- Division of Cell Biology and Biophysics, School of Biological Sciences, University of Missouri–Kansas City, Kansas City, MO, United States
Magnaporthe oryzae is an agricultural mold that causes disease in rice, resulting in devastating crop losses. Since rice is a world-wide staple food crop, infection by M. oryzae poses a serious global food security threat. Fungicides, including azole antifungals, are used to prevent and combat M. oryzae plant infections. The target of azoles is CYP51, an enzyme localized on the endoplasmic reticulum (ER) and required for fungal ergosterol biosynthesis. However, many basic drug-pathogen interactions, such as how the azole gets past the fungal cell wall and plasma membrane, and is transported to the ER, are not understood. In addition, reduced intracellular accumulation of antifungals has consistently been observed as a drug resistance mechanism in many fungal species. Studying the basic biology of drug-pathogen interactions may elucidate uncharacterized mechanisms of drug resistance and susceptibility in M. oryzae and potentially other related fungal pathogens. We characterized intracellular accumulation of azole drugs in M. oryzae using a radioactively labeled fluconazole uptake assay to gain insight on whether azoles enter the cell by passive diffusion, active transport, or facilitated diffusion. We show that azole accumulation is not ATP-dependent, nor does it rely on a pH-dependent process. Instead there is evidence for azole drug uptake in M. oryzae by a facilitated diffusion mechanism. The uptake system is specific for azole or azole-like compounds and can be modulated depending on cell phase and growth media. In addition, we found that co-treatment of M. oryzae with ‘repurposed’ clorgyline and radio-labeled fluconazole prevented energy-dependent efflux of fluconazole, resulting in an increased intracellular concentration of fluconazole in the fungal cell.
Introduction
The fungal plant pathogen Magnaporthe oryzae is considered to be one of the most dangerous and destructive agricultural molds. Named after the host it infects, M. oryzae causes disease in rice (Oryza sativa) called rice blast, which can infect all parts of the rice plant causing lesions on leaves, stems, seeds, and even roots (Martin-Urdiroz et al., 2016; Yan and Talbot, 2016). Hundreds of millions of people world-wide depend on rice as a staple food crop making rice the most important grain with regard to human diet and nutrition (Harrison, 2002; Thornton and Wills, 2015). Consequently, rice blast poses a serious global food security threat. So great is the potential risk for crop failure or severely reduced crop yield due to rice blast, that M. oryzae has been ranked among the most significant plant pathogens (Talbot, 2003; Thornton and Wills, 2015).
Fungicides, including agricultural azoles such as propiconazole, prothioconazole, tebuconazole, and prochloraz, are used to combat rice blast (Talbot, 2003; Wilson and Talbot, 2009; Edwards and Godley, 2010; Parker et al., 2011, 2013; Kaur et al., 2012; Lehoczki-Krsjak et al., 2013, 2015). Fungicides are utilized as both seed treatment to prevent infection of the seedlings after germination, and also as foliar sprays on mature leaves to prevent infection and spread during the growing season (Couch et al., 2005; Edwards and Godley, 2010; Parker et al., 2011, 2013; Kaur et al., 2012; Lehoczki-Krsjak et al., 2013, 2015).
The target of azole antifungals is the fungal Cyp51, Lanosterol 14α-demethylase. This enzyme is a member of the cytochrome P450 superfamily of enzymes required for ergosterol biosynthesis in fungi.
Drugs that have intracellular targets, such as Cyp51 located on the endoplasmic reticulum (ER), must cross both the fungal cell wall and the plasma membrane and then be transported to its ER destination. It is not clear how azoles are able to do this. It has generally been supposed that azoles enter the cell by passive diffusion, until recent analysis of fluconazole (FLC) uptake in C. albicans and A. fumigatus by our lab argue against a passive diffusion mechanism (Mansfield et al., 2010; Esquivel et al., 2015). Identifying drug uptake mechanisms in fungi opens many new avenues for investigation in basic fungal biology, pathogenesis, and new drug design. Insights into new fungal-specific drug targets, or finding treatment strategies using combinations of existing drugs, is of great importance to control the spread of resistant fungi.
Importantly to our research, M. oryzae shares many characteristics associated with other notable cereal pathogens (Talbot, 2003; Wilson and Talbot, 2009). Advances in understanding the genes and mechanisms that govern the resistance and susceptibility in this species, will help advance our understanding of other related fungal diseases.
In this study, we extended our previously reported radioactively labeled fluconazole accumulation assay to measure cellular accumulation of azole drugs in M. oryzae and determine if azoles enter the cells by passive diffusion, active transport, or a facilitated diffusion mechanism. Consistent with our import experiments on the filamentous human fungal pathogen A. fumigatus, our import experiments with M. oryzae have shown that azole drugs do not accumulate in the fungal cell solely by passive diffusion or ATP-dependent active transport. Instead there is evidence for azole import by a facilitated diffusion mechanism such as a protein carrier or channel.
In addition to uptake analysis, we used 3H-FLC to measure glucose-stimulated and glucose-independent azole efflux over time from M. oryzae. The M. oryzae strain used in this study is the first plant pathogenic fungus to be fully sequenced allowing a direct examination of its genome (Dean et al., 2005). Compared to yeast species such as Saccharomyces cerevisiae, Candida albicans, Cryptococcus neoformans, Candida glabrata, and Candida krusei, the filamentous fungus M. oryzae contains an unusually high number of genes encoding predicted membrane transporters. This includes at least 50 ATP-Binding Cassette (ABC) and at least 250 Major Facilitator Superfamily (MFS) transporters (Coleman and Mylonakis, 2009; Kim et al., 2013). The majority of these transporter genes are still uncharacterized to date (Kovalchuk and Driessen, 2010; Kim et al., 2013).
Our 3H-FLC efflux data showed evidence for a glucose-stimulated azole efflux mechanism in M. oryzae, which would be consistent with an ABC superfamily transporter activity.
For a final efflux-related analysis, we evaluated the effects of combination treatment of M. oryzae with 3H-FLC and clorgyline, a drug once used as an antidepressant that has recently been shown to have efflux inhibitor properties (Youdim, 1975; Holmes et al., 2012). These results demonstrate the potential usefulness of combination drug therapies and the idea of repurposing archived compounds for alternative uses as the threat of resistance to current fungal treatments emerges.
Materials and Methods
Strains, Media, Materials, and Strain Preparation
The M. oryzae wild-type, sequenced strain 8958 (70-15), was used for all azole import experiments. M. oryzae cultures were routinely grown and maintained in either liquid yeast nitrogen base, ammonium sulfate and dextrose (YAD) medium (1.7 g yeast nitrogen base without amino acids or ammonium sulfate, 5 g ammonium sulfate and 20 g glucose per liter) or on oatmeal agar plates with 20 g glucose per liter, at room temperature (27°C). Conidia were harvested from 7-day old agar plate cultures by pipetting 0.01% Tween 20/water directly onto sporulating plates and loosening conidia using a sterilized glass spreader. The harvested solution was allowed to sit for 10–15 min to allow mycelia to settle, while the conidia remained suspended.
To propagate M. oryzae, 200 μL of the supernatant from the harvested conidial suspension was either directly inoculated into 5 ml liquid YAD media with glucose and grown in 50 mL conical tubes in a room temperature shaking at 180 rpm, or plated on oatmeal agar plates. For storage, oatmeal agar plates were overlaid with 3–5 Whatman filter paper disks before inoculating with conidial suspension. After 7–10 days of growth, filter paper disks covered with a lawn of M. oryzae were collected, desiccated in a Tupperware container with Drierite desiccant stones (W.A. Hammond Drierite Co. LTD. Xenia, OH, United States), and stored at -20°C.
Medium components, plastic ware, general chemicals, and unlabeled drugs used for competition were obtained from Fisher Scientific (Pittsburg, PA, United States), or Sigma–Aldrich (St. Louis, MO, United States).
M. oryzae E-Test Susceptibility Testing to Common Antifungals
E-test strips (bioMérieux, United States) were used to test M. oryzae susceptibility and determine the minimum inhibitory concentration (MIC) to the antifungals fluconazole (FLC), ketoconazole (KTC), itraconazole (ITC), posaconazole (POS) voriconazole (VRC), caspofungin (CFG), amphotericin B (AMB), and 5-flucytosine (5FC). One side of the plastic E-test strip is calibrated with MIC values of the drug in μg/ml. The drug-gradient on the strip covers 15 twofold dilutions.
Yeast extract Peptone Dextrose, or YEPD or YPD (10 g yeast extract, 20 g peptone and 20 g glucose) plates were inoculated with a lawn of M. oryzae conidia and allowed to dry. A single E-test strip was placed on each inoculated plate and kept at 27°C for 96 h with daily monitoring. The MIC was determined based on the drug concentration on the E-test strip in which the zone of inhibition, or ellipse of non-growth occurred.
Radioactively Labeled Azole Import by M. oryzae
Radioactively labeled FLC (3H-FLC), (481 GBa/mmol, 13 Ci/mmol, 1 μCi/μL; 77 μM FLC) was custom synthesized by Amersham Biosciences. The drug concentration used during the import assay was well below the MIC for the strain (M. oryzae FLC MIC >32 μg/ml).
To directly measure azole intracellular accumulation in the fungal cell, we used 3H-FLC in our drug uptake assay designed for the filamentous morphology of M. oryzae. Studies of plant-pathogen interactions have shown that fungal growth associated with plant disease shows biofilm-like properties including robust hyphal networks and heterogeneous fungal layers (Villa et al., 2017). Unless specifically noted otherwise, 5 ml YAD medium with 2% glucose in 50 mL conical tubes was inoculated with M. oryzae conidia and grown at 27°C, 180 rpm shaking, for 48 h at which point they were filamentous mycelial masses or fungal balls of hyphae, approximately 3 mm in diameter. The mycelial masses were transferred to 2 mL microcentrifuge tubes and washed by centrifugation and resuspension in fresh media three times using YNB complete (1.7 g yeast nitrogen base without amino acids or ammonium sulfate, 5 g ammonium sulfate per liter, pH 5.0) without glucose unless specifically noted.
After the washing, the fungal ball pellet was transferred to 14 mL round bottom tubes containing 1 mL of YNB for a 2 h glucose-deprived (starvation) de-energizing period. The glucose starvation was done to keep the cells in a de-energized state. The de-energized cells showed no further growth for the extent of the import assay, as determined by dry weight at the conclusion of the assay. After the glucose starvation period, the radiolabeled drug treatment reaction mixes were prepared consisting of 1 mL of YNB containing no glucose, fungal balls, and 25 μL of diluted 3H-FLC (freshly diluted 1/100X from stock). The resulting final 3H-FLC concentration is 19.25 nM (5.89 ng/ml). The M. oryzae strain (70–15) has an MIC of >32 μg/ml to FLC. Thus, the azole concentration used for the import assay is not expected to have any effect on cell viability.
After 24 h incubation with 3H-FLC, the reaction was stopped with a 5 ml stop solution (YNB +20 mM [6 mg/L] unlabeled FLC) added to each 14 mL round bottom tube sample. The samples were filtered by vacuum over pre-weighed and wetted glass fiber filters (24 mm GF/C; Whatman; Kent, United Kingdom). The samples on the filters were rinsed with 5 ml stop solution to remove non-specific, cell surface binding of 3H-FLC. The filters with fungal balls were either allowed to dry for 24–48 h or were baked in a drying oven for 15 min at 95°C. Each dried filter containing fungal balls was then re-weighed to obtain the dry mass of each fungal sample. The filters were finally transferred to 5 mL scintillation vials containing 3 ml of scintillation cocktail (Ecoscint XR, National Diagnostics, Atlanta, GA, United States). Radioactivity associated with the fungal sample on each filter was measured in a liquid scintillation analyzer (Beckman Coulter, LS 6500 multipurpose scintillation counter).
Radioactivity values are expressed as 3H-FLC drug accumulation per dry weight of the sample. Results were calculated as CPM/mg of mycelial mass. While absolute CPM values varied between experiments, relative values of import differences between samples remained consistent and repeatable.
Screening of Factors That Modulate 3H-FLC Import
Further studies were done to determine how FLC import is affected by changes in the growth or drug incubation conditions.
Heat Inactivated Cells
Fluconazole uptake was measured in cells that had been exposed to high heat (95°C) for 30–40 min. Samples were processed according to the standard 3H-FLC import protocol with the exception that the heat-killing step was performed immediately prior to 3H-FLC treatment. Heat killed samples were treated with 3H-FLC and analyzed identically to the unheated, control cell samples. The results were compared with live M. oryzae data. Heat inactivated samples were used as a control for baseline drug accumulation and non-specific cell surface binding in all testing conditions unless otherwise noted.
Other Methods of Cell Inactivation
3H-FLC accumulation was measured after 24 h in samples that were exposed to various inactivation methods. Methods of cell inactivation included treatment with high concentrations of AMB (8 μg/ml), Qiagen RLT Lysis solution (600 μL), and CFG (16 μg/ml). Heat killing as described above was found to be the most reliable at reducing viable cell counts to less than 1%. And so this method of inactivation was used as a no-import, baseline control, although the other methods of inactivation were useful to confirm that import is reduced in inactivated cells as opposed to being an artifact of heat treatment.
Energy Depletion
To determine whether 3H-FLC import was energy-dependent, mycelial balls were washed three times from overnight media and resuspended in glucose-free YNB media. Samples were glucose starved for 2 h in this media and considered to be de-energized after this time. Following the de-energization period, the cells were treated with 3H-FLC for 24 h in glucose-depleted media and their 3H-FLC accumulation was compared to cells that were treated with 3H-FLC for 24 h in media containing 2% glucose.
pH
Cells were incubated with 3H-FLC in YNB without supplementation (pH 5), with no pH adjustment, or with YNB medium adjusted to pH 4 or 7 with a 0.1 M citric acid and 0.2 M Na2HPO4 mixed buffer stock solution. Increasing the ratio of the citric acid to Na2HPO4 to lower pH and increasing the ratio of Na2HPO4 to citric acid solution to increase pH. All samples were processed after 24 h as described above.
Growth Media
Magnaporthe oryzae liquid cultures were grown from conidia to mature mycelial masses (48 h) in either Complete Supplemental Medium or CSM (0.75 g CSM, 1.7 g yeast nitrogen base without amino acids or ammonium sulfate, 5 g ammonium sulfate, 20 g glucose per liter); YAD Medium (1.7 g yeast nitrogen base without amino acids or ammonium sulfate, 5 g ammonium sulfate and 20 g glucose per liter); or YPD as described above. The mycelial masses were then washed and treated with 3H-FLC in YNB for 24 h as described for the standard import assay.
CSM is a Complete Supplemental Mixture that is considered synthetic defined, complete medium, containing the chemically defined components Yeast Nitrogen Base (YNB), Ammonium Sulfate (AS) and a carbon source, glucose. It also contains a complete amino acid supplement mixture and so is non-selective.
Yeast nitrogen base, ammonium sulfate and dextrose is considered a synthetic defined, minimal medium, containing the chemically defined components YNB, AS and glucose, but without any supplemented amino acids so only prototroph strains can grow in this media.
Yeast extract Peptone Dextrose, also called YEPD, is considered an undefined, non-selective, rich medium. YPD contains Yeast extract, a complex nutrient base derived from killed yeast cells; Peptone, an enzymatic digest of animal protein that contains nitrogen and a high peptone and amino acid content, and glucose. This medium provides an excess of amino acids, nucleotide precursors, vitamins, and essential metabolites.
Exponential vs. Stationary Phase Uptake of 3H-FLC
Conidia were grown at 27°C in a shaking incubator at 180 rpm in YAD complete media with glucose for either the standard 48 h (exponentially growing) or for 72 h or 96 h (stationary phase). These 2, 3, or 4 days old fungal balls were then treated with 3H-FLC for 24 h as described previously.
Competitive Inhibition of Azole Import
To determine if azoles enter the cell by a specific or non-specific protein carrier, or by passive diffusion, we treated M. oryzae fungal balls with 3H-FLC as well as co-treatment with unlabeled compounds that could be potential competitive inhibitors. Samples were processed as described above with our 3H-FLC import assay, with the additional compound added to the sample at 1.95 μM (100X molar excess of the labeled FLC). 3H-FLC uptake was measured as usual after 24 h incubation with 3H-FLC and competitor.
Decreased 3H-FLC uptake in the presence of unlabeled FLC as well as other unlabeled competitor suggests that both drugs use the same transporter. Non-radiolabeled drugs used for import competition experiments included: amphotericin B (AMB), caspofungin (CFG), fenpropimorph (FEN), fluconazole (FLC), itraconazole (ITC), ketoconazole (KTC), metconazole (MET), tebuconazole (TBZ), terbinafine (TRB), and 1-triphenylmethyl imidazole (1-TRI).
Azole Efflux from M. oryzae
Efflux Kinetics
Magnaporthe oryzae mycelial masses of hyphal cells were preloaded with 3H-FLC by incubating samples with the radiolabeled FLC at 19.5 nM in the absence of glucose for 24 h to allow maximum intracellular accumulation with no energy-dependent glucose. After the 24 h incubation, the mycelial masses were washed and resuspended into fresh YNB media, and the amount of labeled drug remaining in the cells over time was determined at 4, 8, and 16 h. Efflux was evaluated in both glucose-energized (2% glucose) and de-energized (glucose-starved) cells.
Efflux Inhibition by Clorgyline
To test the effect of Clorgyline as a possible inhibitor of energy-dependent efflux in M. oryzae, we treated energized cells (2% glucose, efflux active) with either 3H-FLC alone (19.25 nM) or 3H-FLC with clorgyline [N-Methyl-N-propargyl-3-(2,4-dichlorophenoxy)propylamine hydrochloride] (233 μM) and compared the results to 3H-FLC treatment in de-energized (glucose-starved, efflux inactive) cells. 3H-FLC accumulation was measured in all conditions after 20 h.
Statistical Analysis
Differences between sets of samples were evaluated by an unpaired two-tailed Student’s t-test. A P-value of < 0.05 was considered significant.
Results
M. oryzae Minimum Inhibitory Concentrations (MIC) to Common Antifungals
E-test strips were used to establish M. oryzae susceptibility to common medical antifungals belonging to different classes of drugs and determine if a study using medical azoles was relevant in M. oryzae. The MICs were reported in Figure 1 and Table 1. The drugs tested included the medical azoles FLC, ITC, KTC, POS, and VRC, the echinocandin CFG, the polyene AMB, and the RNA and DNA synthesis inhibitor 5FC.
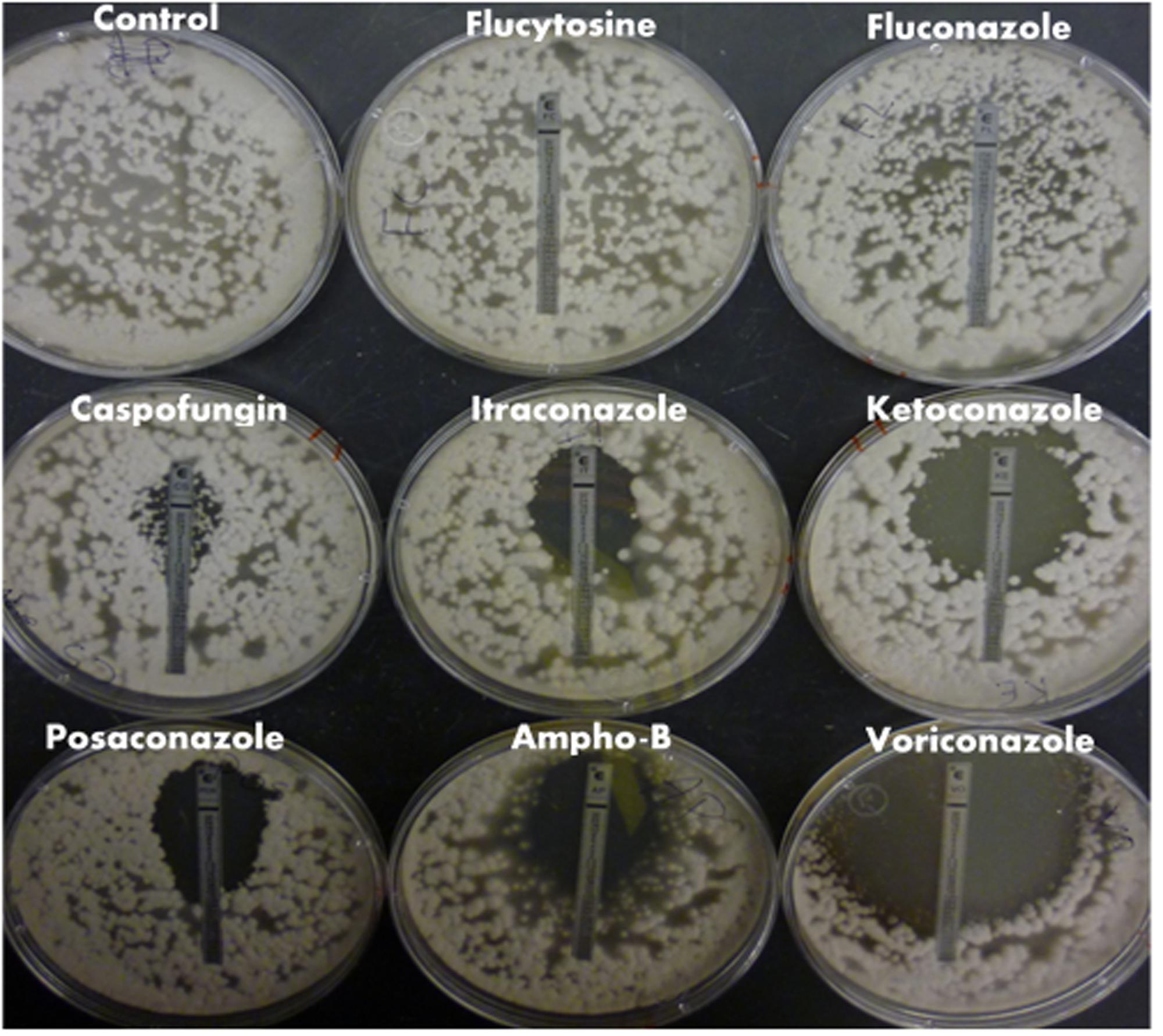
FIGURE 1. Magnaporthe oryzae drug susceptibility to common classes of medical antifungals. E-test strips were used to test M. oryzae susceptibility and determine the MIC to common antifungals belonging to different classes of drug. Plates were allowed to grow at 27°C for 96 h. The MIC was determined based on the ellipse of non-growth intersecting with the drug gradient marked on the test strip.
Magnaporthe oryzae did not show susceptibility to 5FC or FLC at the drug concentrations tested, and although there was a ‘phantom’ zone of clearing around the CFG E-test, there were enough CFG-resistant colonies in the clearing for this strain to be interpreted as resistant at the concentrations tested. There was measurable susceptibility of M. oryzae to the other drugs tested (Figure 1 and Table 1).
Factors That Modulate 3H-FLC Import
Recently, radioactively labeled drugs were used to analyze azole uptake in the human pathogenic yeast Candida albicans and Cryptococcus neoformans, the model yeast Saccharomyces cerevisiae, and the human pathogenic mold A. fumigatus (Mansfield et al., 2010; Esquivel et al., 2015). Here we have adapted the assay used in the previous studies for an initial characterization of azole uptake and efflux in the plant pathogenic mold M. oryzae. Radioactive FLC is used in these import studies because of its availability. While the MIC analysis (Figure 1 and Table 1) indicates that FLC is not effective against M. oryzae, the results presented indicate that the drug is imported into the cell as well as other azoles.
3H-FLC Import in Inactivated Cells
Labeled FLC was used in the import assay to characterize azole import. Cell inactivation by heat, AMB treatment, and lysis solution each reduced azole import significantly (Figure 2), suggesting drug uptake and accumulation requires the maintenance of an intact and functional cell membrane. Inactivation by high heat incubation before 3H-FLC treatment was the chosen method for creating a baseline control sample because heat inactivation reliably and reproducibly reduced intracellular 3H-FLC to very low radioactive counts. Heat inactivation was therefore used in subsequent experiments as a measure of background, or non-specific drug binding.
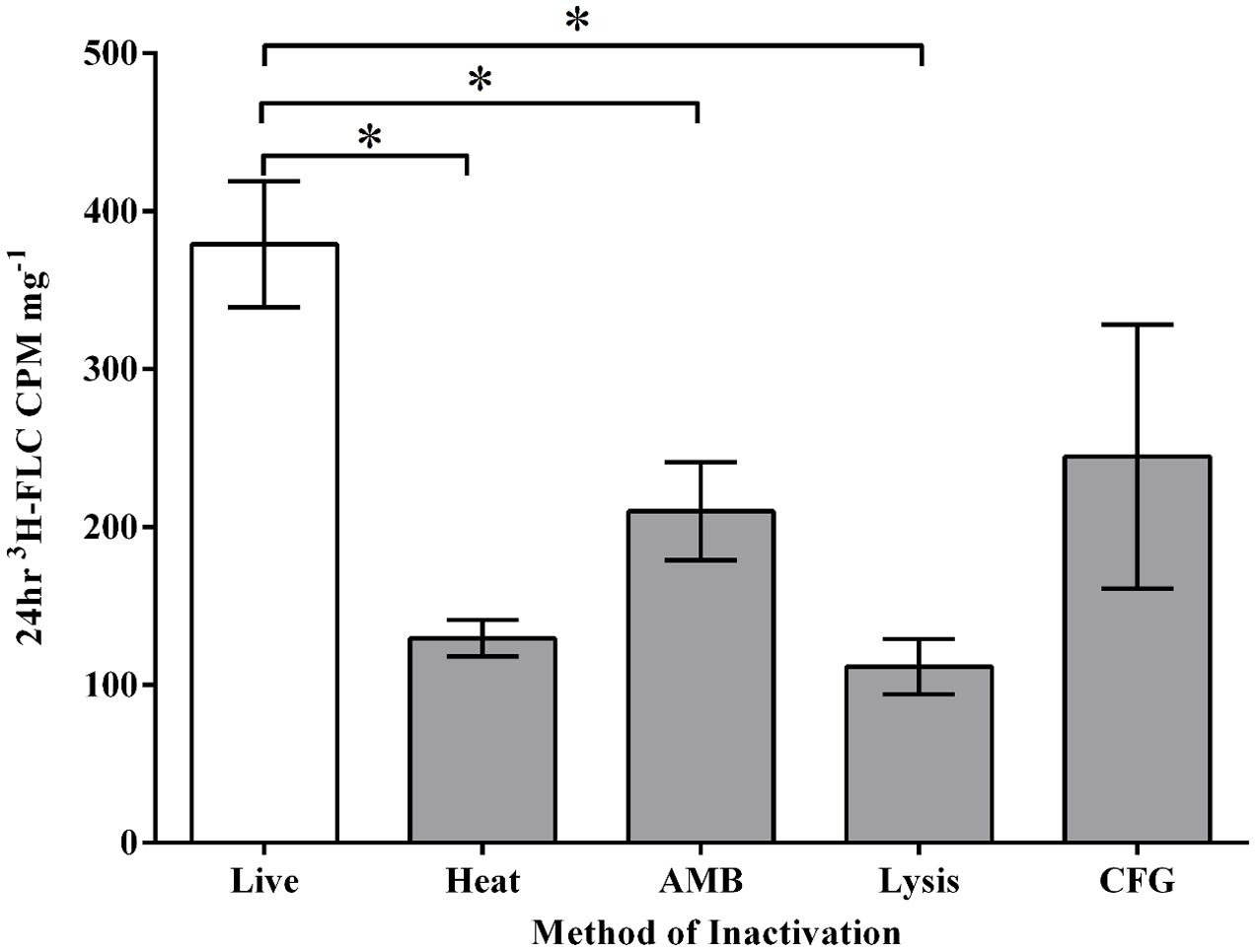
FIGURE 2. Magnaporthe oryzae inactivation methods before 3H-FLC treatment. 3H-FLC accumulation was measured after 24 h in samples that were exposed to various inactivation methods. (Live): no inactivation, (HK): 95°C for 30 min, (AMB): 8 μg/ml of amphotericin B, (Lysis): 600 μL Qiagen RLT buffer, (CFG): 16 μg/ml of caspofungin. Error bars represent standard deviation for each condition. Asterisk indicates a statistical significance of P < 0.05.
The reduced 3H-FLC import due to heavy AMB treatment also gives support to the idea that the uptake of FLC is at least partially dependent on the plasma membrane, perhaps via a protein channel or cell membrane transporter. Disruption of the membrane by AMB treatment above MIC levels (8 μg/ml) partially but significantly inhibits azole uptake.
Caspofungin treatment at 16 μg/ml reduced FLC uptake into the cell but not significantly, suggesting that cell wall disruption does not influence azole import in the way that plasma membrane disruption does. However, the CFG-treated cells did show high import variability and a trend toward reduced azole import so the role of the cell wall for azole import remains to be elucidated.
Energy-Dependent Active Transport
To determine if M. oryzae actively takes up 3H-FLC by an energy-dependent active transport mechanism, we analyzed 3H-FLC accumulation in the presence or absence of glucose as an energy source for ATP-coupled transporters (ABC transporters). The cells for each sample were first de-energized by glucose starvation in glucose-depleted media prior to 3H-FLC incubation to deplete cellular ATP. The 3H-FLC incubation period was then carried out in either glucose replete or glucose deplete conditions and the results were compared for quantifying the effect of energy-dependence during import (Figure 3A).
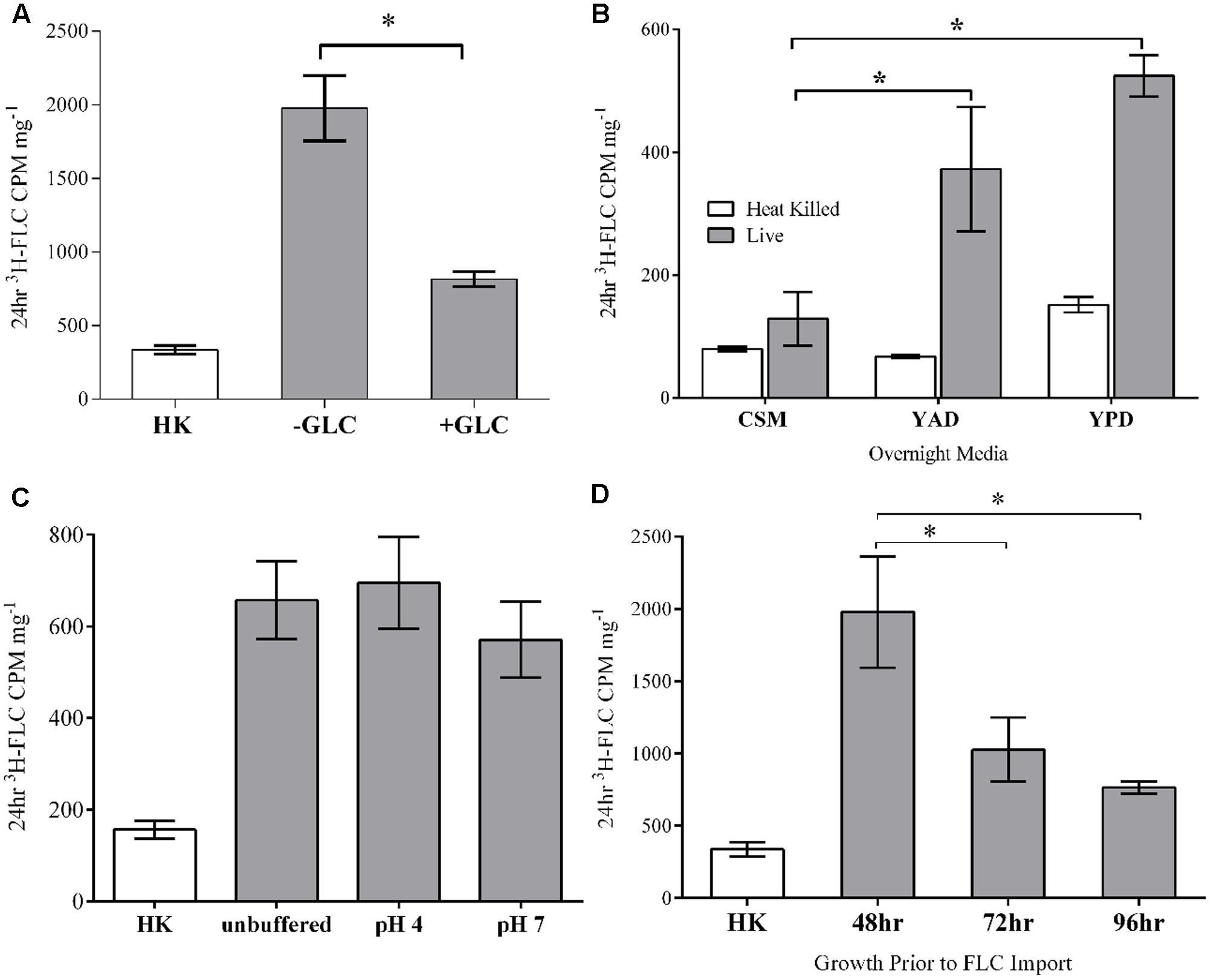
FIGURE 3. Effects of glucose, media, pH and cell phase on FLC import. (A) Glucose: Cells were de-energized by glucose-depleted media (–GLC) compared to cells in the presence of glucose (+GLC) and measured for FLC accumulation. (B) Growth Media: Cells were grown from conidia to mycelial masses for 48 h in either CSM complete, YAD, or YPD media. (C) pH: 3H-FLC was imported into M. oryzae in unbuffered media or at pH 4 and 7 in 100 mM citric acid buffers. There were no statistically significant differences between import at the three conditions. (D) Phase: Cells were grown in shaking liquid media for 48, 72, or 96 h and then treated with 3H-FLC for 24 h. For each panel: Error bars represent standard deviation for each condition. Asterisks indicate a statistical significance of P < 0.05 between the two conditions connected by brackets. HK, heat killed control. Statistical differences to HK are not shown.
3H-FLC accumulated in both de-energized (glucose-starved) and energized (glucose-replete) cells, with a significantly higher intracellular concentration in the de-energized cells. A mechanism of drug transport across the cell membrane that does not require energy, such as the de-energized sample, argues against ATP-dependent active import of FLC in M. oryzae.
The significant reduction of intracellular 3H-FLC concentration in glucose-energized samples, compared to de-energized samples, is most likely the result of activation of ATP-dependent efflux pumps for which azoles have been identified as substrates in many fungi. For subsequent experiments, we used de-energized (glucose-starved) cells to focus solely on drug uptake and eliminate efflux mechanisms.
Growth Media
Magnaporthe oryzae was grown from conidia to mature mycelial masses in either CSM, YAD, or YPD media and then all samples were washed and resuspended in YNB medium and the 3H-FLC accumulation assay was started. 3H-FLC accumulation was compared between the samples that had previously been grown in the different media (Figure 3B).
Cells that were grown from conidia to mycelial balls in CSM took up the least amount of 3H-FLC after 24 h treatment in the assay. Cells initially grown to mycelial balls in YPD media took up the most 3H-FLCin the assay. However, the baseline control was also slightly higher in the YPD-grown cells as well. Cells initially grown in YAD took up an intermediate level of 3H-FLC in the assay, but surprisingly, uptake was more similar to cells from YPD media compared to the CSM. Both YAD and YPD-grown samples had significantly higher import compared to CSM-grown cells, which had near baseline levels of import, even though the 3H-FLC treatment and import assay for all samples was performed identically.
The data suggest some alteration to, or adaptation of, the cell acquired from the different media that affect FLC uptake and accumulation. The mechanism behind this variation is not known and could be a complex mixture of differences in protein synthesis, lipid storage, transcriptional activity, and other metabolic activities. An examination of the effects of different media on cell wall and cell membrane composition and function would be useful to better understand this phenomenon.
Fungal cell environmental adaptations that prevent or enhance azole uptake would be an important aspect for drug resistance analysis. This differential azole uptake between the samples argues against passive diffusion entry into the cell.
pH
To determine if 3H-FLC import is pH dependent or affected by a proton gradient, we measured 3H-FLC accumulation in unbuffered YNB media, or at pH 4 or 7 using a 0.1 M citric acid and 0.2 M Na2HPO4 mixed buffer (Figure 3C). There was significant 3H-FLC accumulation at all pHs tested, but with no statistically significant difference between the different pHs. This indicates that 3H-FLC import is not pH dependent, or at least not constrained to a specific pH.
Stationary vs. Exponential Cells
Samples grown for the standard 48 h before being incubated with 3H-FLC for uptake analysis were compared to samples grown for 72 or 96 h before drug treatment. Figure 3D shows that the exponentially growing (48 h) samples accumulated significantly more 3H-FLC than the stationary phase (72 and 96 h) samples. The fact that there is differential uptake of 3H-FLC between the samples of different age, stage of hyphal growth, or metabolic activity, would not be observed with passive diffusion and provides evidence for a facilitated transport mechanism of FLC import.
Competition for 3H-FLC Import in M. oryzae with Azoles and Other Compounds
To determine whether all azoles use the same transporter or family of transporters in M. oryzae, non- labeled azoles were tested for competition against labeled FLC (Figure 4 and Tables 2, 3). The concentration of all competitors was 1.95 μM (100x molar excess to 3H-FLC), which is well below inhibitory drug treatment concentrations.
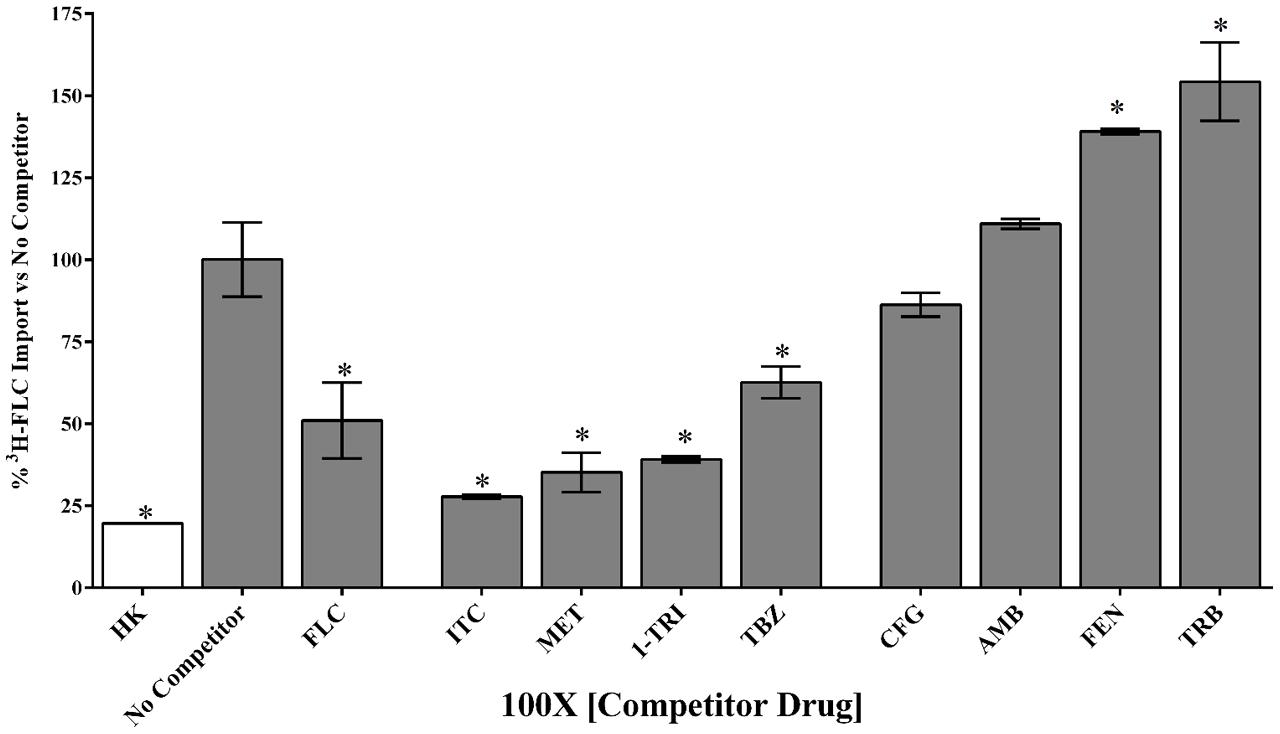
FIGURE 4. Competition for 3H-FLC import in M. oryzae. Unlabeled compounds were tested for competition at 1.95 μM (100x molar excess to 3H-FLC) during simultaneous treatment with 3H-FLC. 3H-FLC accumulation was measured after 24 h incubation with competitors. Asterisks indicate compounds that showed significantly different 3H-FLC accumulation of P < 0.05 from the No Competitor control. Error bars represent standard deviation of biological triplicates for each condition. Drug abbreviations are described in section “Materials and Methods.”
Uptake can clearly be modulated by a variety of pharmacologic agents that can be described as compounds that competed with FLC for uptake, compounds that caused no effect on FLC uptake, and compounds that might stimulate FLC uptake.
Competitive inhibitors, indicated by a significant reduction of the 3H-FLC accumulation to baseline levels, included the medically important azoles (FLC, ITC) as well as agriculturally important azoles (MET, TBZ). 1-TRI, a clotrimazole analog, also competed with FLC for import. Competitive inhibition of 3H-FLC import by structural analogs- namely, other azoles is a strong argument against passive diffusion and in favor of facilitated diffusion, possibly via a protein channel.
Other compounds were also tested for competition against 3H-FLC uptake including common non-azole antifungals (Figure 4 and Tables 2, 3). Non-azole compounds such as AMB and CFG, did not compete with 3H-FLC for import into M. oryzae. The antifungals TRB and FEN also did not compete with 3H-FLC uptake and may even stimulate 3H-FLC accumulation (Figure 4).
The results of these competition experiments indicate transport specificity for certain chemical structures. The structure of FLC and the compounds tested in this assay are shown in Tables 2, 3. FLC has two 5-membered triazole rings containing 3 nitrogen atoms, and a 6-member halogenated benzene ring. Previous screens of moieties important for azole import in C. albicans and A. fumigatus are consistent with this result in M. oryzae (37, 38). Taken together, analyses in C. albicans, A. fumigatus, and now M. oryzae suggests that to compete for FLC import, a compound requires a 5-membered ring with two (imidazole) or three (triazole) nitrogen atoms, in addition to a halogenated 6-membered ring with the halogen in position 1 or 3, but not necessarily both positions (Mansfield et al., 2010; Esquivel et al., 2015). The only exception to this has been the competition of 1-TRI, which has a five membered imidazole ring as well as three 6 membered rings, but is not halogenated.
Efflux of 3H-FLC from Preloaded Cells
Drug accumulation in a fungal cell can be thought of as the net effect of uptake and efflux mechanisms. The conditions of our 3H-FLC import assay remove the energy dependent efflux component so that we can focus on drug entry into the cell. Conversely, Figure 5 demonstrates efflux of FLC from M. oryzae cells. The cells were preloaded with 3H-FLC at 19.5 nM for 24 h following the standard import assay protocol and then were washed and resuspended in fresh media with and without glucose so as to see the effect of energy-dependent efflux on intracellular 3H-FLC concentration. The amount of labeled drug associated with the cells was determined at 4, 8, and 16 h after being transferred to fresh media. Efflux was evaluated in both glucose-energized (gray line with squares) and de-energized (glucose starved) (black line with circles) cells.
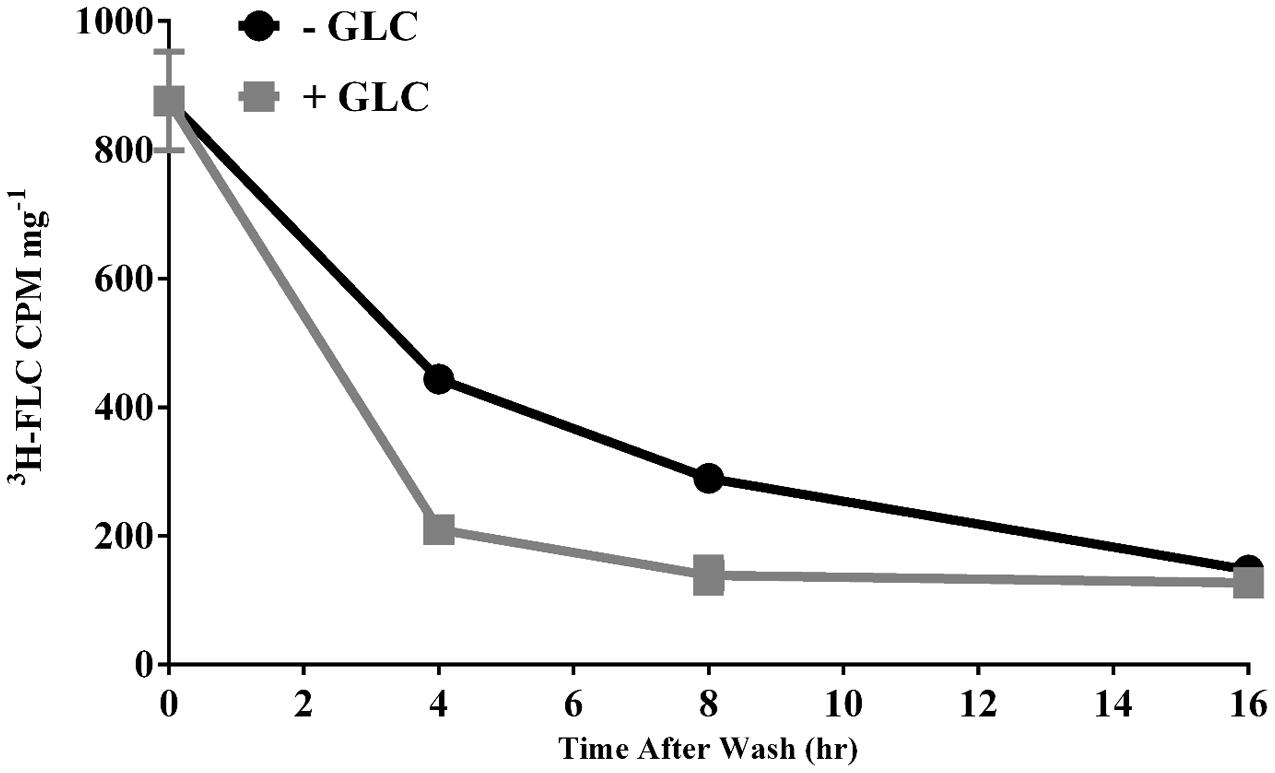
FIGURE 5. Efflux of 3H-FLC from preloaded cells. M. oryzae mycelial cells were preloaded with 19.25 nM 3H-FLC for 24 h. The cells were washed with YNB and placed in either de-energized (glucose free) (solid black line with circles) or a 2% glucose-energized (gray line with squares) media for 4, 8, and 16 h to measure the efflux of 3H-FLC. Error bars represent standard deviation for each condition. Most error bars are hidden by the symbols.
The difference in rate of efflux between energized and de-energized cells at 4 and 8 h was significantly different (Figure 5). By 16 h, most of the 3H-FLC was exported from the cells in both energized and de-energized conditions. The samples incubated in the presence of glucose show faster export of labeled drug, consistent with the idea that membrane efflux pumps require energy and that glucose starvation limited the energy and thus the efflux pump activity.
Clorgyline as a Potential Efflux Inhibitor
Development of a new drug structure and a new mechanism of action would be a valuable addition to our antifungal repertoire; particularly against resistant fungal infections. However, the cost, time and knowledge required to design and develop a brand new compound, as well as testing and approval required before use in plants, animals or humans is very prohibitive. An alternative approach would be the repurposing, or modification of existing drugs, to expedite the drug development process.
Clorgyline is a monoamine oxidase-A inhibitor (MAOI) that has been used for decades as a clinical antidepressant (Fowler et al., 1981; Tipton, 1997). As with many MAOIs, side effects include a hypersensitivity to tyramine-containing foods (especially cheese), and this negative dietary interaction has led to improvements and drug alternatives so that clorgyline use has markedly declined for treatment of depressive illnesses (Fowler et al., 1981; Tipton, 1997).
However, clorgyline remains in the Library of Pharmacologically Active Compounds (LOPAC), which is a collection of compounds, marketed drugs and pharmaceutically relevant structures annotated with biological activities. These compounds can be purchased pre-solubilized, normalized, easily resupplied, and ready-to-use for studies on repurposing applications (Sigma–Aldrich).
Clorgyline has recently been identified in a screen as an inhibitor of two C. albicans ABC efflux pumps, CaCdr1p and CaCdr2p, as well as reversing FLC resistance in S. cerevisiae strains expressing ABC transporters (Holmes et al., 2012). To examine the effect of clorgyline as a possible inhibitor of energy-dependent efflux in M. oryzae, we treated energized (efflux active) cells with either 3H-FLC alone or 3H-FLC with clorgyline and compared the results to 3H-FLC treatment in de-energized (efflux inactive) cells (Figure 6).
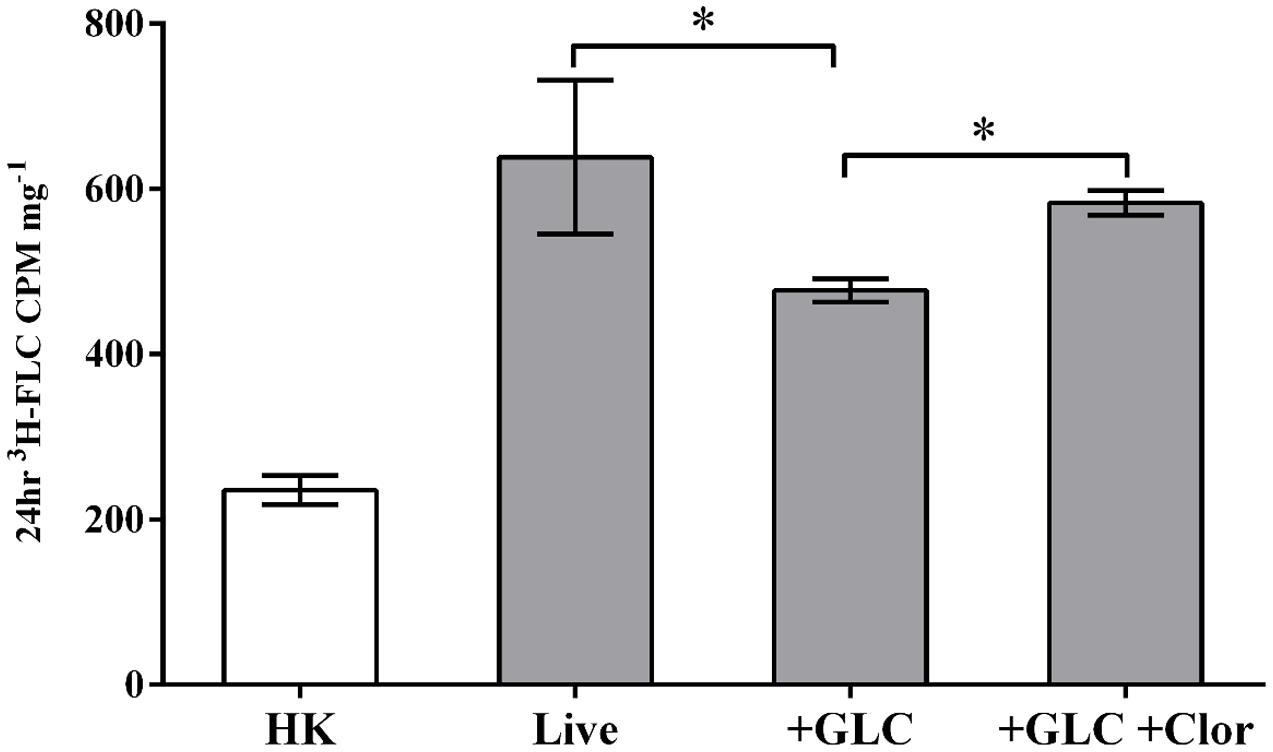
FIGURE 6. Clorgyline as a potential efflux inhibitor. Energized cells (2% glucose, efflux active) were treated with either 3H-FLC alone (+GLC) or 3H-FLC with clorgyline (233 μM) (+GLC +Clor) and the results were compared to 3H-FLC treatment in de-energized (glucose-starved, efflux inactive) cells (Live). 3H-FLC accumulation was measured after 20 h in all conditions. Asterisks indicate 3H-FLC accumulation that is significantly different (P < 0.05) between the two conditions connected by brackets. There was no significant difference in 3H-FLC accumulation between Live and +GLC +Clor. 3H-FLC accumulation in HK samples was significantly lower than all other conditions. Error bars represent standard deviation of biological triplicates for each condition.
Consistent with Figure 3A, 3H-FLC uptake was observed in both de-energized and energized cells, with the energized cells showing significantly reduced intracellular 3H-FLC concentration compared to de-energized cells (Figure 6). The reduced azole accumulation in the energized cells is most likely the result of activation of energy-dependent efflux pumps transporting the 3H-FLC out of the cell. However, when the energized, efflux-active cells were treated with clorgyline, there was significantly increased intracellular 3H-FLC accumulation, indicating energy-dependent efflux was at least partially prevented by the addition of clorgyline.
Discussion
There is a continual emergence of fungicide-resistant pathogens in clinical and agricultural isolates (Fleming et al., 2002). Understanding even the most basic cellular processes and epidemiology of these isolates is imperative to prevent their spread, determine treatment and prevention strategies, optimize future drug design, and to predict future evolution of resistance (Ashkani et al., 2011).
Many fungi with intrinsic resistance to antifungal agents already exist in our environment (36). We illustrated this with E-test susceptibility testing of M. oryzae, which displayed FLC and CFG resistance (Figure 1). Most filamentous fungal species and molds are intrinsically resistant to FLC since they possess multiple target CYP51 paralogs: two in M. oryzae, A. fumigatus, and A. nidulans, and three in A. flavus and species of Fusarium (Lepesheva and Waterman, 2007). This CYP51 redundancy allows for slight changes to occur in the active site of one or all Cyp51 copies that affects the binding affinity to azoles (Hawkins et al., 2014). The structure of FLC in particular allows multiple Cyp51 active site binding conformations that are weak or transitory so that there is incomplete inhibition of the Cyp51 target enzyme compared to other azoles that have stronger Cyp51 binding affinity (Fleming et al., 2002; Mellado et al., 2005; Cools et al., 2010; Warrilow et al., 2010; Parker et al., 2011; Yan et al., 2011; Hawkins et al., 2014).
Regardless, the E-tests confirmed that even azole drugs used to treat human fungal pathogens are taken up by the plant pathogen M. oryzae as evidence by growth inhibition seen with the medical azoles ITC, KTC, POS, and VRC. Overall, the susceptibility and resistance patterns illustrated by the E-tests suggest a common mechanism of action of azoles on all fungal species, including the requirement for entry into the cell, passing through the cell wall and plasma membrane. This may be the first time reporting MICs of medical antifungals on a distinctly plant pathogen.
In this work, we have begun to analyze potential molecular mechanisms of azole drug resistance in the filamentous plant pathogen M. oryzae by characterizing azole import into the fungal cell under a variety of environmental conditions, as well as azole efflux from the cell, using radioactively labeled FLC. Azoles must enter the fungal cell in order to inhibit the intracellular Cyp51 target enzyme. Therefore reduced or modified drug import may help to explain why some pathogenic fungi are more resistant to azoles than others. Our assay can be used to compare drug import in agricultural, medical and other pathogenic fungi.
Our experiments thus far have demonstrated that azole entry into the fungal cell is not solely by a passive diffusion mechanism. There may be some baseline level of azole passive diffusion into the cell but our evidence suggests azoles import into M. oryzae is more substantially through a plasma membrane-localized protein channel or carrier. Likewise, there may be a certain amount of azole import due to uncharacterized ATP-dependent importers that was masked by the high activity of ATP-dependent efflux transporters. Drug uptake was observed in de-energized cells (Figure 3A). However, media that contained glucose showed reduced final drug accumulation levels, presumably due to activation of glucose-dependent efflux pumps.
Our data strongly support the idea of azole entry into the cell by facilitated diffusion via a membrane protein channel or carrier that recognizes a specific moiety found in azole drugs. This finding is in agreement with studies on the human pathogenic fungi C. albicans, C. neoformans, and A. fumigatus as well as the model yeast S. cerevisiae (Mansfield et al., 2010; Esquivel et al., 2015).
Import of azoles did not require a proton gradient as no change was observed in uptake over a range of buffered pHs (Figure 3C). There was a trend toward alkaline sensitivity for drug uptake as seen by a decrease in 3H-FLC uptake in samples at pH 7 media. However, a deficiency in cell growth was observed in M. oryzae cells at pH 7, so import at this pH may be affected by other cellular factors directly or indirectly related to pH and proton gradients.
We did find significant differences in drug accumulation in M. oryzae depending on the growth media used, as shown in Figure 3B. Environmental adaptations that prevent or enhance azole uptake is an important aspect for drug resistance and treatment analysis. Based on these import results and a comparison of the components of these three medias, it is difficult to identify a single factor that would cause such a dramatic difference in uptake between CSM compared to YAD and YPD medias. The cell adaptations to the different media that affect FLC uptake is probably a complex mixture of differences in protein synthesis, lipid storage, transcriptional activity, and other metabolic activities that may alter the cell membrane composition. The altered azole uptake between the samples argues against passive diffusion entry into the cell, in which case one would expect only minimal reduction or increase in drug accumulation between the samples.
A comparison of drug import in exponential vs. post-exponentially growing cells (Figure 3D) shows that fluconazole accumulation is cell phase dependent. Drug accumulation in the older, post-exponential or stationary growing cells is dramatically reduced compared to the exponentially growing cells. This is consistent with major differences in cell metabolic activity when comparing exponentially growing cells and stationary phase cells, including changes to transcription, protein translation, modifications, and secretion, membrane maintenance, and other vital cell processes.
Exponentially growing cells are considered more active and responsive, while stationary phase cells shift to a period of maintenance and conservation (Werner-Washburne et al., 1993). It has been shown for some drugs, that proliferating cells are more sensitive than those cells in a steady state, so an increase in intracellular drug concentrations in exponential phase cells compared to stationary cells would correspond with those observations (43). This could be responsible for the decrease in azole uptake in exponential phase cell growth. The differential uptake of azoles into exponential cell phase is evidence against passive diffusion in which there would be no cell phase dependency.
Strong evidence for a saturable protein carrier is illustrated by competitive inhibition of 3H-FLC uptake by other azoles (Figure 4). 3H-FLC import was significantly inhibited by simultaneous treatment with an excess of unlabeled azoles. However, other antifungal drugs did not compete for import into the cell and may even stimulate uptake of azoles. This indicates substrate specificity for moieties found in the azole structure. The structure of competitive inhibitors and drugs that did not compete for import are shown in Tables 2, 3, respectively.
It is important to understand how different compounds are taken into the cell for the purposes of dosing and increasing treatment effectiveness, and also for combination therapy considerations. A combination of drugs that have the same mode of action but different mechanisms of uptake, may have an effect on synergy of the drugs. Antagonism may also be a result of competition for import into the cell.
Taking the 3H-FLC assay in another direction, we used radioactively labeled FLC to measure azole release from azole-preloaded hyphal cells. Our evidence indicates that the efflux of azoles is stimulated by energy (Figure 5) potentially via ABC efflux transporters, which suggests there are distinct transporters for influx and efflux of azoles, as opposed to a single transporter which functions in both directions.
The ABC superfamily of efflux pumps are well characterized for their ability to carry a broad range of substrates including, but not limited to, antifungal drugs across biological membranes (Decottignies et al., 1994; Holland and Blight, 1999; Wilkens, 2015). Resistance to commonly used antifungals has frequently been shown to develop due to overexpression or increased activity of ABC transporters in human fungal pathogens (Cannon et al., 2009; Sanglard, 2016). More recently, ABC transporters are being recognized for their role in pathogenicity, virulence, stress tolerance, and drug resistance in M. oryzae and other plant pathogens (Urban et al., 1999; Sun et al., 2006; Gupta and Chattoo, 2008; Price et al., 2015).
Much of the efflux of 3H-FLC from M. oryzae in glucose-stimulated and glucose-independent conditions occurred before the 4 h time point and so earlier time points would be useful for an in-depth analysis of efflux kinetics in M. oryzae (Figure 5).
The addition of clorgyline, a compound in the LOPAC collection and potential inhibitor of energy-dependent efflux, showed reduced efflux of 3H-FLC from energized M. oryzae cells (Figure 6). The ability of a compound to increase antifungal drug accumulation in the cell or prevent the rapid efflux of a drug makes it an ideal candidate to be considered for a synergistic combination therapy. A more thorough examination of clorgyline as an efflux inhibitor is needed, but our and others’ preliminary data suggest that clorgyline, or similar compounds, could potentially be used as combination therapy in either agriculture or clinical practice. The notion of considering medically now-outdated drugs for use as agricultural treatments unlocks many new possibilities. It might be more practical to reconsider ‘failed’ human treatment strategies, whether due to pharmacologic properties of absorption, distribution, adherence, etc. in humans, for use in plant fungal pathogens. Repurposing compounds from the LOPAC collection would shave years off the drug development process as these compounds have previously been well characterized, synthesized, stabilized, and near optimized.
Author Contributions
Design of the study: TW and BE. Experimental work: BE. Data analysis and interpretation: TW and BE. Writing the manuscript: BE. Final approval: TW and BE.
Funding
This research was supported by unrestricted research funds from the School of Biological Sciences, University of Missouri- Kansas City to TW. Research funds were also awarded to Brooke Esquivel by the UMKC Women’s Council Graduate Assistance Fund and the UMKC School of Graduate Studies Research Grant.
Conflict of Interest Statement
The authors declare that the research was conducted in the absence of any commercial or financial relationships that could be construed as a potential conflict of interest.
Acknowledgments
We would like to thank the White lab members past and present for their discussion, ideas and input. We would like to thank the Fungal Genetic Stock Center, currently at Kansas State University for providing the M. oryzae strains and in particular for the help of Kevin McCluskey and Aric Wiest for their advice on methods of strain growth and maintenance.
References
Ashkani, S., Rafii, M. Y., Sariah, M., Siti Nor Akmar, A., Rusli, I., Abdul Rahim, H., et al. (2011). Analysis of simple sequence repeat markers linked with blast disease resistance genes in a segregating population of rice (Oryza sativa). Genet. Mol. Res. 10, 1345–1355. doi: 10.4238/vol10-3gmr1331
Cannon, R. D., Lamping, E., Holmes, A. R., Niimi, K., Baret, P. V., Keniya, M. V., et al. (2009). Efflux-mediated antifungal drug resistance. Clin. Microbiol. Rev. 22, 291–321. doi: 10.1128/CMR.00051-08
Coleman, J. J., and Mylonakis, E. (2009). Efflux in fungi: la piece de resistance. PLoS Pathog. 5:e1000486. doi: 10.1371/journal.ppat.1000486
Cools, H. J., Parker, J. E., Kelly, D. E., Lucas, J. A., Fraaije, B. A., and Kelly, S. L. (2010). Heterologous expression of mutated eburicol 14alpha-demethylase (CYP51) proteins of Mycosphaerella graminicola to assess effects on azole fungicide sensitivity and intrinsic protein function. Appl. Environ. Microbiol. 76, 2866–2872. doi: 10.1128/AEM.02158-09
Couch, B. C., Fudal, I., Lebrun, M. H., Tharreau, D., Valent, B., van Kim, P., et al. (2005). Origins of host-specific populations of the blast pathogen Magnaporthe oryzae in crop domestication with subsequent expansion of pandemic clones on rice and weeds of rice. Genetics 170, 613–630. doi: 10.1534/genetics.105.041780
Dean, R. A., Talbot, N. J., Ebbole, D. J., Farman, M. L., Mitchell, T. K., Orbach, M. J., et al. (2005). The genome sequence of the rice blast fungus Magnaporthe grisea. Nature 434, 980–986. doi: 10.1038/nature03449
Decottignies, A., Kolaczkowski, M., Balzi, E., and Goffeau, A. (1994). Solubilization and characterization of the overexpressed PDR5 multidrug resistance nucleotide triphosphatase of yeast. J. Biol. Chem. 269, 12797–12803.
Edwards, S. G., and Godley, N. P. (2010). Reduction of Fusarium head blight and deoxynivalenol in wheat with early fungicide applications of prothioconazole. Food Addit. Contam. Part A Chem. Anal. Control Expo. Risk Assess. 27, 629–635. doi: 10.1080/19440040903515942
Esquivel, B. D., Smith, A. R., Zavrel, M., and White, T. C. (2015). Azole drug import into the pathogenic fungus Aspergillus fumigatus. Antimicrob. Agents Chemother. 59, 3390–3398. doi: 10.1128/AAC.0500314
Fleming, R. V., Walsh, T. J., and Anaissie, E. J. (2002). Emerging and less common fungal pathogens. Infect. Dis. Clin. North Am. 16, 915–933.
Fowler, C. J., Oreland, L., and Callingham, B. A. (1981). The acetylenic monoamine oxidase inhibitors clorgyline, deprenyl, pargyline and J-508: their properties and applications. J. Pharm. Pharmacol. 33, 341–347.
Gupta, A., and Chattoo, B. B. (2008). Functional analysis of a novel ABC transporter ABC4 from Magnaporthe grisea. FEMS Microbiol. Lett. 278, 22–28. doi: 10.1111/j.1574-6968.2007.00937.x
Harrison, P. (2002). World Agriculture: Towards 2015/2030 Summary Report. Rome: Food and Agriculture Organization of the United Nations.
Hawkins, N. J., Cools, H. J., Sierotzki, H., Shaw, M. W., Knogge, W., Kelly, S. L., et al. (2014). Paralog re-emergence: a novel, historically contingent mechanism in the evolution of antimicrobial resistance. Mol. Biol. Evol. 31, 1793–1802. doi: 10.1093/molbev/msu134
Holland, I. B., and Blight, M. A. (1999). ABC-ATPases, adaptable energy generators fuelling transmembrane movement of a variety of molecules in organisms from bacteria to humans. J. Mol. Biol. 293, 381–399. doi: 10.1006/jmbi.1999.2993
Holmes, A. R., Keniya, M. V., Ivnitski-Steele, I., Monk, B. C., Lamping, E., Sklar, L. A., et al. (2012). The monoamine oxidase A inhibitor clorgyline is a broad-spectrum inhibitor of fungal ABC and MFS transporter efflux pump activities which reverses the azole resistance of Candida albicans and Candida glabrata clinical isolates. Antimicrob. Agents Chemother. 56, 1508–1515. doi: 10.1128/AAC.0570611
Kaur, S., Takkar, R., Bhardwaj, U., Kumar, R., Battu, R. S., and Singh, B. (2012). Dissipation kinetics of trifloxystrobin and tebuconazole on wheat leaves and their harvest time residues in wheat grains and soil. Bull. Environ. Contam. Toxicol. 89, 606–610. doi: 10.1007/s00128-012-0748-y
Kim, Y., Park, S. Y., Kim, D., Choi, J., Lee, Y. H., Lee, J. H., et al. (2013). Genome-scale analysis of ABC transporter genes and characterization of the ABCC type transporter genes in Magnaporthe oryzae. Genomics 101, 354–361. doi: 10.1016/j.ygeno.2013.04.003
Kovalchuk, A., and Driessen, A. J. (2010). Phylogenetic analysis of fungal ABC transporters. BMC Genomics 11:177. doi: 10.1186/1471-2164-11177
Lehoczki-Krsjak, S., Varga, M., and Mesterhazy, A. (2015). Distribution of prothioconazole and tebuconazole between wheat ears and flag leaves following fungicide spraying with different nozzle types at flowering. Pest. Manag. Sci. 71, 105–113. doi: 10.1002/ps.3774
Lehoczki-Krsjak, S., Varga, M., Szabo-Hever, A., and Mesterhazy, A. (2013). Translocation and degradation of tebuconazole and prothioconazole in wheat following fungicide treatment at flowering. Pest. Manag. Sci. 69, 1216–1224. doi: 10.1002/ps.3486
Lepesheva, G. I., and Waterman, M. R. (2007). Sterol 14alpha-demethylase cytochrome P450 (CYP51), a P450 in all biological kingdoms. Biochim. Biophys. Acta 1770, 467–477. doi: 10.1016/j.bbagen.2006.07.018
Mansfield, B. E., Oltean, H. N., Oliver, B. G., Hoot, S. J., Leyde, S. E., Hedstrom, L., et al. (2010). Azole drugs are imported by facilitated diffusion in Candida albicans and other pathogenic fungi. PLoS Pathog. 6:e1001126. doi: 10.1371/journal.ppat.1001126
Martin-Urdiroz, M., Oses-Ruiz, M., Ryder, L. S., and Talbot, N. J. (2016). Investigating the biology of plant infection by the rice blast fungus Magnaporthe oryzae. Fungal Genet. Biol. 90, 61–68. doi: 10.1016/j.fgb.2015.12.009
Mellado, E., Garcia-Effron, G., Buitrago, M. J., Alcazar-Fuoli, L., Cuenca-Estrella, M., and Rodriguez-Tudela, J. L. (2005). Targeted gene disruption of the 14-alpha sterol demethylase (cyp51A) in Aspergillus fumigatus and its role in azole drug susceptibility. Antimicrob. Agents Chemother. 49, 2536–2538. doi: 10.1128/AAC.49.6.2536-2538.2005
Parker, J. E., Warrilow, A. G., Cools, H. J., Fraaije, B. A., Lucas, J. A., Rigdova, K., et al. (2013). Prothioconazole and prothioconazole-desthio activities against Candida albicans sterol 14-alpha-demethylase. Appl. Environ. Microbiol. 79, 1639–1645. doi: 10.1128/AEM.03246-12
Parker, J. E., Warrilow, A. G., Cools, H. J., Martel, C. M., Nes, W. D., Fraaije, B. A., et al. (2011). Mechanism of binding of prothioconazole to Mycosphaerella graminicola CYP51 differs from that of other azole antifungals. Appl. Environ. Microbiol. 77, 1460–1465. doi: 10.1128/AEM.01332-10
Price, C. L., Parker, J. E., Warrilow, A. G., Kelly, D. E., and Kelly, S. L. (2015). Azole fungicides - understanding resistance mechanisms in agricultural fungal pathogens. Pest. Manag. Sci. 71, 1054–1058. doi: 10.1002/ps.4029
Sanglard, D. (2016). Emerging threats in antifungal-resistant fungal pathogens. Front. Med. (Lausanne) 3:11. doi: 10.3389/fmed.2016.00011
Sun, C. B., Suresh, A., Deng, Y. Z., and Naqvi, N. I. (2006). A multidrug resistance transporter in Magnaporthe is required for host penetration and for survival during oxidative stress. Plant Cell 18, 3686–3705. doi: 10.1105/tpc.105.037861
Talbot, N. J. (2003). On the trail of a cereal killer: exploring the biology of Magnaporthe grisea. Annu. Rev. Microbiol. 57, 177–202. doi: 10.1146/annurev.micro.57.030502.090957
Thornton, C. R., and Wills, O. E. (2015). Immunodetection of fungal and oomycete pathogens: established and emerging threats to human health, animal welfare and global food security. Crit. Rev. Microbiol. 41, 27–51. doi: 10.3109/1040841X.2013.788995
Tipton, K. F. (1997). Monoamine oxidase inhibitors and pressor response to dietary amines. Vopr. Med. Khim. 43, 494–503.
Urban, M., Bhargava, T., and Hamer, J. E. (1999). An ATP-driven efflux pump is a novel pathogenicity factor in rice blast disease. EMBO J. 18, 512–521. doi: 10.1093/emboj/18.3.512
Villa, F., Cappitelli, F., Cortesi, P., and Kunova, A. (2017). Fungal biofilms: targets for the development of novel strategies in plant disease management. Front. Microbiol. 8:654. doi: 10.3389/fmicb.2017.00654
Warrilow, A. G., Melo, N., Martel, C. M., Parker, J. E., Nes, W. D., Kelly, S. L., et al. (2010). Expression, purification, and characterization of Aspergillus fumigatus sterol 14-alpha demethylase (CYP51) isoenzymes A and B. Antimicrob. Agents Chemother. 54, 4225–4234. doi: 10.1128/AAC.00316-10
Werner-Washburne, M., Braun, E., Johnston, G. C., and Singer, R. A. (1993). Stationary phase in the yeast Saccharomyces cerevisiae. Microbiol. Rev. 57, 383–401.
Wilkens, S. (2015). Structure and mechanism of ABC transporters. F1000Prime Rep. 7:14. doi: 10.12703/P7-14
Wilson, R. A., and Talbot, N. J. (2009). Under pressure: investigating the biology of plant infection by Magnaporthe oryzae. Nat. Rev. Microbiol. 7, 185–195. doi: 10.1038/nrmicro2032
Yan, X., Ma, W. B., Li, Y., Wang, H., Que, Y. W., Ma, Z. H., et al. (2011). A sterol 14alpha-demethylase is required for conidiation, virulence and for mediating sensitivity to sterol demethylation inhibitors by the rice blast fungus Magnaporthe oryzae. Fungal Genet. Biol. 48, 144–153. doi: 10.1016/j.fgb.2010.09.005
Yan, X., and Talbot, N. J. (2016). Investigating the cell biology of plant infection by the rice blast fungus Magnaporthe oryzae. Curr. Opin. Microbiol. 34, 147–153. doi: 10.1016/j.mib.2016.10.001
Keywords: Magnaporthe, drug import, azole antifungals, filamentous fungi, clorgyline, facilitated diffusion
Citation: Esquivel BD and White TC (2017) Accumulation of Azole Drugs in the Fungal Plant Pathogen Magnaporthe oryzae Is the Result of Facilitated Diffusion Influx. Front. Microbiol. 8:1320. doi: 10.3389/fmicb.2017.01320
Received: 04 May 2017; Accepted: 29 June 2017;
Published: 13 July 2017.
Edited by:
Elaine M. Bignell, University of Manchester, United KingdomReviewed by:
Dominique Sanglard, University of Lausanne, SwitzerlandMalcolm Whiteway, Concordia University, Canada
Copyright © 2017 Esquivel and White. This is an open-access article distributed under the terms of the Creative Commons Attribution License (CC BY). The use, distribution or reproduction in other forums is permitted, provided the original author(s) or licensor are credited and that the original publication in this journal is cited, in accordance with accepted academic practice. No use, distribution or reproduction is permitted which does not comply with these terms.
*Correspondence: Theodore C. White, whitetc@umkc.edu Brooke D. Esquivel, bdp3k9@mail.umkc.edu