Offshore Aquaculture: I Know It When I See It
- 1National Center for Ecological Analysis and Synthesis, University of California, Santa Barbara, Santa Barbara, CA, United States
- 2Bren School of Environmental Science and Management, University of California, Santa Barbara, Santa Barbara, CA, United States
- 3Department of Life Sciences, Imperial College London, Ascot, United Kingdom
Offshore aquaculture is increasingly viewed as a mechanism to meet growing protein demand for seafood, while minimizing adverse consequences on the environment and other uses in the oceans. However, despite growing interest in offshore aquaculture, there appears to be no consensus as to what measures commonly define an offshore site or how effects of offshore aquaculture—relative to more nearshore practices—are assessed. This lack of agreement on what constitutes offshore aquaculture has the potential to convolute communication, create uncertainty in regulatory processes, and impede understanding of the ecological implications of offshore farming. To begin addressing these issues, we reviewed and analyzed biologically-focused primary and gray literature (Ntotal = 70) that categorize and quantify characteristics of offshore aquaculture from around the world. We found that many “offshore” descriptions are relatively close to shore (<3 nm) and significantly shallower (minimum depth ≤30 m) than may be assumed. We also uncovered an overall lack of consistent reporting of even the most common location-focused metrics (distance from shore, depth, current), a dearth of impact related studies (n = 17), and narrow scope of the studies themselves (i.e., 82% nutrient pollution). Of the finite subset of articles that investigated negative ecological impacts of offshore aquaculture, we found the probability of any measurable impact from an offshore farm appears to significantly decrease with distance from the farm (probability of measurable response at 90 m ± SE = 0.01 ± 0.03). Such general, but informative points of reference could be more robustly quantified with better systematic and standardized reporting of physical farm characteristics and a broader scope of ecological investigation into the effects of marine aquaculture. With offshore aquaculture still in its infancy, consistent metrics are needed for a comparable framework to guide sustainable offshore aquaculture research and development globally.
Introduction
Aquaculture now surpasses global capture fisheries in seafood production (FAO, 2016). With an ever increasing global appetite for seafood and need for protein (Watson et al., 2015; FAO, 2016), expansion of aquaculture farther into the ocean appears inevitable. Indeed, the United States of America (USA) just passed its first regulatory policy for offshore aquaculture (NOAA, 2016), and other countries are also exploring and/or implementing offshore farming regulations, including the European Union, New Zealand, and Australia (Skladany et al., 2007; Sturrock et al., 2008; Ögmundarson et al., 2011). Yet, official reports of these different regulations are difficult to find and appear to vary in how “offshore” (or “open-ocean”) is used. Indeed, some scientific articles on aquaculture report there is no agreed definition of “offshore aquaculture” (Holmer, 2010; Klinger and Naylor, 2012). Such terminology is not simply semantics; it has important implications for public perception and understanding, regulatory implementation and comparison, and environmental consequences (Holmer, 2010; Froehlich et al., 2017).
One of the biggest concerns over aquaculture development is its impact on the environment (Dalton, 2004; Bostock et al., 2010), but these concerns largely derive from specific examples of coastal or inland aquaculture practices. Offshore aquaculture may help mitigate some of the negative consequences associated with more nearshore and land-based farms. Less freshwater need, greater distance and depth from shore, and/or faster currents could help reduce impacts such as pollution, disease occurrence, and area use conflicts (Bostock et al., 2010; Holmer, 2010; Price et al., 2015). However, it is difficult to critically assess the impacts and benefits of offshore aquaculture when the very concept of offshore is open to a variety of interpretations. The few studies of offshore aquaculture are either site or species specific, or use a qualitative review to develop a (top-down) description of “offshore” (Holmer, 2010; Klinger and Naylor, 2012). These approaches aid in disentangling some of the uncertainty around what makes a farm “offshore,” but it remains exceedingly difficult to compare, interpret, and communicate offshore aquaculture potential at regional or global scales without a more comprehensive understanding of the similarities and differences in how “offshore” is defined and used.
Terminology is important for streamlining marine policy, communication, and research. Better understanding about how words or terms are used can help identify key areas of overlap and/or differences, and help make concepts more tractable to stakeholders. Indeed, communication between the public, managers, and scientists requires better elucidations of terms, particularly at a global scale. For instance, Ecosystem Based Management (EBM) is a strategy being adopted in various management contexts—including the marine environment—but how the term is used has huge implications for measuring its adoption and usefulness as a management approach (Arkema et al., 2006). Evaluation of how EBM was referenced uncovered a disconnect in the translation between scientific descriptions and actual management plans, providing key insight and need for clarifying language (Arkema et al., 2006). The same appears to be true for offshore aquaculture (Holmer, 2010; Klinger and Naylor, 2012). Major benefits of synthesizing and investigating trends in terminology of offshore aquaculture include identifying common comparative metrics, data needs, and possible reference points for future research and development.
Our study provides the most comprehensive evaluation of offshore aquaculture in primary and gray literature. This research had three primary objectives: (1) use a bottom-up approach to compile characteristics of aquaculture farms that are described in the literature as “offshore” and compare the most common definitions and metrics, (2) quantify the environmental performance of self-defined offshore farms and assess the relationship between environmental impact and site characteristics, and (3) identify the ecological implications and data gaps of offshore studies. In considering these objectives, we also compare the offshore metrics relative to country and species being investigated. Ultimately, this paper helps establish a baseline knowledge for comparing offshore vs. nearshore aquaculture and suggestions on which site characteristics are most important for promoting sustainability objectives. Investigating and quantifying how “offshore” is being used around the world also provides a necessary glimpse of current offshore practices and provides insight into future potential.
Materials and Methods
Offshore Articles and Metrics
We first compiled primary and gray literature definitions and the most common numeric measures of the term “offshore aquaculture.” The literature search was conducted (Nov. 2015–March 2016) using Google Scholar, Web of Science (WOS), and the journal Aquaculture. Initial review of papers explicitly defining “offshore” provided a very small sample size (n = 27). A paper was considered as clearly defining offshore aquaculture if it included any qualitative or quantitative discussion of the treatment of the term. We expanded our dataset by including papers with implicit or common-use definitions of “offshore aquaculture” (44 additional papers). For example, papers that did not overtly set a definition of offshore, but referred to a study site(s) as being “offshore” were included. Any papers that did not explicitly use “offshore” in the title, abstract, and/or tags were not included in the analysis of quantifying offshore. Synonymous terms to “offshore,” including “open-ocean” and “sea ranching” were well captured by the “offshore” search. To avoid biases of a particular metric or definition, if the same author referenced the identical study site and metrics in multiple papers, they were included in the dataset but treated as a single data point for quantitative analysis.
Only biological-based articles associated with offshore aquaculture were included for in-depth analysis (Ntotal = 70). Papers with purely financial, technical, political, or theoretical aspects were not considered. The purpose of this biological-based exclusion criteria was to create a dataset of comparable articles as they relate to the potential environmental implications of offshore farming. In other words, including the non-biological papers may skew the relative “data gaps” of impact studies.
Physical characteristics of the study site(s) or definitions (as numeric metrics) were included as data for the quantitative analysis of offshore. A total of 10 unique classification criteria were considered. If multiple numeric values for a single metric were given in an article, we would extract the maximum and minimum values. If applicable, we also noted the species and country of the farm described in each article. Some papers mentioned these criteria without giving any specific value for the metric. As a result, we collected data on whether the metric was mentioned and/or actually measured to get a more complete understanding of metric importance (mentioned) vs. practice (measured).
We also recorded whether a paper measured any specific ecological impact. Of the papers that did measure such impacts (n = 17), we collected additional measurements on the specific impact (positive or negative and type), sampling distance(s) from the farm (nm), current (m/s), if and when (i.e., what distance from the farm) statistically significant effects were detected, the farm's distance from the seafloor (m), the size/production of the farm, grow-out period, and feeding rate. It is important to note that the reporting of the latter three measures was sparse and highly variable in how they were reported (e.g., tons yr −1 vs. number of fish), making them inherently difficult to robustly analyze. Nonetheless, we collected any and all available information on farm production parameters in order to make some level of qualitative inference concerning farming impacts, highlight the inconsistences in recording, and underscore these important data-gaps.
Analysis
Before selecting the biologically focused papers for more in-depth analysis, we first assessed the relative frequency of “offshore aquaculture” in the primary literature by measuring the trend of citations from WOS (Nwos = 104). We specifically estimated the linear rate of change of the number of offshore citations per number of publications per year. The citation values were natural-log transformed for constant variance. We also compared which journals most commonly publish offshore aquaculture research in order to determine if offshore aquaculture is a concept restricted to aquaculture-specific audiences or is being used in more general ecological and/or higher impact journals.
Of the biologically focused articles (Ntotal = 70), we compared general themes across the articles, including combinations of how and if “offshore” was defined, what species were most commonly assessed, and/or which countries most commonly referenced the term. Whether or not an article explicitly defined “offshore” helped identify a consensus—or lack thereof—of a particular global description. Exploring the type of species and taxon groups (i.e., finfish, mollusc, multispecies, and seaweed) most commonly reported offered some additional insight into what is, or can be, farmed in an offshore context; the same idea applied to measuring countries referencing or conducting offshore research. Indeed, there is little synthesized data concerning what and where specifically offshore production is being explored and/or implemented. Chi-squared (X2) tests were applied to detect statistical differences between the proportion of articles defining offshore, the type of species and groups mentioned, and countries reporting on offshore aquaculture.
We then ranked the 10 most common metrics that were used to describe or define the physical location of the offshore aquaculture farms, and recorded the value for each of these criteria when stated. Comparing the metrics and associated numeric values highlighted important data-gaps for some of the most cited, but unmeasured, metrics.
Depth (m) and distance from shore (nm) were two a priori criteria used to determine the minimum threshold for where “offshore” farms are sited or described. As a meaningful measure of comparison, we calculated what proportion of articles described minimum values greater than 3nm [border between state waters and federal exclusive economic zone (EEZ) in the United States] and/or depth greater than or equal to 30 m (shallower depths is considered relatively shallow, sub-tidal habitat). We specifically focused on minimum measures to explore the lower limit of “offshore” descriptions. Not all studies report both metrics, so we analyzed distance and depth data independently and together using Chi-squared tests to determine statistical differences between the respective proportions.
Lastly, for the subset of impact studies (n = 17) we calculated the probability of a measurable, negative impact given a set of predictor variables. Descriptive comparisons of the impact-based studies involved the type of impact measured and whether the results were reported as statistically significant. We then used binomial generalized linear models (GLMs) with a logit-link function to estimate the probability of any measurable, negative response (1 = response detected; 0 = response not detected) for each independent variable. Only three of the possible five covariates had enough data to compute a probability estimate; they included sampling distance(s) from the farm (nm), current (m/s), and farm distance from the seafloor (m). These were first modeled separately and the level of deviance explained and statistical significance (p < 0.05) of the respective predictors were noted. We then compared the strength of the covariates to each other by using a subset of articles that reported numeric values for all three metrics (n = 6). Predictor performance in explaining the impact response variable was determined using Akaike Information Criterion Corrected (AICc) (Burnham and Anderson, 2002). Models with AICc values within two units of the smallest AICc model were considered equivalent (Burnham and Anderson, 2002), at which time principal of parsimony was used in the selection of the “best” model (i.e., model with the fewest numbers of parameters). Although it involved a smaller sample size, the AICc model selection approach gave additional support to the individual-based model comparisons. All analyses were performed in R v3.2.0 (RCoreTeam, 2015).
Production information (size/production of the farm, grow-out period, and feeding rate) and studies comparing nearshore vs. offshore practices were sparse. However, we do explore and highlight studies that provide general inference to offshore performance and compare the findings to our generalized model results.
Results
Citation Trends
Citations of “offshore aquaculture” reported in WOS are increasing rapidly, particularly in the last decade, usually in the journal Aquaculture (Figure 1A). The first publication to use “offshore aquaculture” occurred in 1986 in the journal Aquaculture Engineering (Twu et al., 1986), but it was not until the mid-2000s that the term started to increase in prevalence in the primary literature (Ntotal = 104 articles). Over the last 12 years (2004–2016) citations relative to the number of publications has increased at a rate of 2.1 (SE ± 0.11; = 0.88) per year. In fact, just in 2016, reference to “offshore aquaculture” spiked with 44 offshore citations (Figure 1A). This may be due to a combination of interest and convergence of terminology. Notably, reference to offshore aquaculture is not common in high impact (e.g., Science) or policy journals and instead is more common in more specialized, aquaculture periodicals (Figure 1B).
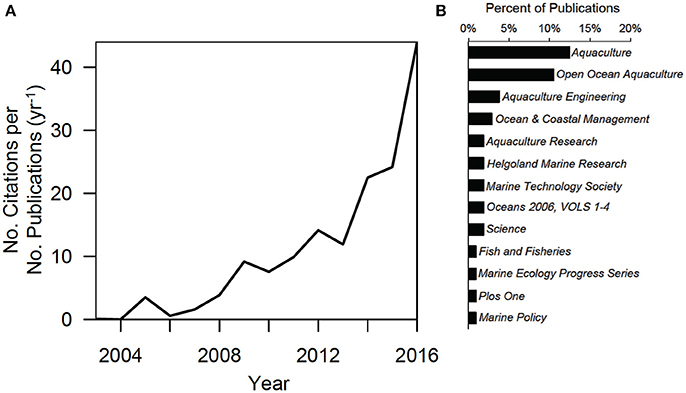
Figure 1. (A) Web of Science (WOS) citations of “offshore aquaculture” over time, relative to the number of publications per year and (B) the associated journals pertaining to the publications (percent contribution).
Metrics
Only a subset (39%) of the biologically relevant papers define or reference a definition for “offshore,” most of which focus on finfish and the United States of America (USA). A total of 70 publications, spanning 1999 to 2016 used the term “offshore aquaculture” and were biologically focused. Significantly fewer papers (X2 = 3.21, p = 0.037; n = 27) actually provided some reference to or tried to define “offshore aquaculture.” Of all 70 papers, significantly more involved finfish (X2 = 5.02, p = 0.013; Figure 2), the majority of which focused on seabream spp., cobia Rachycentron canadum, sea bass spp., and/or Pacific threadfin (moi) Polydactylus sexfilis (Figure 2). The fewer mollusc references were almost entirely focused on blue mussel Mytilus edulis and Pacific oyster Crassostrea gigas, while seaweed represented only one species, Laminaria saccharina. A total of 16 countries/regions were captured in the offshore papers (Figure 2). Most articles (62%) described farms located in the USA, Spain, and Germany (X2 = 2.91, p = 0.044). However, China showed the most diverse array of studies, with articles on each taxonomic category (Figure 2). Papers that define “offshore” focus on farms located in only 10 regions, and these definitions tended to vary. For example, only articles pertaining to the United States (nusa = 8 out of 11) defined offshore aquaculture based on the Exclusive Economic Zone (EEZ).
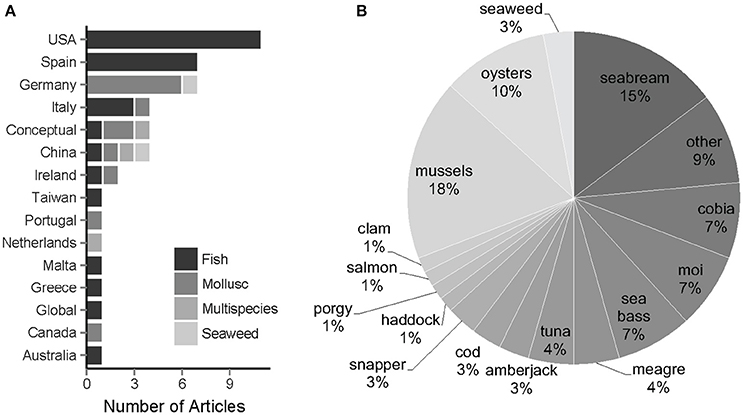
Figure 2. (A) Number of biologically relevant articles and the associated countries and taxonomic groups referenced in gray and primary literature. Conceptual refers to articles that had no particular country of focus, but rather reviewed and/or discussed offshore aquaculture in a conceptual context. In the legend, multispecies (medium gray) represents studies that incorporate a combination of two or more of the taxonomic groups. (B) Percent of offshore aquaculture articles that focus on the species or group of species listed.
A total of 10 metrics were frequently used to describe offshore aquaculture locations, with distance to shore, depth, and current as the most common (Figure 3). The average article mentioned 2.7 (SD ± 1.8) metrics, with some articles listing as many as eight and five papers not offering any clear categorizations of “offshore.” Of the 65 articles that did cite at least one offshore metric, only 58 provided at least one numeric criteria measure (Table 1). However, only 5 of the 10 metrics had any quantifiable measure and the values varied widely (Table 1). Notably, current was the third most cited offshore metric (Figure 3) and correlated strongly with the impact related studies (13 of 17).
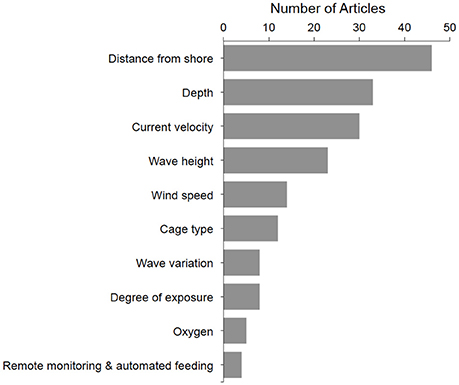
Figure 3. Top 10 most referenced criteria for “offshore aquaculture” and the number of articles that reference each metric. A paper can fall into one or more of these classifications.
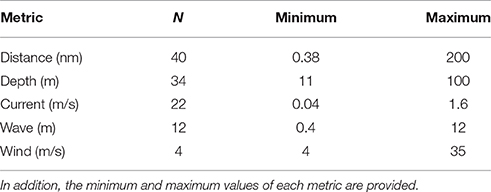
Table 1. List of metrics and the number of articles (N) that provide that particular numeric measure.
Using minimum distance from shore and depth to analytically compare “offshore” categorizations uncovered a large proportion of offshore references relatively close to shore and/or in relatively shallow water (Figure 4). A total of 52 of the 70 biology-based articles specifically provided a distance and/or depth. Regarding distance (n = 40), 50% of publications described offshore sites that are less than 3 nm from shore. In fact, the closest “offshore” farm reference was only 0.38 nm (ca. 0.7 km) from shore. For depth (n = 34), a significantly (X2 = 4.97, p = 0.013) larger proportion (71%) was described within the 30m sub-tidal zone (Figure 4). The absolute minimum depth reported was 11 m. Although, in a smaller subset of the data (n = 22), approximately half of the studies that provide a distance and depth measure were close to shore (<3 nm) and shallow (≤30 m; Figure 4). Four case-study, scientific articles referenced distances greater than 10nm in the German Bight (Brenner et al., 2012), the Gulf of Mexico (Bridger and Costa-Pierce, 2002), the North Sea (Buck and Buchholz, 2005), and southern Australian waters (Kirchhoff et al., 2011).
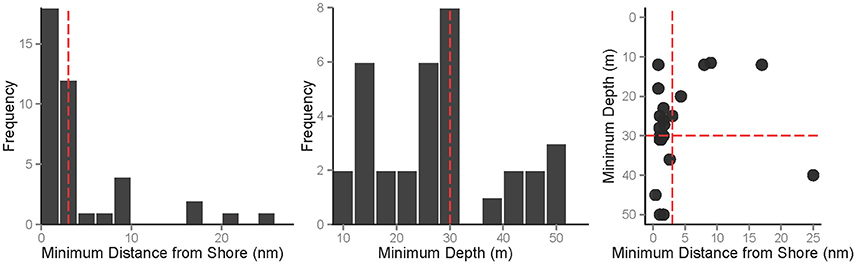
Figure 4. The two most common metrics, minimum distance from shore (nm) and minimum depth (m). Depicted are the frequency distributions of the individual metrics (distance left panel; depth middle panel) and the articles that provided both distance and depth (right panel). The red dashed lines show the pre-assigned threshold for distance (3 nm) and depth (30 m) comparisons.
Impact Studies
Only 23% percent of studies focused on negative ecological impacts, which included nutrient pollution, benthic community changes, parasitic loading and condition factor of farmed species, hydrological disruption (i.e., cage induced downwelling), and changes in eelgrass isotopic signatures. The majority of those (14 of 17) were nutrient and benthos related. The eelgrass study (Ruiz et al., 2010) was excluded from the final impact analysis because the documented changes could not be conclusively linked to aquaculture farming; however, it was an important outlier in being the only study to explore isotopic changes of non-infaunal species. Only 4 of the 17 studies reported statistically significant results, 5 found some (non-significant) measurable change, and 8 of the 17 studies documented no discernable negative impacts. For these papers, minimum distance from shore ranged from 0.38 to 25 nm (mean ± SD = 5.2 nm ± 8.3), minimum depth 15 to 45 m (28.9 m ± 9.2), and minimum current 0.05 to 0.31 m/s (0.18 m/s ± 0.13).
The probability of any measurable, negative impact was best predicted by distance sampled from the farm (Figure 5). When distance from farm (nm), current (m/s), and farm distance from seafloor (m) were compared independently and together, only distance from farm was statistically significant (z-value = −2.33, df = 36, p(distance) = 0.02) and had the strongest model criterion support (i.e., smallest ΔAICc and fewest number of parameters; Table 2), respectively. The probability of a measurable response spanned from 0 m, directly under the farm, [P(response) ± SE = 0.76 ± 0.12] to 0.05 nm, ca. 90 m from the farm [P(response) = 0.01 ± 0.03]. However, it should be noted that the AICc analysis showed some support (ΔAICc ≤ 2; Table 2) for the other predictor variables when combined with sampling distance. Although not statistically significant, the current velocity of studies that reported any measurable change were, on average, 0.07 m/s slower than the studies that found no impact (mean ± SD = 0.22 m/s ± 0.16). Similarly, the mean distance from the seafloor was also slightly closer (16.4 m ± 20.1) for the studies that documented some level of change compared to those that found no measurable impact (18.9 m ± 13.4). However, the highly variable, small samples sizes made it difficult to robustly model the effect. This suggests more impact-related research is needed to truly quantify the relative probability of current and/or distance from the bottom influencing ecological system responses.
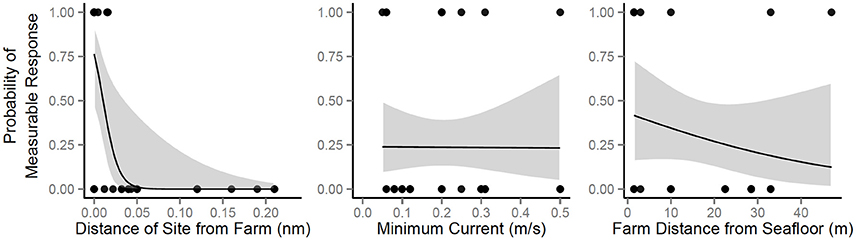
Figure 5. Probability of measurable response given sampling distance from the farm (left panel), minimum current (middle panel), or distance of the cage(s) from the seafloor (right panel). Response data points are depicted as black dots (response = 1; no response = 0). For better visualization, a truncated view of the sample distance from farm probability distribution is depicted, but all data points were used in the GLM analyses [max sample distance (no response) = 1.51 nm].
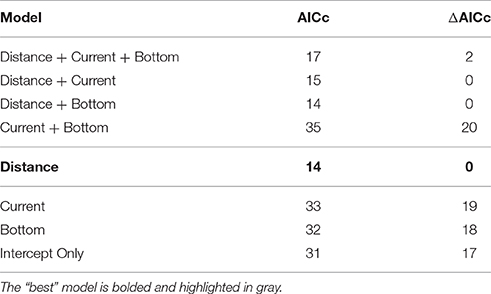
Table 2. Comparative AICc model results for predicting some measureable impact or response on the surrounding environment of the offshore farm.
Production information and comparative farm studies (nearshore vs. offshore) were limited, but do demonstrate offshore potential to reduce some environmental impacts. Only seven studies described any measure of total production and even fewer provided any additional farming parameters. Just three studies provided relative comparisons of nearshore vs. offshore practices. The first study focused on seabream and sea bass production in the Mediterranean, Italy (Vizzini and Mazzola, 2006). The offshore farm (1.08 nm from shore, 0.1 m/s current) had a 3-fold increase in production compared to the nearshore facility (distance not provided, 0.07 m/s), with apparent indistinguishable enrichment (δ15N) on the surrounding environment. The second, (Kirchhoff et al., 2011), assessed the parasitic loads, condition, and performance of more nearshore (distance = 16 nm, depth = 20 m, and current ≤ 0.01 m/s) and offshore (25 nm, 40 m, and 0.05 m/s) Southern Bluefin tuna Thunnus maccoyii cultivation off the southern coast of Australia and found at or above significant improvement for all measures. The last study evaluated offshore (16 nm) vs. nearshore (0.07 nm) blue mussels in the German Bight, finding significantly better condition factors and reduced parasite loads due to dilution of contamination (Brenner et al., 2012); current and depth were not provided. While the studies are few, the findings do appear to show considerable potential of offshore practices to mitigate pollution and disease across species and taxonomic groups.
Discussion
Offshore aquaculture research is increasing, but there are still very few biologically focused articles. Our synthesis supports what other studies have documented in the past (Holmer, 2010; Klinger and Naylor, 2012): there is no clear consensus describing what “offshore aquaculture” really means. Some trends emerged, such as depth (m), distance from shore (nm), and current (m/s) as the most common metrics used to describe offshore sites, but these metrics, especially current, were not necessarily measured or reported. Importantly, many descriptions or uses (ca. 50%) of “offshore aquaculture” were relatively close to shore and/or shallow. Nonetheless, studies that focused on potential ecological impacts of offshore farms, although few (n = 17), tended to report no significant effect. Modeling the probability of a measurable impact based on these studies revealed a “farm ecotone” of ~90 m; beyond this distance, evidence of an environmental impact being extremely unlikely. Although more data is certainly needed to robustly evaluate the biophysical characteristics and levels that drive differences in environmental performance across sites—specifically offshore vs. nearshore—our work does provide a simplified approach and support in elucidating the potential advantages of offshore aquaculture and suggesting preliminary thresholds where higher performance may be expected. Ultimately, such information could provide general points of reference for improved communication and management for stakeholders, regulators, and scientists.
Offshore aquaculture, as it is described now, tends to be closer to shore and/or shallower than may be assumed, but inferences around the described metrics can be used for future research and development. While it might be easier—particularly from a policy perspective—to designate “offshore” in or beyond the EEZ, using a combination of basic metrics, such as distance from shore, depth, and current, can provide more detailed points of reference that are ecologically relevant and perhaps more economically feasible (i.e., closer to shore) (Knapp, 2008; Klinger and Naylor, 2012). Based on our analysis, placing offshore farm structures greater than 90 m away from essential habitats in regions could be used as a starting point for thresholds that minimize the ecological risk of aquaculture development. In fact, this value is comparable to other general, single study recommendations for marine, cage-based farming (Wu et al., 1994; Wu, 1995; Beveridge, 2008). It has also been proposed to place farms at locations with currents faster than 0.05m/s—near equivalent velocity to the lower range (mean current – SD = 0.06m/s) of the non-impact current speeds from our analysis—and depths a minimum of two times that of the net pen to support better water quality (Ladenburg and Sturges, 1999; Belle and Nash, 2008; Beveridge, 2008). These suggested reference points are based on the best available, but limited, information and correspond to a more precautionary approach of reducing any measurable change—statistically significant or otherwise—to the environment. Providing clearer, consistent metrics would help identify and track offshore potential and issues in a more comparable context.
More systematic effort reporting of both physical and ecological parameters related to offshore aquaculture development is needed. Assessing parameters over a multitude of studies can help elucidate ecologically meaningful thresholds, but only if the values are reported and in comparable units. The level of production, including biomass, grow-out period, and feeding rate were some of the most sparse and variable pieces of information in the offshore literature. Yet, these metrics can greatly impact the ecological and economic sustainability of a farm (Domínguez et al., 2001; Bostock et al., 2010). Offshore research interest appears to be growing at a relatively rapid rate, so before any major shifts in investigation and/or expansion occur, we propose studies make sure to document and report (1) production in tons per year, (2) stocking density in kg per m3, (3) number of cages, (4) grow-out period in units of years, and (5) feeding rate in kg per day. Current speed (m/s) is another seemingly critical component to offshore practices (Holmer, 2010; Klinger and Naylor, 2012) that is mentioned in the literature but not always reported numerically. In order to be useful, these fundamental measurements not only need to be part of offshore aquaculture management planning, but also available for comparative assessments.
While nutrient pollution and benthic community impacts are the most heavily studied impacts related to marine aquaculture, other risk factors remain less clear. Quantified vulnerability of wild populations to disease, genetic, and/or invasive risk from offshore farms remains relatively theoretical (Holmer et al., 2008; Phillips and Subasinghe, 2008; Bostock et al., 2010; Holmer, 2010; Klinger and Naylor, 2012; Sumaila et al., 2016). Two studies in our analysis did report significant improvement of offshore cultivated Southern Bluefin tuna and blue mussels (with respect to parasitic load and condition) compared to their nearshore counterparts (Kirchhoff et al., 2011; Brenner et al., 2012). The National Ocean and Atmospheric Administration (NOAA) has also very recently developed an Offshore Mariculture Escapes Genetics Assessment (OMEGA) model. Although promising, there remains an absence of larger, quantitative risk evaluations of offshore expansion. One constraint, as captured in this study, is the narrow scope of measured impacts associated with offshore farming (i.e., nutrient pollution). It is also important to note that considerations beyond ecological impact, such as avoiding use conflict and regulatory restrictions, can be the primary driver for offshore aquaculture location; these factors most likely speak to the diversity of “offshore” definitions. In addition, other potential benefits, such as improved product quality and higher growth rates in open water environments, should be investigated further.
Other limiting factors to offshore risk evaluations include the uncertainty around the locations of offshore aquaculture sites, and information about which countries are actively investing in increased offshore aquaculture development. Offshore aquaculture interest is growing, particularly in the USA and for specific finfish species, but this is not representative of countries or species that are associated with marine production overall. The USA is trying to advance offshore aquaculture in the Gulf of Mexico (Kaiser et al., 2011; NOAA, 2016), but as of 2014 was ranked 17th in global aquaculture production (FAO, 2016). In fact, China produces approximately 60% of all marine aquaculture biomass (FAO, 2016), which may be why they had the most diverse array of species being considered for offshore farming. Indeed, there are over 200 species that are or are being explored for marine aquaculture; most of those are associated with more tropical regions (Froehlich et al., 2016). Finfish do appear as the apparent pioneer taxonomic group for offshore production because of their high potential economic return, but the environmental sustainability of offshore expansion cannot be overlooked (Naylor et al., 2009; Holmer, 2010). As a result, the diverse array of known cultured species and groups that are less carnivorous should continue to be explored in an offshore context.
Our study begins to address the conflating issue of ecologically sustainable aquaculture and offshore practices—which are not synonymous. In other words, classifying a farm as “offshore” does not inherently mean it is sustainable, just as describing “nearshore” aquaculture does not infer unsustainable farming. Although more intuitive for general discussion, a simple distance-based criterion does not ensure environmentally sound outcomes for marine aquaculture. As emphasized in this study, conditions such as current and depth, in addition to distance, undoubtedly contribute to the relative ecological impact on the surrounding environment. While these physical metrics can covary, it is not always the case. Moving forward, offshore research and development thus needs clear evidence-based (empirical and/or model) metric values that correspond to more sustainable offshore practices and are comparable to other aquatic farming approaches.
This research provides, to our knowledge, the most complete evaluation of the term “offshore aquaculture,” and a snapshot of research interest from around the globe. Using terminology to guide the quantitative snapshot of offshore farming revealed common patterns in specific regions, species, and metrics, but no consensus around a common description. Notably, offshore aquaculture, as it is used now, does not necessarily translate to extreme distances from shore and/or deeper depths. We were also able to assess the probability of an ecological effect and match that to more qualitative recommendations. However, our research uncovered the extent of data-gaps and discrepancies in how farm parameters are reported. The issue of limited data makes it inherently difficult to not only comparatively assess offshore aquaculture, but also to set baseline, ecologically meaningful reference points (e.g., minimum distance, depth, and current velocity). In the absence of more and diverse impact studies, aquaculture looks to benefit from more theoretical model-based approaches to begin exploring risk (e.g., OMEGA model); something that is common practice in capture fisheries research. Offshore aquaculture may still be in the earlier stages of development, but the increasing attention surrounding the topic supports the need for setting more rigorous standards of documentation and reporting now, instead of retrospectively.
Author Contributions
HF: scientific idea, data collection, analysis, and writing. AS: data collection and writing. RG: writing. BH: scientific idea, writing, and funding.
Conflict of Interest Statement
The authors declare that the research was conducted in the absence of any commercial or financial relationships that could be construed as a potential conflict of interest.
Acknowledgments
This research was conducted by the Open-Ocean Aquaculture Expert Working Group supported by SNAPP: Science for Nature and People Partnership, a partnership of The Nature Conservancy, the Wildlife Conservation Society, and the National Center for Ecological Analysis and Synthesis (NCEAS; proposal SNP015).
References
Arkema, K. K., Abramson, S. C., and Dewsbury, B. M. (2006). Marine ecosystem-based management: from characterization to implementation. Front. Ecol. Environ. 4, 525–532. doi: 10.1890/1540-9295(2006)4[525:MEMFCT]2.0.CO;2
Belle, S. M., and Nash, C. E. (2008). “Better management practices for net-pen aquaculture,” in Environmental Best Management Practices for Aquaculture, eds C. S. T. Researchessor and J. A. H. A. Consultant (Oxford, UK: Wiley-Blackwell), 261–330.
Bostock, J., McAndrew, B., Richards, R., Jauncey, K., Telfer, T., Lorenzen, K., et al. (2010). Aquaculture: global status and trends. Philos. Trans. R. Soc. Lond. B Biol. Sci. 365, 2897–2912. doi: 10.1098/rstb.2010.0170
Brenner, M., Buchholz, C., Heemken, O., Buck, B. H., and Köhler, A. (2012). Health and growth performance of the blue mussel (Mytilus edulis L.) from two hanging cultivation sites in the German bight: a nearshore—offshore comparison. Aquac. Int. 20, 751–778. doi: 10.1007/s10499-012-9501-0
Bridger, C. J., and Costa-Pierce, B. A. (2002). “53rd gulf and caribbean fisheries institute,” in Sustainable Development of Offshore Aquaculture in the Gulf of Mexico, ed R. L. Creswell (Ft Pierce, FL: Gulf Caribbean Fisheries Inst. Gcfi), 255–265.
Buck, B. H., and Buchholz, C. M. (2005). Response of offshore cultivated Laminaria saccharina to hydrodynamic forcing in the North Sea. Aquaculture 250, 674–691. doi: 10.1016/j.aquaculture.2005.04.062
Burnham, K. P., and Anderson, D. R. (2002). Model Selection and Multimodel Inference: A Practical Information-Theoretic Approach. New York, NY: Springer Science & Business Media.
Domínguez, L., López Calero, G., Vergara Martín, J. M., and Robaina Robaina, L. (2001). A comparative study of sediments under a marine cage farm at Gran Canaria Island (Spain). Preliminary results. Aquaculture 192, 225–231. doi: 10.1016/s0044-8486(00)00450-6
Froehlich, H. E., Gentry, R. R., and Halpern, B. S. (2016). Synthesis and comparative analysis of physiological tolerance and life-history growth traits of marine aquaculture species. Aquaculture 460, 75–82. doi: 10.1016/j.aquaculture.2016.04.018
Froehlich, H. E., Gentry, R. R., Rust, M. B., Grimm, D., and Halpern, B. S. (2017). Public perceptions of aquaculture: evaluating spatiotemporal patterns of sentiment around the world. PLoS ONE 12:e0169281. doi: 10.1371/journal.pone.0169281
Holmer, M. (2010). Environmental issues of fish farming in offshore waters: perspectives, concerns and research needs. Aquac. Environ. Interact. 1, 57–70. doi: 10.3354/aei00007
Holmer, M., Hansen, P. K., Karakassis, I., Borg, J. A., and Schembri, P. J. (2008). “Monitoring of environmental impacts of marine aquaculture,” in Aquaculture in the Ecosystem, eds M. Holmer, K. Black, C. M. Duarte, N. Marbà, and I. Karakassis (New York, NY: Springer), 47–85.
Kaiser, M. J., Snyder, B., and Yu, Y. (2011). A review of the feasibility, costs, and benefits of platform-based open ocean aquaculture in the Gulf of Mexico. Ocean Coast. Manage. 54, 721–730. doi: 10.1016/j.ocecoaman.2011.07.005
Kirchhoff, N. T., Rough, K. M., and Nowak, B. F. (2011). Moving cages further offshore: effects on southern bluefin tuna, T. maccoyii, parasites, health and performance. PLoS ONE 6:e23705. doi: 10.1371/journal.pone.0023705
Klinger, D., and Naylor, R. (2012). Searching for solutions in aquaculture: charting a sustainable course. Annu. Rev. Environ. Resour. 37, 247–276. doi: 10.1146/annurev-environ-021111-161531
Knapp, G. (2008). “Economic potential for US offshore aquaculture: an analytical approach,” in Offshore Aquaculture in the United States: Economic Considerations, Implications and Opportunities, NOAA Technical Memorandum NMFS F/SPO-103, ed M. Rubino (Silver Spring, MD: U.S. Department of Commerce), 161–188.
Ladenburg, C., and Sturges, S. (1999). Potential Offshore Finfish Aquaculture in the State of Washington: Technical Report. Washington State Department of Natural Resources.
NOAA (2016). Fisheries of the Caribbean, Gulf, and South Atlantic; Aquaculture. Washington, DC: National Marine Fisheries Service (NMFS); National Oceanic and Atmospheric Administration (NOAA); Department of Commerce.
Naylor, R. L., Hardy, R. W., Bureau, D. P., Chiu, A., Elliott, M., Farrell, A. P., et al. (2009). Feeding aquaculture in an era of finite resources. Proc. Natl. Acad. Sci. U.S.A. 106, 15103–15110. doi: 10.1073/pnas.0905235106
Ögmundarson, Ó., Holmyard, J., Þórðarson, G., Sigurðsson, F., and Gunnlaugsdóttir, H. (2011). Offshore Aquaculture Farming - Report from the Initial Feasibility Study and Market Requirements for the Innovations from the Project. Reykjavík: Icelandic Food & Biotech.
Phillips, M. J., and Subasinghe, R. P. (2008). “Application of risk analysis to environmental issues in aquaculture,” in Understanding and Applying Risk Analysis in Aquaculture, Fisheries and Aquaculture Technical Paper No. 519, eds M. G. Bondad-Reantaso, J. R. Arthur, and R. P. Subasinghe (Rome: FAO), 101–119.
Price, C., Black, K. D., Hargrave, B. T., and Morris, J. A. Jr. (2015). Marine cage culture and the environment: effects on water quality and primary production. Aquac. Environ. Interact. 6, 151–174. doi: 10.3354/aei00122
RCoreTeam (2015). R: A Language and Environment for Statistical Computing. Vienna: R Foundation Statistical Computing. Available online at: http://www.R-project.org/
Ruiz, J. M., Marco-Méndez, C., and Sánchez-Lizaso, J. L. (2010). Remote influence of off-shore fish farm waste on Mediterranean seagrass (Posidonia oceanica) meadows. Mar. Environ. Res. 69, 118–126. doi: 10.1016/j.marenvres.2009.09.002
Skladany, M., Clausen, R., and Belton, B. (2007). Offshore aquaculture: the frontier of redefining oceanic property. Soc. Nat. Resour. 20, 169–176. doi: 10.1080/08941920601052453
Sturrock, H., Newton, R., Paffrath, S., Bostock, J., Muir, J., Young, J., et al. (2008). Prosepctive Analysis of the Aquaculture Sector in the EU. Seville: Joint Research Center European Comission.
Sumaila, U. R., Bellmann, C., and Tipping, A. (2016). Fishing for the future: an overview of challenges and opportunities. Mar. Policy 69, 173–180. doi: 10.1016/j.marpol.2016.01.003
Twu, S. W., Kao, R. C., and Hwung, H. H. (1986). Aquacultural engineering and simulation on a wave dissipation method for offshore aquaculture areas. Aquac. Eng. 5, 271–286. doi: 10.1016/0144-8609(86)90021-X
Vizzini, S., and Mazzola, A. (2006). The effects of anthropogenic organic matter inputs on stable carbon and nitrogen isotopes in organisms from different trophic levels in a southern Mediterranean coastal area. Sci. Total Environ. 368, 723–731. doi: 10.1016/j.scitotenv.2006.02.001
Watson, R. A., Green, B. S., Tracey, S. R., Farmery, A., and Pitcher, T. J. (2015). Provenance of global seafood. Fish Fish. 17, 585–595. doi: 10.1111/faf.12129
Wu, R. S. S. (1995). The environmental impact of marine fish culture: towards a sustainable future. Mar. Pollut. Bull. 31, 159–166. doi: 10.1016/0025-326X(95)00100-2
Keywords: offshore, open-ocean, aquaculture, sustainable, ecological impact, mariculture
Citation: Froehlich HE, Smith A, Gentry RR and Halpern BS (2017) Offshore Aquaculture: I Know It When I See It. Front. Mar. Sci. 4:154. doi: 10.3389/fmars.2017.00154
Received: 13 October 2016; Accepted: 08 May 2017;
Published: 22 May 2017.
Edited by:
Rob Harcourt, Macquarie University, AustraliaReviewed by:
Marcus Geoffrey Haward, University of Tasmania, AustraliaJames Roger Watson, Stockholm University, Sweden
Copyright © 2017 Froehlich, Smith, Gentry and Halpern. This is an open-access article distributed under the terms of the Creative Commons Attribution License (CC BY). The use, distribution or reproduction in other forums is permitted, provided the original author(s) or licensor are credited and that the original publication in this journal is cited, in accordance with accepted academic practice. No use, distribution or reproduction is permitted which does not comply with these terms.
*Correspondence: Halley E. Froehlich, froehlich@nceas.ucsb.edu