Front Immunol (2014) 5:450. doi:10.3389/fimmu.2014.00450
15. Gapin L, Matsuda JL, Surh CD, Kronenberg M. NKT cells derive from double-positive thymocytes that are positively selected by CD1d. Nat Immunol (2001) 2:971–8. doi:10.1038/ni710
16. Fagarasan S, Shinkura R, Kamata T, Nogaki V, Ikuta V, Honjo T. Mechanism of B1 cell differentiation and migration in GALT. Curr Top Microbiol Immunol (2000) 252:221–9.
17. Lopes-Carvalho T, Kearney JF. Marginal zone B cell physiology and disease. Curr Dir Autoimmun (2005) 8:91–123. doi:10.1159/000082100
18. Wu Y, Wu W, Wong WM, Ward E, Thrasher AJ, Goldblatt D, et al. Human gamma delta T cells: a lymphoid lineage cell capable of professional phagocytosis. J Immunol (2009) 1(183):5622–9. doi:10.4049/jimmunol.0901772
19. Wan F, Hu CB, Ma JX, Gao K, Xiang LX, Shao JZ. Characterization of γδ T cells from zebrafish provides insights into their important role in adaptive humoral immunity. Front Immunol (2017) 7:675. doi:10.3389/fimmu.2016.00675
20. Gober HJ, Kistowska M, Angman L, Jenö P, Mori L, De Libero G. Human T cell receptor gammadelta cells recognize endogenous mevalonate metabolites in tumor cells. J Exp Med (2003) 197:163–8. doi:10.1084/jem.20021500
21. Poggi A, Catellani S, Musso A, Zocchi MR. Gammadelta T lymphocytes producing IFNgamma and IL-17 in response to Candida albicans or mycobacterial antigens: possible implications for acute and chronic inflammation. Curr Med Chem (2009) 16:4743–9. doi:10.2174/092986709789878238
22. Nedellec S, Sabourin C, Bonneville M, Scotet E. NKG2D costimulates human V gamma 9V delta 2 T cell antitumor cytotoxicity through protein kinase C theta-dependent modulation of early TCR-induced calcium and transduction signals. J Immunol (2010) 185:55–63. doi:10.4049/jimmunol.1000373
23. Bonneville M, O’Brien RL, Born WK. Gammadelta T cell effector functions: a blend of innate programming and acquired plasticity. Nat Rev Immunol (2010) 10:467–78. doi:10.1038/nri2781
24. Born WK, Yin Z, Hahn YS, Sun D, O’Brien RL. Analysis of gamma delta T cell functions in the mouse. J Immunol (2010) 184:4055–61. doi:10.4049/jimmunol.0903679
25. Corpuz TM, Stolp J, Kim HO, Pinget GV, Gray DH, Cho JH, et al. Differential responsiveness of innate-like IL-17- and IFN-γ-producing γδ T cells to homeostatic cytokines. J Immunol (2016) 196:645–54. doi:10.4049/jimmunol.1502082
26. Xiao X, Cai J. Mucosal-associated invariant T cells: new insights into antigen recognition and activation. Front Immunol (2017) 8:1540. doi:10.3389/fimmu.2017.01540
27. Le Bourhis L, Guerri L, Dusseaux M, Martin E, Soudais C, Lantz O. Mucosal-associated invariant T cells: unconventional development and function. Trends Immunol (2011) 32:212–8. doi:10.1016/j.it.2011.02.005
28. Pereira P, Boucontet L. Innate NKTgammadelta and NKTalphabeta cells exert similar functions and compete for a thymic niche. Eur J Immunol (2012) 42:1272–81. doi:10.1002/eji.201142109
29. Vomhof-DeKrey EE, Yates J, Leadbetter EA. Invariant NKT cells provide innate and adaptive help for B cells. Curr Opin Immunol (2014) 28:12–7. doi:10.1016/j.coi.2014.01.007
30. Berzins SP, Smyth MJ, Baxter AG. Presumed guilty: natural killer T cell defects and human disease. Nat Rev Immunol (2011) 11:131–42. doi:10.1038/nri2904
31. Tung JW, Mrazek MD, Yang Y, Herzenberg LA, Herzenberg LA. Phenotypically distinct B cell development pathways map to the three B cell lineages in the mouse. Proc Natl Acad Sci U S A (2006) 103:6293–8. doi:10.1073/pnas.0511305103
32. Gao J, Ma X, Gu W, Fu M, An J, Xing Y, et al. Novel functions of murine B1 cells: active phagocytic and microbicidal abilities. Eur J Immunol (2012) 42:982–92. doi:10.1002/eji.201141519
33. Milner EC, Anolik J, Cappione A, Sanz I. Human innate B cells: a link between host defense and autoimmunity? Springer Semin Immunopathol (2005) 26:433–52. doi:10.1007/s00281-004-0188-9
34. Rosado MM, Aranburu A, Capolunghi F, Giorda E, Cascioli S, Cenci F, et al. From the fetal liver to spleen and gut: the highway to natural antibody. Mucosal Immunol (2009) 2:351–61. doi:10.1038/mi.2009.15
35. Hastings WD, Gurdak SM, Tumang JR, Rothstein TL. CD5+/Mac-1- peritoneal B cells: a novel B cell subset that exhibits characteristics of B-1 cells. Immunol Lett (2006) 105:90–6. doi:10.1016/j.imlet.2006.01.002
36. Fagarasan S, Honjo T. T-Independent immune response: new aspects of B cell biology. Science (2000) 290:89–92. doi:10.1126/science.290.5489.89
37. Genestier L, Taillardet M, Mondiere P, Gheit H, Bella C, Defrance T. TLR agonists selectively promote terminal plasma cell differentiation of B cell subsets specialized in thymus-independent responses. J Immunol (2007) 178:7779–86. doi:10.4049/jimmunol.178.12.7779
38. Rawlings DJ, Schwartz MA, Jackson SW, Meyer-Bahlburg A. Integration of B cell responses through toll-like receptors and antigen receptors. Nat Rev Immunol (2012) 12:282–94. doi:10.1038/nri3190
39. Kroese FG, Bos NA. Peritoneal B-1 cells switch in vivo to IgA and these IgA antibodies can bind to bacteria of the normal intestinal microflora. Curr Top Microbiol Immunol (1999) 246:343–9.
40. Kabelitz D, Marischen L, Oberg HH, Holtmeier W, Wesch D. Epithelial defence by gamma delta T cells. Int Arch Allergy Immunol (2005) 137:73–81. doi:10.1159/000085107
41. Jameson JM, Sharp LL, Witherden DA, Havran WL. Regulation of skin cell homeostasis by gamma delta T cells. Front Biosci (2004) 9:2640–51. doi:10.2741/1423
42. Castro R, Bernard D, Lefranc MP, Six A, Benmansour A, Boudinot P. T cell diversity and TcR repertoires in teleost fish. Fish Shellfish Immunol (2011) 5:644–54. doi:10.1016/j.fsi.2010.08.016
43. Boschi I, Randelli E, Buonocore F, Casani D, Bernini C, Fausto AM, et al. Transcription of T cell-related genes in teleost fish, and the European sea bass (Dicentrarchus labrax) as a model. Fish Shellfish Immunol (2011) 5:655–62. doi:10.1016/j.fsi.2010.10.001
44. Scapigliati G. Functional aspects of fish lymphocytes. Dev Comp Immunol (2013) 41(2):200–8. doi:10.1016/j.dci.2013.05.012
45. Nuñez Ortiz N, Gerdol M, Stocchi V, Marozzi C, Randelli E, Bernini C, et al. T cell transcripts and T cell activities in the gills of the teleost fish sea bass (Dicentrarchus labrax). Dev Comp Immunol (2014) 47:309–18. doi:10.1016/j.dci.2014.07.015
46. Abelli L, Baldassini MR, Mastrolia L, Scapigliati G. Immunodetection of lymphocyte subpopulations involved in allograft rejection in a teleost, Dicentrarchus labrax (L.). Cell Immunol (1999) 191:152–60. doi:10.1006/cimm.1998.1430
47. Meloni S, Scapigliati G. Evaluation of immunoglobulins produced in vitro by head kidney leucocytes of sea bass Dicentrarchus labrax by immunoenzymatic assay. Fish Shellfish Immunol (2000) 10:95–9. doi:10.1006/fsim.1999.0226
48. Meloni S, Zarletti G, Benedetti S, Randelli E, Buonocore F, Scapigliati G. Cellular activities during a mixed leucocyte reaction in the teleost sea bass Dicentrarchus labrax. Fish Shellfish Immunol (2006) 20:739–49. doi:10.1016/j.fsi.2005.10.001
49. Zhang YA, Salinas I, Li J, Parra D, Bjork S, Xu Z, et al. IgT, a primitive immunoglobulin class specialized in mucosal immunity. Nat Immunol (2010) 11:827–35. doi:10.1038/ni.1913
50. Castro R, Jouneau L, Pham HP, Bouchez O, Giudicelli V, Lefranc MP, et al. Teleost fish mount complex clonal IgM and IgT responses in spleen upon systemic viral infection. PLoS Pathog (2013) 9:e1003098. doi:10.1371/journal.ppat.1003098
51. Fillatreau S, Six A, Magadan S, Castro R, Sunyer JO, Boudinot P. The astonishing diversity of Ig classes and B cell repertoires in teleost fish. Front Immunol (2013) 4:28. doi:10.3389/fimmu.2013.00028
52. Somamoto T, Koppang EO, Fischer U. Antiviral functions of CD8(+) cytotoxic T cells in teleost fish. Dev Comp Immunol (2014) 2:197–204. doi:10.1016/j.dci.2013.07.014
53. Benedetti S, Randelli E, Buonocore F, Zou J, Secombes CJ, Scapigliati G. Evolution of cytokine response: IL-1 directly affects intracellular Ca2+ concentration of teleost fish leukocytes through a receptor-mediated mechanism. Cytokine (2006) 34:9–16. doi:10.1016/j.cyto.2006.03.009
54. Wang T, Secombes CJ. The cytokine networks of adaptive immunity in fish. Fish Shellfish Immunol (2013) 35:1703–18. doi:10.1016/j.fsi.2013.08.030
55. Nakanishi T, Toda H, Shibasaki Y, Somamoto T. Cytotoxic T cells in teleost fish. Dev Comp Immunol (2011) 35:1317–23. doi:10.1016/j.dci.2011.03.033
56. Takizawa F, Magadan S, Parra D, Xu Z, Korytář T, Boudinot P, et al. Novel teleost CD4-bearing cell populations provide insights into the evolutionary origins and primordial roles of CD4+ lymphocytes and CD4+ macrophages. J Immunol (2016) 196:4522–35. doi:10.4049/jimmunol.1600222
57. Kasheta M, Painter CA, Moore FE, Lobbardi R, Bryll A, Freiman E, et al. Identification and characterization of T reg-like cells in zebrafish. J Exp Med (2017) 214:3519–30. doi:10.1084/jem.20162084
58. Fischer U, Koppang EO, Nakanishi T. Teleost T and NK cell immunity. Fish Shellfish Immunol (2013) 35:197–206. doi:10.1016/j.fsi.2013.04.018
59. Nakanishi T, Shibasaki Y, Matsuura Y. T cells in fish. Biology (2015) 4:640–63. doi:10.3390/biology4040640
60. Tafalla C, Leal E, Yamaguchi T, Fischer U. T cell immunity in the teleost digestive tract. Dev Comp Immunol (2016) 64:167–77. doi:10.1016/j.dci.2016.02.019
61. Fischer U, Utke K, Somamoto T, Kollner B, Ototake M, Nakanishi T. Cytotoxic activities of fish leucocytes. Fish Shellfish Immunol (2006) 20:209–26. doi:10.1016/j.fsi.2005.03.013
62. Buonocore F, Randelli E, Bird S, Secombes CJ, Costantini S, Facchiano A, et al. The CD8alpha from sea bass (Dicentrarchus labrax L.): cloning, expression and 3D modelling. Fish Shellfish Immunol (2006) 20:637–46. doi:10.1016/j.fsi.2005.08.006
63. Buonocore F, Randelli E, Casani D, Guerra L, Picchietti S, Costantini S, et al. A CD4 homologue in sea bass (Dicentrarchus labrax): molecular characterisation and structural analysis. Mol Immunol (2008) 45:3168–77. doi:10.1016/j.molimm.2008.02.024
64. Buonocore F, Castro R, Randelli E, Lefranc M-P, Six A, Kuhl H, et al. Diversity, molecular characterization and expression of T cell receptor in a teleost fish, the sea bass (Dicentrarchus labrax, L). PLoS One (2012) 7(10):e47957. doi:10.1371/journal.pone.0047957
65. Picchietti S, Guerra L, Bertoni F, Randelli E, Belardinelli MC, Buonocore F, et al. Intestinal T cells of Dicentrarchus labrax (L.): gene expression and functional studies. Fish Shellfish Immunol (2011) 30:609–17. doi:10.1016/j.fsi.2010.12.006
66. Tacchi L, Musharrafieh R, Larragoite ET, Crossey K, Erhardt EB, Martin SAM, et al. Nasal immunity is an ancient arm of the mucosal immune system of vertebrates. Nat Commun (2014) 5:5205. doi:10.1038/ncomms6205
67. Bernard D, Six A, Rigottier-Gois L, Messiaen S, Chilmonczyk S, Quillet E, et al. Phenotypic and functional similarity of gut intraepithelial and systemic T cells in a teleost fish. J Immunol (2006) 176:3942–9. doi:10.4049/jimmunol.176.7.3942
68. Picchietti S, Terribili FR, Mastrolia L, Scapigliati G, Abelli L. Expression of lymphocyte antigenic determinants in developing GALT of the sea bass Dicentrarchus labrax (L.). Anat Embriol (1997) 196:457–63. doi:10.1007/s004290050113
69. Rombout JH, Huttenhuis HB, Picchietti S, Scapigliati G. Phylogeny and ontogeny of fish leucocytes. Fish Shellfish Immunol (2005) 19:441–55. doi:10.1016/j.fsi.2005.03.007
70. Zapata A, Diez B, Cejalvo T, Gutiérrez-de Frías C, Cortés A. Ontogeny of the immune system of fish. Fish Shellfish Immunol (2006) 20:126–36. doi:10.1016/j.fsi.2004.09.005
71. Piazzon MC, Wentzel AS, Tijhaar EJ, Rakus KŁ, Vanderplasschen A, Wiegertjes GF, et al. Cyprinid herpesvirus 3 IL-10 inhibits inflammatory activities of carp macrophages and promotes proliferation of IgM+ B cells and memory T cells in a manner similar to carp IL-10. J Immunol (2015) 195:3694–704. doi:10.4049/jimmunol.1500926
72. Hohn C, Petrie-Hanson L. Rag1-/- mutant zebrafish demonstrate specific protection following bacterial re-exposure. PLoS One (2012) 7:e44451. doi:10.1371/journal.pone.0044451
73. Star B, Nederbragt AJ, Jentoft S, Grimholt U, Malmstrøm M, Gregers TF, et al. The genome sequence of Atlantic cod reveals a unique immune system. Nature (2011) 477:207–10. doi:10.1038/nature10342
74. Danilova N, Bussmann J, Jekosch K, Steiner LA. The immunoglobulin heavychain locus in zebrafish: identification and expression of a previously unknown isotype, immunoglobulin Z. Nat Immunol (2005) 6:295–302. doi:10.1038/ni1166
75. Hansen JD, Landis ED, Phillips RB. Discovery of a unique Ig heavy-chain isotype (IgT) in rainbow trout: implications for a distinctive B cell developmental pathway in teleost fish. Proc Natl Acad Sci U S A (2005) 102:6919–24. doi:10.1073/pnas.0500027102
76. Picchietti S, Nuñez-Ortiz N, Stocchi V, Randelli E, Buonocore F, Guerra L, et al. Evolution of lymphocytes: immunoglobulin T of the teleost sea bass (Dicentrarchus labrax): quantitation of gene expressing and immunoreactive cells. Fish Shellfish Immunol (2017) 63:40–52. doi:10.1016/j.fsi.2017.02.002
77. Hsu E, Criscitiello MF. Diverse immunoglobulin light chain organizations in fish retain potential to revise B cell receptor specificities. J Immunol (2006) 177:2452–62. doi:10.4049/jimmunol.177.4.2452
78. Ramirez-Gomez F, Greene W, Rego K, Hansen JD, Costa G, Kataria P, et al. Discovery and characterization of secretory IgD in rainbow trout: secretory IgD is produced through a novel splicing mechanism. J Immunol (2012) 188:1341–9. doi:10.4049/jimmunol.1101938
79. Salinas I, Zhang YA, Sunyer JO. Mucosal immunoglobulins and B cells of teleost fish. Dev Comp Immunol (2011) 35:1346–65. doi:10.1016/j.dci.2011.11.009
80. Ye J, Kaattari IM, Ma C, Kaattari S. The teleost humoral immune response. Fish Shellfish Immunol (2013) 35:1719–28. doi:10.1016/j.fsi.2013.10.015
81. Scapigliati G, Chausson F, Cooper EL, Scalia D, Mazzini M. Qualitative and quantitative analysis of serum immunoglobulins of four Antarctic fish species. Polar Biol (1997) 18:209–13. doi:10.1007/s003000050177
82. Magnadottir B, Gudmundsdottir S, Gudmundsdottir BK, Helgason S. Natural antibodies of cod (Gadus morhua L.): specificity, activity and affinity. Comp Biochem Physiol B Biochem Mol Biol (2009) 154:309–16. doi:10.1016/j.cbpb.2009.07.005
83. Katzenback BA, Plouffe DA, Belosevic M. Goldfish (Carassius auratus L.) possess natural antibodies with trypanocidal activity towards Trypanosoma carassii in vitro. Fish Shellfish Immunol (2013) 34:1025–32. doi:10.1016/j.fsi.2012.12.018
84. Kaattari SL, Zhang HL, Khor IW, Kaattari IM, Shapiro DA. Affinity maturation in trout: clonal dominance of high affinity antibodies late in the immune response. Dev Comp Immunol (2002) 26:191–200. doi:10.1016/S0145-305X(01)00064-7
85. Ma C, Ye J, Kaattari SL. Differential compartmentalization of memory B cells versus plasma cells in salmonid fish. Eur J Immunol (2013) 43:360–70. doi:10.1002/eji.201242570
86. Li J, Barreda DR, Zhang YA, Boshra H, Gelman AE, Lapatra S, et al. B lymphocytes from early vertebrates have potent phagocytic and microbicidal abilities. Nat Immunol (2006) 7:1116–24. doi:10.1038/ni1389
87. Kobayashi I, Saito K, Moritomo T, Araki K, Takizawa F, Nakanishi T. Characterization and localization of side population (SP) cells in zebrafish kidney hematopoietic tissue. Blood (2008) 111:1131–7. doi:10.1182/blood-2007-08-104299
88. Castro R, Abós B, González L, Granja AG, Tafalla C. Expansion and differentiation of IgM+ B cells in the rainbow trout peritoneal cavity in response to different antigens. Dev Comp Immunol (2017) 70:119–27. doi:10.1016/j.dci.2017.01.012
89. Pietretti D, Wiegertjes GF. Ligand specificities of toll-like receptors in fish: indications from infection studies. Dev Comp Immunol (2014) 43:205–22. doi:10.1016/j.dci.2013.08.010
90. Amemiya CT, Alföldi J, Lee AP, Fan S, Philippe H, Scapigliati G, et al. Analysis of the African coelacanth genome sheds light on tetrapod evolution. Nature (2013) 496:311–6. doi:10.1038/nature12027
91. Matsunaga T, Rahman A. In search of the origin of the thymus: the thymus and GALT may be evolutionarily related. Scand J Immunol (2001) 53:1–6. doi:10.1046/j.1365-3083.2001.00854.x
92. Abelli L, Picchietti S, Romano N, Mastrolia L, Scapigliati G. Immunocytochemical detection of a thymocyte antigenic determinant in developing lymphoid organs of sea bass Dicentrarchus labrax (L.). Fish Shellfish Immunol (1996) 6:493–505. doi:10.1006/fsim.1996.0047
93. Peaudecerf L, Rocha B. Role of the gut as a primary lymphoid organ. Immunol Lett (2011) 140:1–6. doi:10.1016/j.imlet.2011.05.009
94. McVay LD, Carding SR. Extrathymic origin of human γδ T cells during fetal development. J Immunol (1996) 157:2873–82.
95. McVay LD, Jaswal SS, Kennedy C, Hayday A, Carding SR. The generation of human gammadelta T cell repertoires during fetal development. J Immunol (1998) 160:5851–60.
96. Weitkamp J-H, Rosen MJ, Zhao Z, Koyama T, Geem D, Denning TL, et al. Small intestinal intraepithelial TCRcd+ T lymphocytes are present in the premature intestine but selectively reduced in surgical necrotizing enterocolitis. PLoS One (2014) 9:e99042. doi:10.1371/journal.pone.0099042
97. Kobayashi I, Moritomo T, Ototake M, Nakanishi T. Isolation of side population cells from ginbuna carp (Carassius auratus langsdorfii) kidney hematopoietic tissues. Dev Comp Immunol (2007) 31:696–707. doi:10.1016/j.dci.2006.10.003
98. Danilova N, Steiner LA. B cells develop in the zebrafish pancreas. Proc Natl Acad Sci U S A (2002) 99:13711–6. doi:10.1073/pnas.212515999
99. Picchietti S, Scapigliati G, Fanelli M, Barbato F, Canese S, Mastrolia L, et al. Sex-related variation in serum immunoglobulins during reproduction in gilthead seabream and evidence for a transfer from the female to the eggs. J Fish Biol (2002) 59:1503–11. doi:10.1111/j.1095-8649.2001.tb00215.x
100. Yoshimoto M. The first wave of B lymphopoiesis develops independently of stem cells in the murine embryo. Ann N Y Acad Sci (2015) 1362:16–22. doi:10.1111/nyas.12612
101. Aranburu A, Piano Mortari E, Baban A, Giorda E, Cascioli S, Marcellini V, et al. Human B-cell memory is shaped by age- and tissue-specific T-independent and GC-dependent events. Eur J Immunol (2017) 47:327–44. doi:10.1002/eji.201646642
102. Gonzalez-Quintela A, Alende R, Gude F, Campos J, Rey J, Meijide LM, et al. Serum levels of immunoglobulins (IgG, IgA, IgM) in a general adult population and their relationship with alcohol consumption, smoking and common metabolic abnormalities. Clin Exp Immunol (2008) 151:42–50. doi:10.1111/j.1365-2249.2007.03545.x
103. Macpherson AJ, Geuking MB, McCoy KD. Immunoglobulin A: a bridge between innate and adaptive immunity. Curr Opin Gastroenterol (2011) 27:529–33. doi:10.1097/MOG.0b013e32834bb805
104. Tsukada H, Fukui A, Tsujita T, Matsumoto M, Iida T, Seya T. Fish soluble toll-like receptor 5 (TLR5S) is an acute-phase protein with integral flagellin-recognition activity. Int J Mol Med (2005) 15:519–25.
105. Zhang J, Kong X, Zhou C, Li L, Nie G, Li X. Toll-like receptor recognition of bacteria in fish: ligand specificity and signal pathways. Fish Shellfish Immunol (2014) 41:380–8. doi:10.1016/j.fsi.2014.09.022
106. Gerdol M, Buonocore F, Scapigliati G, Pallavicini A. Analysis and characterization of the head kidney transcriptome from the Antarctic fish Trematomus bernacchii (Teleostea, Notothenioidea): a source for immune relevant genes. Mar Genomics (2015) 20:13–5. doi:10.1016/j.margen.2014.12.005
Keywords: innate immunity, innate-like lymphocytes, fish lymphocytes, innate lymphoid cells, comparative immunology
Citation: Scapigliati G, Fausto AM and Picchietti S (2018) Fish Lymphocytes: An Evolutionary Equivalent of Mammalian Innate-Like Lymphocytes? Front. Immunol. 9:971. doi: 10.3389/fimmu.2018.00971
Received: 14 February 2018; Accepted: 18 April 2018;
Published: 07 May 2018
Edited by:
Brian Dixon, University of Waterloo, CanadaReviewed by:
Teruyuki Nakanishi, Nihon University, JapanMike Criscitiello, Texas A&M University, United States
Copyright: © 2018 Scapigliati, Fausto and Picchietti. This is an open-access article distributed under the terms of the Creative Commons Attribution License (CC BY). The use, distribution or reproduction in other forums is permitted, provided the original author(s) and the copyright owner are credited and that the original publication in this journal is cited, in accordance with accepted academic practice. No use, distribution or reproduction is permitted which does not comply with these terms.
*Correspondence: Giuseppe Scapigliati, scapigg@unitus.it