The Effect of Apolipoprotein E ε4 (APOE ε4) on Visuospatial Working Memory in Healthy Elderly and Amnestic Mild Cognitive Impairment Patients: An Event-Related Potentials Study
- 1Department of Neurology, Affiliated ZhongDa Hospital, School of Medicine, Southeast University, Nanjing, China
- 2Department of Psychology, Xinxiang Medical University, Xinxiang, China
- 3Department of Biophysics, Medical College of Wisconsin, Milwaukee, WI, United States
Background: Apolipoprotein E (APOE) ε4 is the only established risk gene for late-onset, sporadic Alzheimer’s disease (AD). Previous studies have provided inconsistent evidence for the effect of APOE ε4 status on the visuospatial working memory (VSWM).
Objective: The aim was to investigate the effect of APOE ε4 on VSWM with an event-related potential (ERP) study in healthy controls (HC) and amnestic mild cognitive impairment (aMCI) patients.
Methods: The study recorded 39 aMCI patients (27 APOE ε4 non-carriers and 12 APOE ε4 carriers) and their 43 matched controls (25 APOE ε4 non-carriers and 18 APOE ε4 carriers) with an 64-channel electroencephalogram. Participants performed an N-back task, a VSWM paradigm that manipulated the number of items to be stored in memory.
Results: The present study detected reduced accuracy and delayed mean correct response time (RT) in aMCI patients compared to HC. P300, a positive component that peaks between 300 and 500 ms, was elicited by the VSWM task. In addition, aMCI patients showed decreased P300 amplitude at the central–parietal (CP1, CPz, and CP2) and parietal (P1, Pz, and P2) electrodes in 0- and 1-back task compared to HC. In both HC and aMCI patients, APOE ε4 carriers showed reduced P300 amplitude with respect to non-carriers, whereas no significant differences in accuracy or RT were detected between APOE ε4 carriers and non-carriers. Additionally, standardized low-resolution brain electromagnetic tomography analysis (s-LORETA) showed enhanced brain activation in the right parahippocampal gyrus (PHG) during P300 time range in APOE ε4 carriers with respect to non-carriers in aMCI patients.
Conclusion: It demonstrated that P300 amplitude could predict VSWM deficits in aMCI patients and contribute to early detection of VSWM deficits in APOE ε4 carriers.
Introduction
Alzheimer’s disease (AD) is the most common cause of dementia, with progressive cognitive impairment as the primary symptom. Mild cognitive impairment (MCI) represents a transitional stage between normal aging and dementia (Petersen et al., 1999; Laczó et al., 2009). Amnestic mild cognitive impairment (aMCI) refers to the amnestic form of MCI. It has been demonstrated that MCI patients convert to probable AD at a rate of approximately 10–15% per year (Grundman et al., 2004), while normal controls progress to dementia at a rate of 1–2% per year (Bischkopf et al., 2002). Thus, early detection of MCI patients might be crucial for slowing down its progression to AD. Humans possess three major apolipoprotein E (APOE) isoforms encoded by ε2, ε3, and ε4 alleles. APOE ε4 is the only established risk gene for late-onset, sporadic AD (Seripa et al., 2009). Previous investigations demonstrated the critical role of APOE ε4 in the onset and progression of AD. APOE ε4 carriers show more faster cognitive decline (Bretsky et al., 2003), atrophic hippocampal volume in magnetic resonance imaging (MRI) markers (Petersen et al., 2000; Shi et al., 2009), increased cerebral amyloid deposition (Klunk et al., 2004) and cerebral hypometabolism compared to non-carriers (Reiman et al., 2005).
Working memory (WM) is a cognitive system for temporary holding, processing and manipulation of information. Regarding WM, previous studies confirmed that it is related to information processing speed and executive function (Brown et al., 2012). Episodic and semantic memory were deemed as earliest cognitive impairments in aMCI patients in previous investigations (Hodges et al., 2006; Leyhe and Muller, 2009). However, recent studies reported that WM impairment might also be a sensitive biomarker for the early detection of aMCI (Saunders and Summers, 2011; Zheng et al., 2012). Previous studies demonstrated that visuospatial working memory (VSWM) deficits start near the beginning of the MCI phase (Twamley et al., 2006; Alescio-Lautier et al., 2007; Webster et al., 2014). In APP mouse models of AD, spatial working memory (SWM) assessed by Morris water maze test was the earliest observable cognitive domain to demonstrate deficits, followed by recognition memory (Alescio-Lautier et al., 2007; Webster et al., 2013, 2014). In recent years, the applications of functional MRI (fMRI) studies have made prominent advances in the investigations of MCI. Several fMRI investigations identified the presence of VSWM related functional brain activation changes in aMCI patients (Kochan et al., 2010; Alichniewicz et al., 2012, Mitolo et al., 2013; Lou et al., 2015). However, regarding the effect of APOE ε4 status on the VSWM, previous fMRI studies provide inconsistent evidence. A study reported that APOE ε4 carriers in cognitively intact adults show lesser activation in medial temporal lobes (MTL) compared to non-carriers during VSWM (Borghesani et al., 2008), whereas Alichniewicz et al. (2012) indicated that APOE ε4 status has no impact on brain activation during VSWM in healthy controls (HC) or aMCI patients. Another neuropsychological assessment revealed no influence of APOE polymorphisms on WM in cognitively intact adults (Ward et al., 2014). Thus, the present study aimed to investigate the effect of APOE ε4 status on VSWM with event-related potential (ERP).
ERP has an excellent temporal resolution and reflects instantly the summated excitatory postsynaptic potential (EPSP) and inhibitory postsynaptic potential (IPSP), primarily of pyramidal cells in the neocortex (Nunez and Srinivasan, 2006). The high temporal resolution of ERP enabled the understanding of the neural correlates of cognitive processing and functioning. The present investigation used the N-back paradigm to evaluate the effect of APOE ε4 status on VSWM. The load factor N can be adjusted to make the task more or less difficult (e.g., 0-back and 1-back). In previous ERP studies, P300, a positive component that peaks between 300 and 500 ms, was elicited by WM tasks (Watter et al., 2001; Nakao et al., 2012). According to neuropsychological opinion, classical P300 (P3b) was produced by updating operation of WM (Papageorgiou et al., 2002; Polich, 2007). A study with an auditory target detection task indicated decreased P300 amplitude and prolonged P300 latency in APOE ε4 carriers compared to non-carriers in cognitively intact adults (Irimajiri et al., 2010). The current ERP study was designed as a novel investigation on the impact of APOE ε4 status on VSWM in HC and aMCI patients.
The aim of the present study was to investigate the influence of APOE ε4 status on ERP changes during VSWM activation in HC and aMCI patients. On the basis of the above-mentioned theory, we hypothesized that: First, aMCI patients showed reduced P300 amplitude and delayed P300 latency compared to HC; Second, P300 amplitude and latency was correlated with information processing speed and executive function; Third, the P300 component with lower amplitude and delayed latency was observed in APOE ε4 carriers with respect to non-carriers in non-demented participants and aMCI patients; Forth, APOE ε4 carriers showed brain activation alteration compared to non-carriers.
Materials and Methods
Participants
In this study, a cohort of 39 aMCI patients and 43 HC were enrolled through community health screening, newspaper advertisements and a memory outpatient clinic (all participants were Chinese Han). The responsible Human Participants Ethics Committee of the Affiliated ZhongDa Hospital, Southeast University (2016ZDSYLL011.0) approved our study. All the participants provided written informed consents. Moreover, all the participants ranged from 55 to 80 years old and were right-handed.
Neuropsychological Assessments
As described previously (Shu et al., 2016; Su et al., 2017), all participants underwent neuropsychological assessments, including Mini-Mental State Examination (MMSE), Mattis Dementia Rating Scale-2 (MDRS-2), Clinical Dementia Rating (CDR), Auditory Verbal Learning Test-Delayed Recall (AVLT-DR), the Rey–Osterrieth Complex Figure Test-Delayed Recall (ROCFT-DR), Trail Making Tests (TMT), and Stroop Test (ST), Digit Span Test, Symbol Digit Modalities Test, and the Clock Drawing Test to assess cognitive functions (general cognitive functions, episodic memory, executive function, information processing speed, and visual spatial domains).
Inclusion Criteria and Exclusion Criteria
All aMCI patients met the diagnostic criteria (Petersen, 2004; Albert et al., 2011; Bai et al., 2011), which include: Subjective memory impairment confirmed by the participant and his informant; Objective memory performance documented by an AVLT-delayed recall score less than or equal to 1.5 standard deviation (SD) of age- and education-adjusted norms (cut-off of ≤ 4 correct responses on 12 items for patients with ≥8 years of education); MMSE score equal or greater than 24; CDR of 0.5; No or minimal impairment in activities of daily living; Absence of dementia or insufficient dementia to meet the NINCDS-ADRDA (National Institute of Neurological and Communicative Disorders and Stroke and the AD and Related Disorders Association) Alzheimer’s Criteria. Additionally, the controls had a CDR of 0, an MMSE score equal or greater than 26, and an AVLT-delayed recall score greater than 4 for participants with 8 or more years of education. Participants who had a history of neurological or psychiatric disorders, major medical illness, or severe visual or hearing loss were excluded from the study.
APOE Genotyping
The process of APOE genotyping was on the basis of previous protocols (Shu et al., 2014, 2016). Each participant’s genomic deoxyribonucleic acid (DNA) was extracted, with a DNA direct kit, from 250-μL ethylenediaminetetraacetic acid (EDTA)-anticoagulated blood (Tiangen, China). A polymerase chain reaction-based restriction fragment length polymorphism (PCR-RFLP) assay detected alleles of rs7412 and rs429358, respectively. Eventually, the APOE genotype was determined by the haplotype of rs7412 and rs429358. Thus, the participants were further divided into four groups according to their APOE genotypes, as displayed in Table 1. Each group was identified as HC-APOE ε4-, HC-APOE ε4+, aMCI-APOE ε4-, and aMCI-APOE ε4+.
Procedure and Stimuli
Participants sat in front of a 17 inch computer screen (refresh rate 75 Hz) placed at a distance of 70 cm from their eyes in a quiet, dimly lit, sound-proofed room, with an ambient temperature of about 24°C (Chen et al., 2013; Jiu et al., 2013). Stimuli and task were controlled with E-Prime 2.0 software (Psychology Software Tools Inc., Pittsburgh, PA, United States). The investigation used a visual N-back task (0- and 1-back task, see Figure 1) to assess WM. Each block started with the statements of instructions. Participants were asked to minimize head, body movements and to keep their eyes fixated on a cross presented in the middle of the screen throughout the stimulus presentation. A continuous stream of white block (size: 2.6 × 2.6 degree at 70 cm from the face) was presented, one white block per frame, for 300 ms each. Inter stimulus interval (ISI) were 2000 ms in both studied conditions. Participants were asked to respond using their dominant hand. Each white block was randomly positioned in one of eight possible locations (both ends of horizontal X-axis, vertical Y-axis and the lower and upper position of both diagonals). In 0-back load, participants were asked to recognize whether a white block appeared on the upper left side of the screen. In 1-back load, participants had to identify whether the current stimulus displayed in the same locations as the previous presentation. Participants had to distinguish between targets and non-targets by pressing the button with their right index or middle finger. Response time (RT) and accuracy were recorded after pressing the button. Trials were presented in eight blocks (4 blocks for 0- and 1-back task, respectively); each block representing either the control (0-back) or WM conditions (1-back). Each block was composed of 20 trials with a 3:7 target/no target stimulus (Gevins and Cutillo, 1993). All the participants were instructed to respond as fast and as accurately as possible.
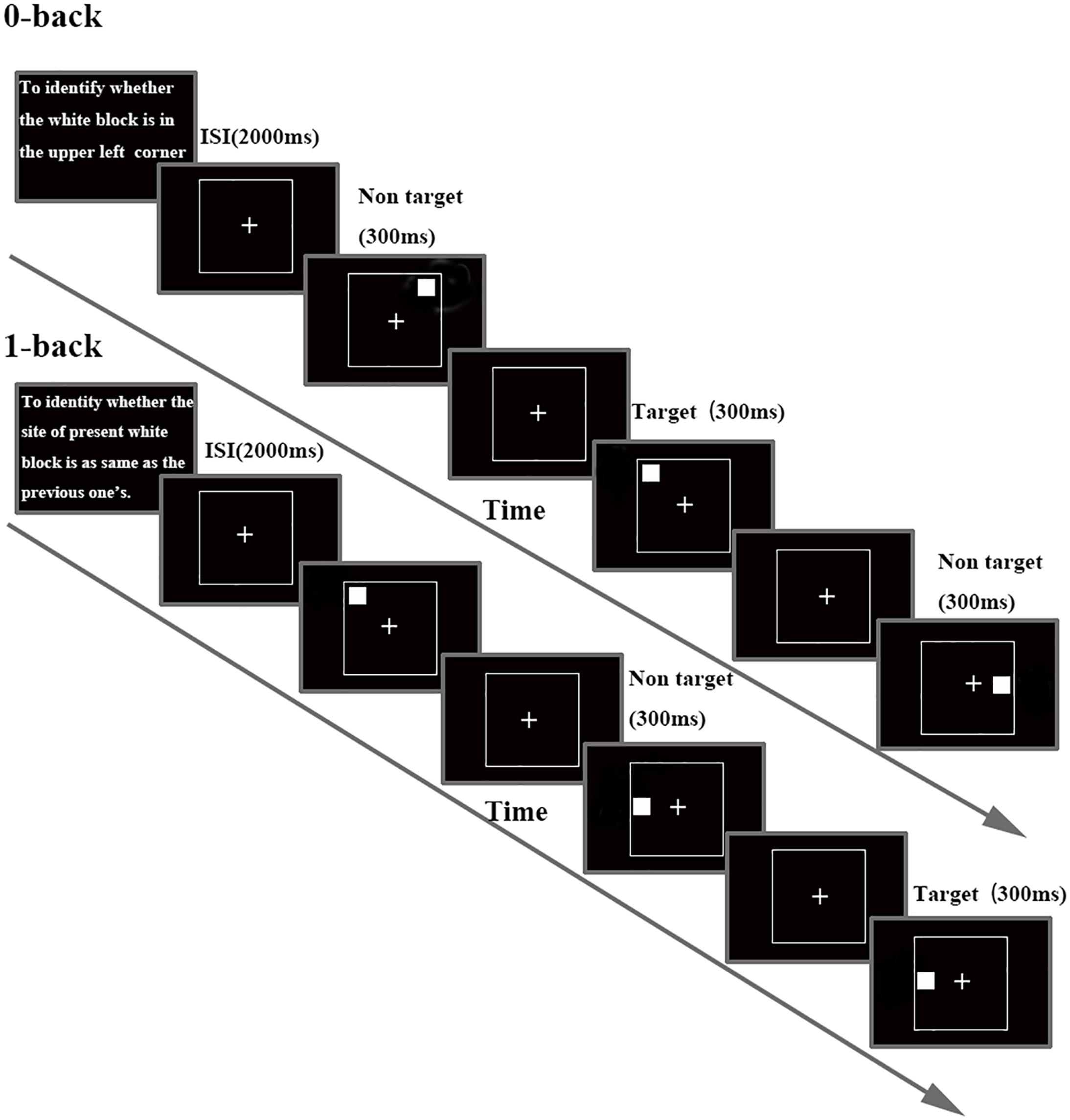
FIGURE 1. Schematic diagram of the N-back task, separately for each task load. Details about timing for stimulus and inter stimulus interval (ISI) are included.
EEG Acquisition and Analysis
Electroencephalogram (EEG) measurements were recorded with a BrainAmp MR portable ERP system (Brain Products GmbH; Munich, Germany) from 64 scalp electrodes (impedances < 5 kΩ; vertex reference; 500 Hz digitization). Electrodes were arranged according to the international extended 10–20 system. FCz electrode acted as an internal reference during online recording. All electrodes were re-referenced to an average reference for data off-line analysis. The electrooculogram (EOG) was positioned above and below the right eye (vertical EOG) and at the outer canthi of both eyes (horizontal EOG). Ocular artifact correction was performed with independent component analysis (Jung et al., 2000; Neuhaus et al., 2011). The EEG was band-pass filtered from 0.1 to 100 Hz (and a gain of 20,000). Data were analyzed with BrainVision Analyzer software 2.0 (Brain Products GmbH; Munich, Germany). In offline analyses, the EEG signal was band-pass filtered 0.01–30 Hz (Chen et al., 2014a,b). EEG signals with amplitude larger than ±100 μV were rejected (Chen et al., 2014c). To calculate the ERP, epochs of EEG were averaged offline from 100 ms pre stimulus to 700 ms post stimulus relative to a 100-ms pre stimulus baseline. The latency ranges were 300–500 ms for the P300. The peak amplitude and latency of the P300 component were acquired with peak detection process. On the basis of these studies (Sokhadze et al., 2008; Shishkin et al., 2011; Yu and Zhang, 2014), P300 variables were obtained at central–parietal and parietal electrodes.
Statistical Analysis
The statistical analyses were implemented with SPSS 21.0 software. Statistical threshold was set at a p < 0.05.
Demographic and Neuropsychological Data
One-way analysis of variance (ANOVA) and Mann-Fisher’s exact probability tests were performed to compare the demographic data and neuropsychological performances between APOE ε4 carriers and non-carriers in aMCI patients and HC. Bonferroni’s post hoc test was used to detect the source of ANOVA difference.
Behavioral Analysis
Accuracy and RT were analyzed with a repeated measures analysis of covariance (RMANCOVA) with appropriate Greenhouse–Geisser corrections. Each RMANCOVA was conducted using memory loads (0-back and 1-back), stimulus types (target stimulus and non-target stimulus) as within-subject factors, and groups (aMCI patients and HC), APOE genotypes (APOE ε4 carriers and APOE ε4 non-carriers) as between-subject factors. Age, gender, years of education were included as covariates. Post hoc comparisons were adjusted using the Bonferroni correction.
ERP Analysis
Mean peak amplitude and latency were subjected to RMANCOVA with memory loads and electrode locations (CP1, CPz, CP2, P1, Pz, and P2) as within-subject factors, groups, APOE genotypes as between-subjects factors. Age, gender, years of education were included as covariates. Post hoc comparisons were adjusted using the Bonferroni correction.
Next, the mean P300 amplitudes and latencies for each task load were obtained by averaging across electrodes. Pearson Correlation was conducted between P300 amplitude/latency and neuropsychological performance (general cognitive functions, episodic memory, executive function, information processing speed, and visual spatial domains) partialing out the effect of age, gender, education, and APOE genotypes in HC and aMCI patients, respectively.
Source Localization Analysis
The standardized low-resolution brain electromagnetic tomography analysis (sLORETA) is an efficient functional imaging method for source localization and widely used in ERP studies (Pandey et al., 2012). It was implemented widely in WM studies (Suchan et al., 2005; Keeser et al., 2011). The sLORETA version in our investigation received considerable validation in previous studies (Pascual-Marqui, 2002; Pandey et al., 2012; Li et al., 2016), available at: http://www.uzh.ch/keyinst/loreta.htm. The cortex was modeled as a collection of volume elements (voxels) in the digitized Montreal Neurological Institute (MNI) coordinates corrected to the Talairach coordinates (Pascual-Marqui, 2002). The electrode coordinates were created from the 61 electrode locations. A transformation matrix was created with the electrode coordinates. In the study, the averaged waveforms (for all 400 time samples, from 100 ms pre stimulus to 700 ms post stimulus) for each participant were converted and saved into ASCII values. The contrast for the 1-back condition relative to the 0-back condition was selected for statistical study in order to derive statistical power by comparing the active task with the control task. To test for hypothesized differences in brain activation as a function of genotype, the contrast for the 1-back relative to 0-back was entered into an independent t-test analysis in sLORETA, with the APOE genotypes as the between-subject factors. Source localization was composed of two key parts: ERP analysis and LORETA analysis. During ERP analysis, we detected 1-back relative to 0-back difference between APOE ε4 carriers and non-carriers with t-statistic analysis. After view of the combined ERP (which is not an actual ERP), latency where maximum t-value occurred were attained for LORETA analysis. The sLORETA values for each participant were computed with ASCII values, electrode coordinates and transformation matrix, separately. Regarding the sLORETA analysis, 1-back relative to 0-back differences between two groups were compared in the time range of P300 component which showed difference in amplitude or latency. Finally, images were generated to localize these differences in the three dimensional (3-D) space within the brain.
Results
Demographic and Neuropsychological Results
Demographic and neuropsychological characteristics of participants are shown in Table 1. One way ANOVA indicated no significant differences between four groups in age, gender, and years of education. It detected significant differences between four groups in MMSE scores [F(3,78) = 4.503, p = 0.006], episodic memory [F(3,78) = 22.537, p < 0.001], information processing speed [F(3,78) = 2.941, p = 0.038] and executive function [F(3,78) = 4.057, p = 0.010], whereas no significant differences were indicated in Mattis Dementia Rating Scale-2 (MDRS-2) scores and visuospatial function between four groups. Post hoc analysis indicated significantly decreased MMSE scores, episodic memory and executive function in aMCI-APOE ε4- participants compared to HC-APOE ε4- participants. In addition, HC-APOE ε4+ participants showed better episodic memory compared to aMCI-APOE ε4+ participants.
Behavioral Results
Table 2 showed accuracy and correct RTs in each memory load condition (0- and 1-back) for target and non-target stimuli for aMCI patients and HC with different APOE genotypes.
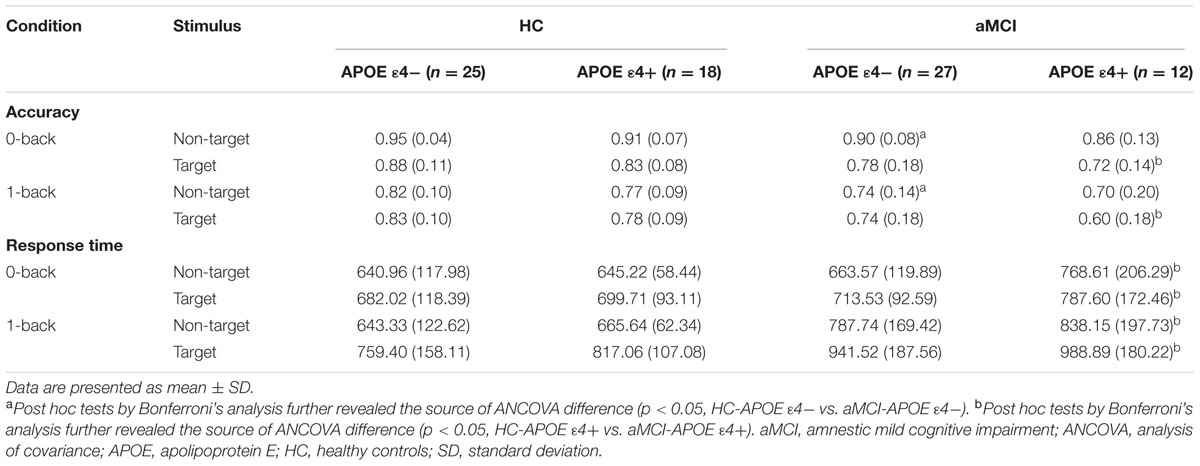
TABLE 2. Behavioral data [accuracy and response time (RT)] for healthy controls and aMCI patients with different APOE ε4 status.
RMANCOVA showed a significant main effect of groups on accuracy [F(1,78) = 10.443, p = 0.002], whereas it indicated no significant main effect of APOE genotypes on accuracy. Post hoc analysis detected that MCI-APOE ε4- participants showed decreased accuracy with non-target stimuli in both two conditions (0-back and 1-back) with respect to HC-APOE ε4- participants (all p < 0.05, see Table 2). Additionally, post hoc analysis showed reduced accuracy for aMCI-APOE ε4+ participants compared to HC-APOE ε4+ participants with target stimulus in both two conditions (0-back and 1-back) (all p < 0.05, see Table 2).
RMANCOVA showed a significant main effect of groups on RT [F(1,78) = 9.506, p = 0.003], whereas it revealed no significant main effect of APOE genotypes on RT. Post hoc analysis detected that aMCI-APOE ε4+ participants showed prolonged RT compared to HC-APOE ε4+ participants with non-target and target stimuli in both two conditions (0-back and 1-back) (all p < 0.05, see Table 2).
ERP Results
P300 amplitude and latency were assessed at central–parietal (CP1, CPz, and CP2) and parietal (P1, Pz, and P2) electrodes, where the component was maximal (see Figure 2). RMANCOVA indicated main effects of groups and APOE genotypes on P300 amplitude [groups: F(1,78) = 9.160, p = 0.004; APOE genotypes: F(1,78) = 5.077, p = 0.028]. Post hoc analysis detected decreased P300 amplitude in HC-APOE ε4+ participants compared to HC-APOE ε4- participants at P2 electrode in 0-back task and CPz electrode in 1-back task (all p < 0.05, see Table 3). In addition, aMCI-APOE ε4+ participants showed decreased P300 amplitude compared to aMCI-APOE ε4- participants at P1 electrode in 0-back task and CP2 electrode in 1-back task (all p < 0.05, see Table 3). Moreover, post hoc analysis indicated that P300 amplitude was diminished for aMCI-APOE ε4- participants compared to HC-APOE ε4- participants at CPz, P2 electrodes in 0-back task and CPz, P1, P2 electrodes in 1-back task. Additionally, aMCI-APOE ε4+ participants showed reduced P300 amplitude with respect to HC-APOE ε4+ participants at P1 electrode in 0-back task and CP2, P2 electrodes in 1-back task. RMANCOVA indicated no significant main effects of groups or APOE genotypes on P300 latency.
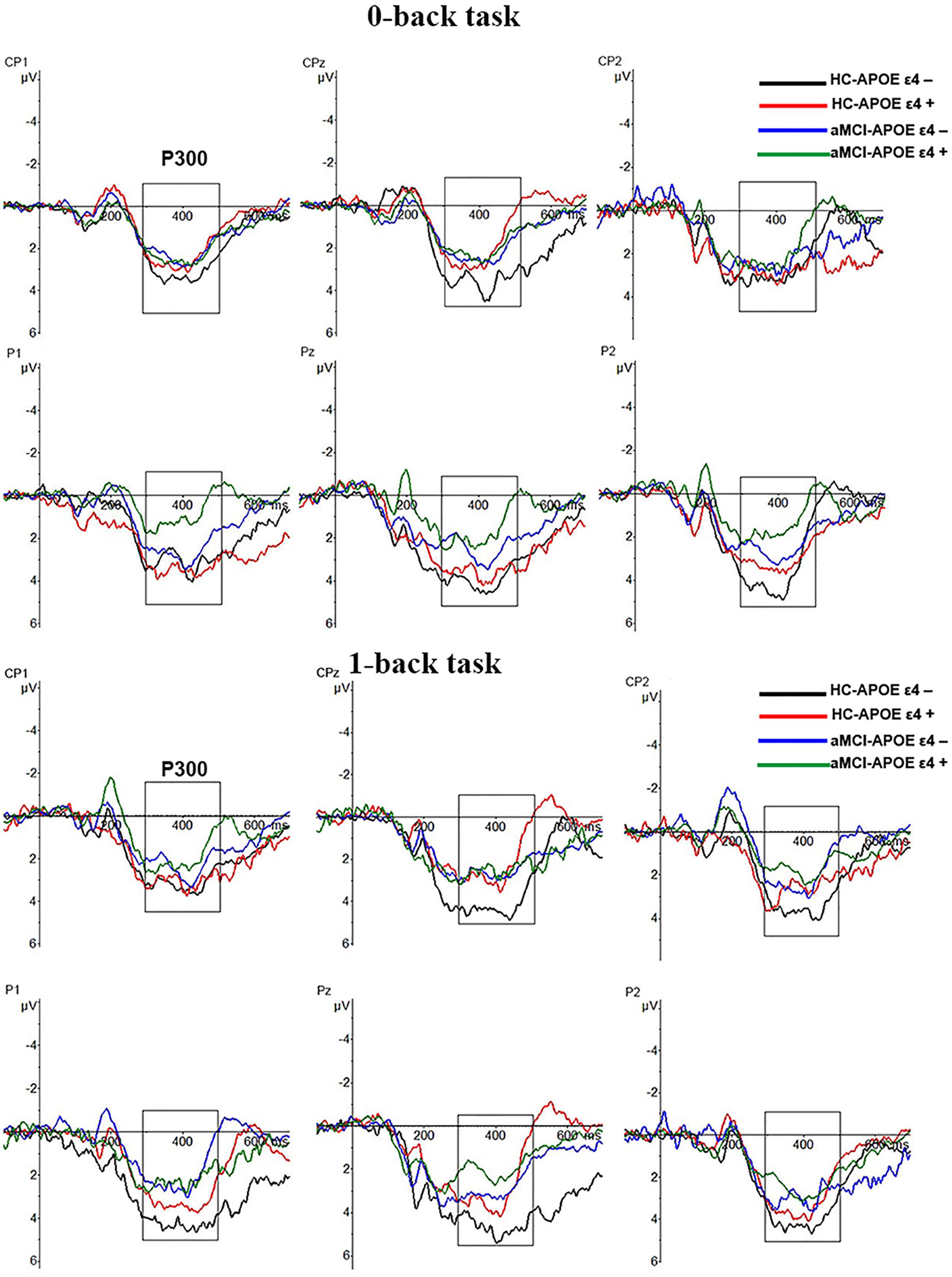
FIGURE 2. Grand-average event-related potential (ERP) waveforms of the 0-back and 1-back task for HC-APOE ε4–, HC-APOE ε4+, aMCI-APOE ε4–, and aMCI-APOE ε4+ participants. Grand-average ERP at the central–parietal (CP1, CPz, and CP2) and parietal (P1, Pz, and P2) electrodes for HC-APOE ε4– participants in 0- and 1-back task (black line), HC-APOE ε4+ participants in 0- and 1-back task (red line) and aMCI-APOE ε4– participants in 0- and 1-back task (blue line), aMCI-APOE ε4+ participants in 0- and 1-back task (green line). Dashed squares on each graph represent the time windows used for analyses of the P300 component. Abbreviations: aMCI, amnestic mild cognitive impairment; APOE, apolipoprotein E; HC, healthy controls.
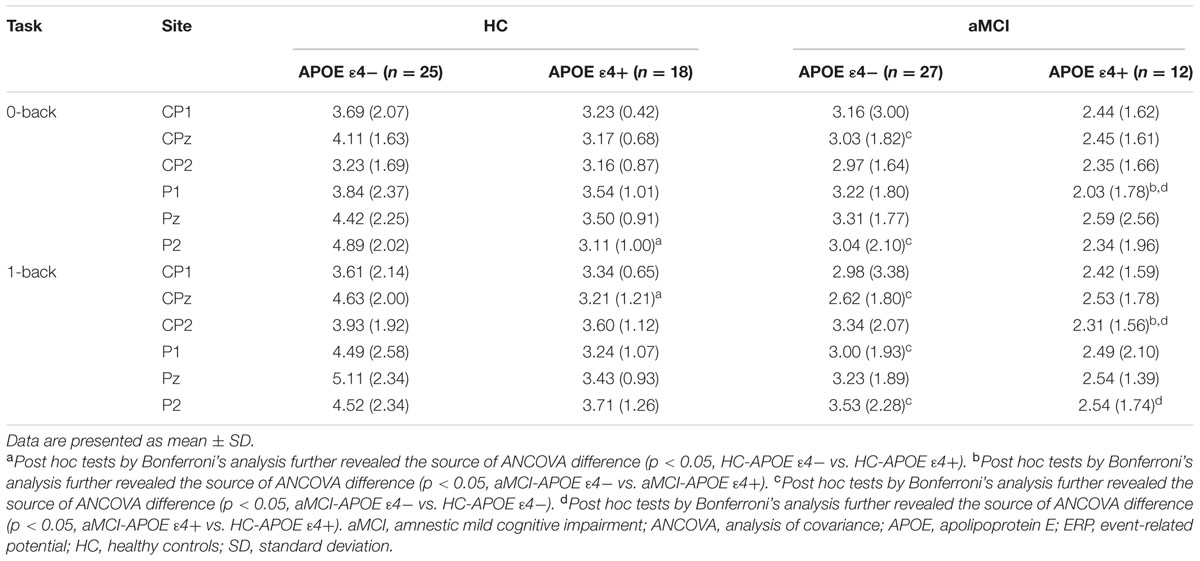
TABLE 3. Event-related potential (ERP) data (P300 amplitude) for HC and aMCI patients with different APOE ε4 status.
Based on the results of ERP components analysis, the correlation between cognitive assessment score and ERP components parameters (P300 amplitude) partialing out the effect of age, gender, years of education, and APOE genotype was calculated in HC and aMCI patients, respectively. It revealed positive correlations between P300 amplitude and information processing speed in both 0-back and 1-back tasks (0-back task: r = 0.504, p = 0.001 for HC and r = 0.475, p = 0.004 for aMCI patients, respectively; 1-back task: r = 0.346, p = 0.031 for HC and r = 0.368, p = 0.029 for aMCI patients, respectively). Moreover, a significantly positive correlation was revealed between P300 amplitude in 0-back task and executive function (r = 0.346, p = 0.031 for HC and r = 0.422, p = 0.012 for aMCI patients) (see Figure 3).
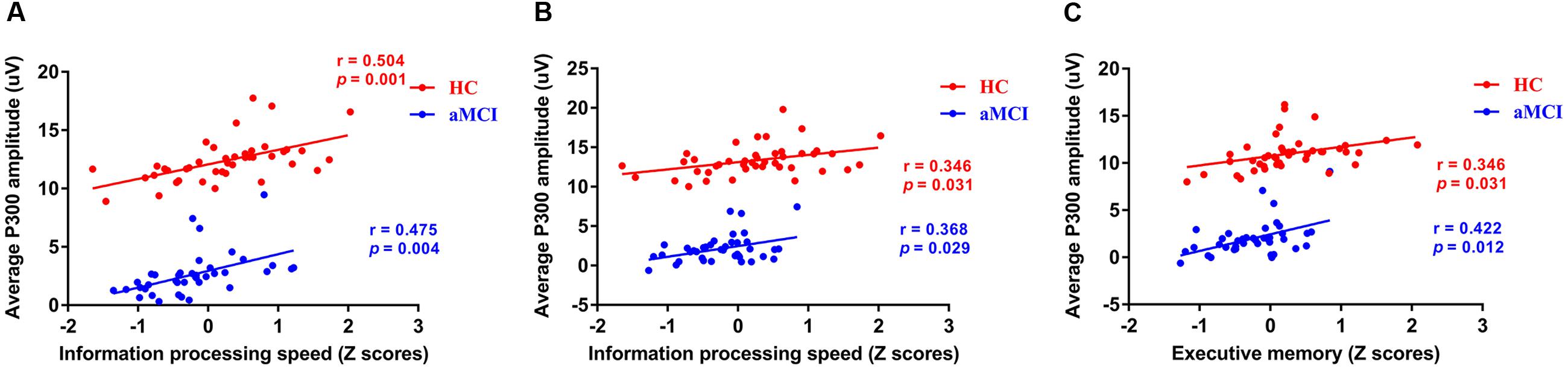
FIGURE 3. Scatterplots illustrating the correlation between P300 amplitude and Z scores of information processing speed in 0-back task (A), Z scores of information processing speed in 1-back task (B), and Z scores of executive function in 1-back task (C) in healthy controls and aMCI patients partialing out the effect of age, gender, years of education, and APOE ε4 status. Red circles represents HC. Blue circles represents aMCI patients. Abbreviations: aMCI, amnestic mild cognitive impairment; APOE, apolipoprotein E; HC, healthy controls.
Source Localization Analysis
Regarding the brain activation during VSWM, the differences between groups were displayed in Table 4. Brain regions that showed activation difference at <0.05 level were showed in Figure 4 (activation maxima are color coded in yellow). It showed greater brain activation in the right parahippocampal gyrus [PHG, Brodmann area (BA) 27] in aMCI-APOE ε4+ compared to aMCI-APOE ε4- group during P300 time range (see Table 4 and Figure 4). However, the difference in cortex activation between HC- APOE ε4+ and HC-APOE ε4- during P300 time range did not reach a significant level during P300 time range (see Table 4).
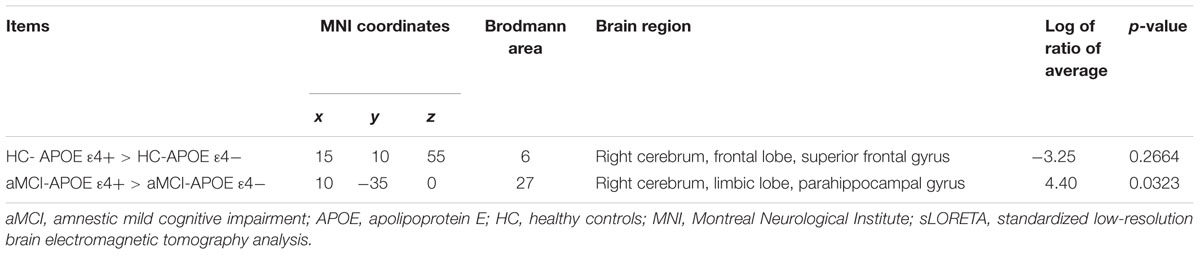
TABLE 4. Comparison of participants with different APOE ε4 status in sLORETA in HC and aMCI patients, respectively (voxels showing maximal difference).
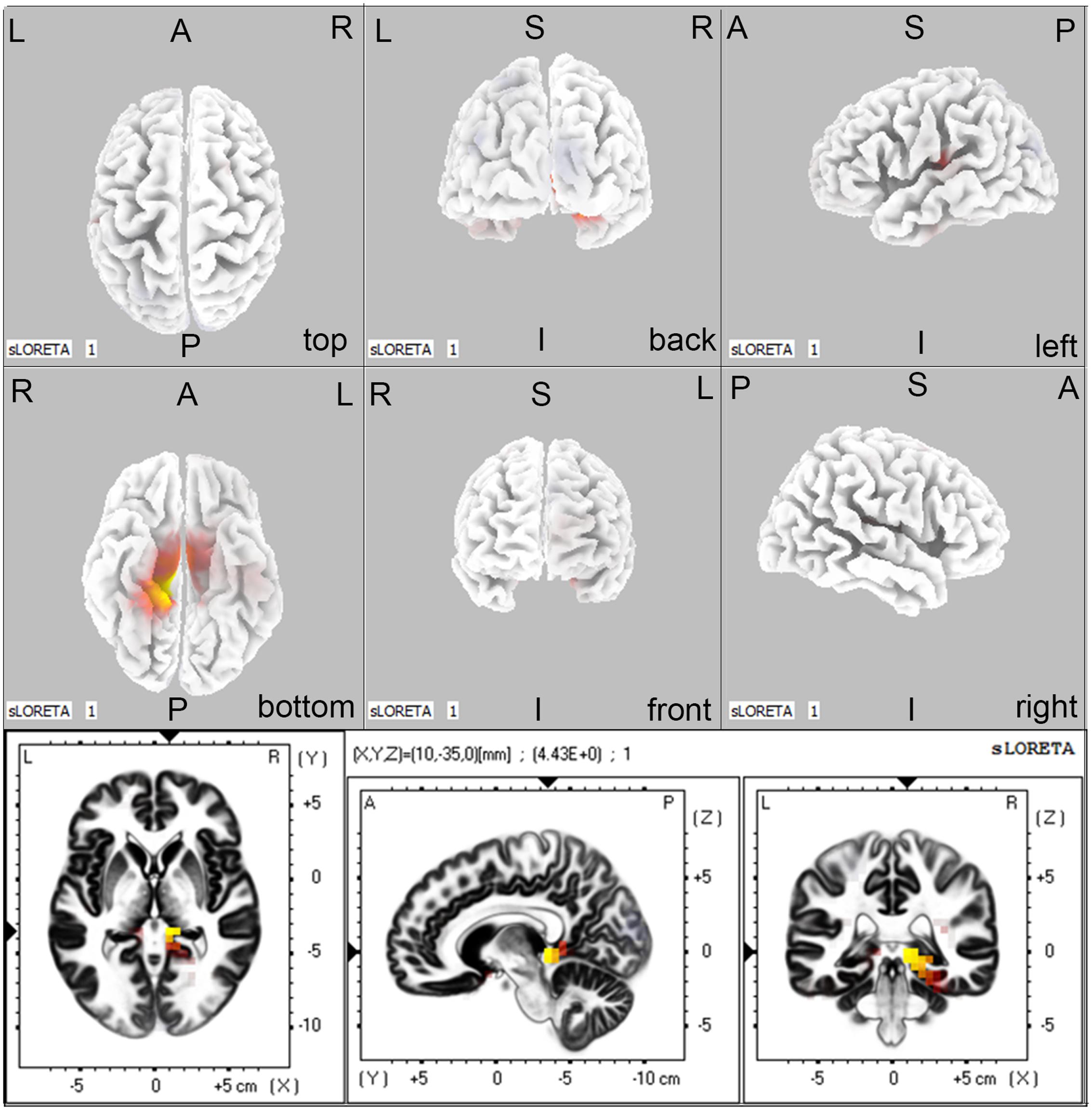
FIGURE 4. The sLORETA images showing statistical differences between aMCI-APOE ε4+ and aMCI-APOE ε4– group (three dimensional-view and slice-view) during the P300 time range. The three slice-view images below located the maximal difference between aMCI-APOE ε4+ and aMCI-APOE ε4– group (MNI coordinates x, y, z = 10, –35, 0). Activation maxima are color coded in yellow. These images represent greater brain activation in the right PHG in APOE ε4 carriers with respect to non-carriers in aMCI patients. Abbreviations: aMCI, amnestic mild cognitive impairment; APOE, apolipoprotein E; MNI, Montreal Neurological Institute; PHG, parahippocampal gyrus; sLORETA, standardized low-resolution brain electromagnetic tomography analysis.
Discussion
The current study reported that aMCI patients showed reduced accuracy, delayed mean correct RT and decreased P300 amplitude at central–parietal and parietal electrodes compared to HC. P300 alteration is the most widely investigated and best understood component change in ERP studies on AD patients. P300 component represents different cognitive processes during different tasks (Kirschner et al., 2015). P3b is an important signature of WM processes (Linden, 2005). Consistent with our investigation, previous studies have shown P300 amplitude reduction during WM task in aMCI patients (Newsome et al., 2013; Li et al., 2016). These studies indicate that decreased P300 amplitude could be a sensitive biomarker for identifying aMCI patients. Moreover, our study detected that P300 amplitude was related to information processing speed and executive function in both HC and aMCI patients. Brown et al. (2012) suggested that information processing affects ERP results via speed of encoding. In addition, Miyake et al. (2001) indicated that central executive function plays a critical role in WM. In summary, P300 amplitude could predict VSWM deficits in aMCI patients.
Notably, P300 amplitude significantly differed between APOE ε4 carriers and non-carriers in cognitively intact adults, distinct from the lack of performance difference between different APOE ε4 status. Moreover, according to our study, P300 amplitude declined prior to task performance change, supporting the viewpoint that P300 amplitude alteration might be a sensitive index for detecting VSWM deficits in APOE ε4 carriers. However, sLORETA results showed no significant brain activation difference between different APOE ε4 status during P300 time range. A fMRI study with VSWM task also reported a similar result (Alichniewicz et al., 2012), but it was inconsistent with another fMRI investigation, that detected lesser neural activation in the MTL during VSWM task in APOE ε4 carriers compared to non-carriers in HC (Borghesani et al., 2008). In addition, another fMRI study reported that APOE ε4 carriers in cognitively intact adults showed greater brain activation in the medial frontal cortex and PHG during WM task compared to APOE ε4 non-carriers (Filbey et al., 2010). Moreover, a fMRI study indicated that APOE ε4 carriers showed greater brain activation in the bilateral medial frontal and parietal regions and the right dorsolateral prefrontal cortex with respect to APOE ε4 non-carriers during WM (Wishart et al., 2006). Heterogeneity of included individuals and differences in tasks might be the sources of inconsistence. It was reported that APOE ε4 allele had a dose effect on AD risk (Corder et al., 1993; Hostage et al., 2013). In addition, a recent meta-analysis indicated that APOE 3/4 allele status had no effect on global cognitive ability, including visuospatial skills in cognitively healthy individuals (Wisdom et al., 2011). However, due to the limitation of sample size, the dose effect could not be explored in the investigation. Interestingly, APOE ε4 allele showed no effect on brain activation in cognitively intact adults during visuospatial N-back WM tasks (the present study and Alichniewicz et al.’s study), whereas brain activation alteration presented between APOE genotypes during other VSWM tasks [e.g., delayed match to sample (DMS) tasks] (Borghesani et al., 2008). Moreover, the different biological backgrounds of fMRI and sLORETA might provide additional explanation for the source localization difference (Herrmann and Debener, 2008). Blood oxygenation level-dependent (BOLD) signal reflects blood oxygenation changes associated with neural activation (mainly presynaptic) and is based on metabolic processes (Logothetis and Pfeuffer, 2004), whereas ERP corresponds to electrical neuronal activation (mainly postsynaptic). Additionally, a disadvantage of ERP is the limitation of deep brain monitoring (Rusiniak et al., 2013). As proposed above, the different biological characters and brain monitoring depth might explain the source localization difference acquired from LORETA and fMRI. In summary, P300 amplitude might sensitively assess the WM deficits for AD high-risk participants.
Moreover, aMCI-APOE ε4 carriers showed reduced P300 amplitude compared to non-carriers, whereas the two groups performed tasks at the same level. Laczó et al. (2009) demonstrated more profound SWM deficits in aMCI-APOE ε4 carriers compared to non-carriers. Additionally, our sLORETA investigation revealed significantly enhanced brain activation in the right PHG in APOE ε4 carriers compared to non-carriers during P300 time range. It was inconsistent with a fMRI study during a VSWM task, that indicated no significant brain activation difference between different APOE ε4 status in aMCI patients (Alichniewicz et al., 2012). Both Alichniewicz et al.’s (2012) study and the present study applied visuospatial N-back WM. Heterogeneity of included individuals and different biological backgrounds of fMRI and sLORETA might provide an interpretation for the inconsistent results. Right PHG is a part of MTL, which is composed of hippocampus, entorhinal, perirhinal, and parahippocampal cortex. MTL is a critical region for long-term memory. Recent findings revealed that MTL is essential for relational processing to form WM, related to encoding of WM and could predict subsequent recall (Olson et al., 2006a,b; Hartley et al., 2007; Axmacher et al., 2008; Garrett et al., 2011). In the present study, APOE ε4 carriers showed significantly enhanced brain activation in the right PHG compared to non-carriers, whereas no significant task performance difference was indicated between APOE ε4 carriers and non-carriers. The situation might result from neural compensation. Neural compensation refers to recruitment of additional, compensatory networks to accomplish the task after primary task-related networks afflicted by pathology (Barulli and Stern, 2013). Several fMRI studies are also consistent with the neural compensation hypothesis in older adults at risk for AD, although none specifically investigated the VSWM (Bookheimer et al., 2000; Bondi et al., 2005; Bassett et al., 2006; Han et al., 2007). Investigations on patterns of association between the right PHG activation and VSWM task performance in different APOE genotypes might further demonstrate the neural compensation hypothesis. However, due to the limitation of LORETA on source localization, the present studies could not provide more evidence for the neural compensation hypothesis. According to our study, reduced P300 amplitude could act as a biomarker for VSWM deficits in aMCI-APOE ε4 carriers, and neural compensation mechanism might contribute to the maintenance of task performance.
There are a few limitations in the investigation. The present study did not explore the dose effect and the effect of specific genotype among APOE ε4 non-carriers due to the limitation of sample size. It was reported that different effect on cognition was indicated between APOE ε2 carriers and participants with APOE ε3/ε3 genotype (Benjamin et al., 1994). Source localization with LORETA lacks high spatial resolution, simultaneous ERP-fMRI could solve the problem. This study explored brain activation difference during low-load WM task exclusively; a high-load task could also be investigated to detect the impact of APOE ε4 status on WM with different task loads.
Conclusion
The present investigation reported that aMCI patients showed reduced P300 amplitude compared to HC. Moreover, APOE ε4 status influenced P300 amplitude during VSWM in both non-demented adults and aMCI patients. However, the study did not detect task performance difference between APOE ε4 carriers and non-carriers, which might derive from neural compensation mechanism. Hence, the present investigation demonstrated that P300 amplitude could predict VSWM deficits in aMCI patients and contribute to early detection of VSWM deficits in APOE ε4 carriers compared to non-carriers.
Author Contributions
L-HG, L-JG, and JC were in charge of EEG recording, ERP data acquisition and analysis. HS, ZW, DL, and Y-NY were in charge of patient enrollment, neuropsychological assessments. L-HGu had the major responsibility for preparing the paper and manuscript writing. Z-JZ, S-JL, and JC contributed to the design and plan of the present study. Z-JZ supervised the project.
Conflict of Interest Statement
The authors declare that the research was conducted in the absence of any commercial or financial relationships that could be construed as a potential conflict of interest.
Acknowledgments
This study was supported by the National Natural Science Foundation of China (No. 81420108012; 81671046), the Key Program for Clinical Medicine and Science and Technology: Jiangsu Province Clinical Medical Research Center (No. BL2013025; BL2014077), the Disciplinary group of Psychology and Neuroscience, Xinxiang Medical University (2016PN-KFKT-01), the Fundamental Research Funds for the Central Universities and the Scientific Research Innovation Program for College and University Graduates of Jiangsu Province (KYZZ16_0131).
References
Albert, M. S., Dekosky, S. T., Dickson, D., Dubois, B., Feldman, H. H., Fox, N. C., et al. (2011). The diagnosis of mild cognitive impairment due to Alzheimer’s disease: recommendations from the National Institute on Aging-Alzheimer’s Association workgroups on diagnostic guidelines for Alzheimer’s disease. Alzheimers Dement. J. Alzheimers Association 7, 257–262. doi: 10.1016/j.jalz.2011.03.008
Alescio-Lautier, B., Michel, B. F., Herrera, C., Elahmadi, A., Chambon, C., Touzet, C., et al. (2007). Visual and visuospatial short-term memory in mild cognitive impairment and Alzheimer disease: role of attention. Neuropsychologia 45, 1948–1960. doi: 10.1016/j.neuropsychologia.2006.04.033
Alichniewicz, K. K., Brunner, F., Klünemann, H. H., and Greenlee, M. W. (2012). Structural and functional neural correlates of visuospatial information processing in normal aging and amnestic mild cognitive impairment. Neurobiol. Aging 33, 2782–2797. doi: 10.1016/j.neurobiolaging.2012.02.010
Axmacher, N., Schmitz, D. P., Weinreich, I., Elger, C. E., and Fell, J. (2008). Interaction of working memory and long-term memory in the medial temporal lobe. Cereb. Cortex 18, 2868–2878. doi: 10.1093/cercor/bhn045
Bai, F., Liao, W., Watson, D. R., Shi, Y., Yuan, Y., Cohen, A. D., et al. (2011). Mapping the altered patterns of cerebellar resting-state function in longitudinal amnestic mild cognitive impairment patients. J. Alzheimer’s Dis. 23, 87–99. doi: 10.3233/JAD-2010-101533
Barulli, D., and Stern, Y. (2013). Efficiency, capacity, compensation, maintenance, plasticity: emerging concepts in cognitive reserve. Trends Cogn. Sci. 17, 502–509. doi: 10.1016/j.tics.2013.08.012
Bassett, S. S., Yousem, D. M., Cristinzio, C., Kusevic, I., Yassa, M. A., Caffo, B. S., et al. (2006). Familial risk for Alzheimer’s disease alters fMRI activation patterns. Brain 129, 1229–1239. doi: 10.1093/brain/awl089
Benjamin, R., Leake, A., McArthur, F. K., Ince, P. G., Candy, J. M., Edwardson, J. A., et al. (1994). Protective effect of apoE epsilon 2 in Alzheimer’s disease. Lancet (London, England) 344, 473. doi: 10.1016/S0140-6736(94)91804-X
Bischkopf, J., Busse, A., and Angermeyer, M. C. (2002). Mild cognitive impairment–A review of prevalence, incidence and outcome according to current approaches. Acta Psychiatr. Scand. 106, 403–414. doi: 10.1034/j.1600-0447.2002.01417.x
Bondi, M. W., Houston, W. S., Eyler, L. T., and Brown, G. G. (2005). fMRI evidence of compensatory mechanisms in older adults at genetic risk for Alzheimer disease. Neurology 64, 501–508. doi: 10.1212/01.WNL.0000150885.00929.7E
Bookheimer, S. Y., Strojwas, M. H., Cohen, M. S., Saunders, A. M., Pericak-Vance, M. A., Mazziotta, J. C., et al. (2000). Patterns of brain activation in people at risk for Alzheimer’s disease. N. Engl. J. Med. 343, 450–456. doi: 10.1056/NEJM200008173430701
Borghesani, P. R., Johnson, L. C., Shelton, A. L., Peskind, E. R., Aylward, E. H., Schellenberg, G. D., et al. (2008). Altered medial temporal lobe responses during visuospatial encoding in healthy APOE∗4 carriers. Neurobiol. Aging 29, 981–991. doi: 10.1016/j.neurobiolaging.2007.01.012
Bretsky, P., Guralnik, J. M., Launer, L., Albert, M., and Seeman, T. E. (2003). The role of APOE-epsilon4, of APOE-epsilon4 in longitudinal cognitive decline: MacArthur studies of Successful Aging. Neurology 60, 1077–1081. doi: 10.1212/01.WNL.0000055875.26908.24
Brown, L. A., Brockmole, J. R., Gow, A. J., and Deary, I. J. (2012). Processing speed and visuospatial executive function predict visual working memory ability in older adults. Exp. Aging Res. 38, 1–19. doi: 10.1080/0361073X.2012.636722
Chen, J., Ma, W., Zhang, Y., Wu, X., Wei, D., Liu, G., et al. (2014a). Distinct facial processing related negative cognitive bias in first-episode and recurrent major depression: evidence from the N170 ERP component. PLoS ONE 9:e109176. doi: 10.1371/journal.pone.0109176
Chen, J., Ma, W., Zhang, Y., Yang, L. Q., Zhang, Z., Wu, X., et al. (2014b). Neurocognitive impairment of mental rotation in major depressive disorder: evidence from event-related brain potentials. J. Nervous Mental Dis. 202, 594–602. doi: 10.1097/NMD.0000000000000167
Chen, J., Yang, L., Ma, W., Wu, X., Zhang, Y., Wei, D., et al. (2013). Ego-rotation and object-rotation in major depressive disorder. Psychiatry Res. 209, 32–39. doi: 10.1016/j.psychres.2012.10.003
Chen, J., Zhang, Y., Wei, D., Wu, X., Fu, Q., Xu, F., et al. (2014c). Neurophysiological handover from MMN to P3a in first-episode and recurrent major depression. J. Affect. Disord. 174C, 173–179. doi: 10.1016/j.jad.2014.11.049
Corder, E. H., Saunders, A. M., Strittmatter, W. J., Schmechel, D. E., Gaskell, P. C., Small, G. W., et al. (1993). Gene dose of apolipoprotein E type 4 allele and the risk of Alzheimer’s disease in late onset families. Science (New York, NY) 261, 921–923. doi: 10.1126/science.8346443
Filbey, F. M., Chen, G., Sunderland, T., and Cohen, R. M. (2010). Failing compensatory mechanisms during working memory in older apolipoprotein E-ε4 healthy adults. Brain Imaging Behav. 4, 177–188. doi: 10.1007/s11682-010-9097-9
Garrett, A., Kelly, R., Gomez, R., Keller, J., Schatzberg, A. F., and Reiss, A. L. (2011). Aberrant brain activation during a working memory task in psychotic major depression. Am. J. Psychiatry 168, 173–182. doi: 10.1176/appi.ajp.2010.09121718
Gevins, A., and Cutillo, B. (1993). Spatiotemporal dynamics of component processes in human working memory 73. Electroencephalogr. Clin. Neurophysiol. 87, 128–143. doi: 10.1016/0013-4694(93)90119-G
Grundman, M., Petersen, R. C., Ferris, S. H., Thomas, R. G., Aisen, P. S., Bennett, D. A., et al. (2004). Mild cognitive impairment can be distinguished from Alzheimer disease and normal aging for clinical trials. Arch. Neurol. 61, 59–66. doi: 10.1001/archneur.61.1.59
Han, S. D., Houston, W. S., Jak, A. J., Eyler, L. T., Nagel, B. J., Fleisher, A. S., et al. (2007). Verbal paired-associate learning by APOE genotype in non-demented older adults: fMRI evidence of a right hemispheric compensatory response. Neurobiol. Aging 28, 238–247. doi: 10.1016/j.neurobiolaging.2005.12.013
Hartley, T., Bird, C. M., Chan, D., Cipolotti, L., Husain, M., Vargha-Khadem, F., et al. (2007). The hippocampus is required for short-term topographical memory in humans. Hippocampus 17, 34–48. doi: 10.1002/hipo.20240
Herrmann, C. S., and Debener, S. (2008). Simultaneous recording of EEG and BOLD responses: a historical perspective. Int. J. Psychophysiol. 67, 161–168. doi: 10.1016/j.ijpsycho.2007.06.006
Hodges, J. R., Erzinclioglu, S., and Patterson, K. (2006). Evolution of cognitive deficits and conversion to dementia in patients with mild cognitive impairment: a very-long-term follow-up study. Dement. Geriatr. Cogn. Disord. 21, 380–391. doi: 10.1159/000092534
Hostage, C. A., Roy, C. K., Doraiswamy, P. M., and Petrella, J. R. (2013). Dissecting the gene dose-effects of the APOE ε4 and ε2 alleles on hippocampal volumes in aging and Alzheimer’s disease. PLoS ONE 8:e54483. doi: 10.1371/journal.pone.0054483
Irimajiri, R., Golob, E. J., and Starr, A. (2010). ApoE genotype and abnormal auditory cortical potentials in healthy older females. Neurobiol. Aging 31, 1799–1804. doi: 10.1016/j.neurobiolaging.2008.09.005
Jiu, C., Lai-Qi, Y., Zhi-Jun, Z., Wen-Tao, M., Xing-Qu, W., Xiang-Rong, Z., et al. (2013). The association between the disruption of motor imagery and the number of depressive episodes of major depression. J. Affect. Disord. 150, 337–343. doi: 10.1016/j.jad.2013.04.015
Jung, T. P., Makeig, S., Westerfield, M., Townsend, J., Courchesne, E., and Sejnowski, T. J. (2000). Removal of eye activity artifacts from visual event-related potentials in normal and clinical subjects. Clin. Neurophysiol. 111, 1745–1758. doi: 10.1016/S1388-2457(00)00386-2
Keeser, D., Padberg, F., Reisinger, E., Pogarell, O., Kirsch, V., Palm, U., et al. (2011). Prefrontal direct current stimulation modulates resting EEG and event-related potentials in healthy subjects: a standardized low resolution tomography (sLORETA) study. Neuroimage 55, 644–657. doi: 10.1016/j.neuroimage.2010.12.004
Kirschner, A., Cruse, D., Chennu, S., Owen, A. M., and Hampshire, A. (2015). A P300-based cognitive assessment battery. Brain Behav. 5, e00336. doi: 10.1002/brb3.336
Klunk, W. E., Engler, H., Nordberg, A., Wang, Y., Blomqvist, G., Holt, D. P., et al. (2004). Imaging brain amyloid in Alzheimer’s disease with Pittsburgh Compound-B. Ann. Neurol. 55, 306–319. doi: 10.1002/ana.20009
Kochan, N. A., Breakspear, M., Slavin, M. J., Valenzuela, M., McCraw, S., Brodaty, H., et al. (2010). Functional alterations in brain activation and deactivation in mild cognitive impairment in response to a graded working memory challenge. Dement. Geriatr. Cogn. Disord. 30, 553–568. doi: 10.1159/000322112
Laczó, J., Vlček, K., Vyhnálek, M., Mat´oška, V., Buksakowska, I., Magerová, H., et al. (2009). APOE E4 affects spatial working memory and attention in patients with amnestic mild cognitive impairment. Alzheimers Dement. 5, 357. doi: 10.1016/j.jalz.2009.04.1129
Leyhe, T., and Muller, S. M. (2009). Impairment of episodic and semantic autobiographical memory in patients with mild cognitive impairment and early Alzheimer’s disease. Neuropsychologia 47, 2464–2469. doi: 10.1016/j.neuropsychologia.2009.04.018
Li, B. Y., Tang, H. D., and Chen, S. D. (2016). Retrieval deficiency in brain activity of working memory in amnesic mild cognitive impairment patients: a brain event-related potentials study. Front. Aging Neurosci. 8:54. doi: 10.3389/fnagi.2016.00054
Linden, D. E. J. (2005). The P300: where in the brain is it produced and what does it tell us? Neuroscientist 11, 563–576.
Logothetis, N. K., and Pfeuffer, J. (2004). On the nature of the BOLD fMRI contrast mechanism. Magn. Reson. Imaging 22, 1517–1531. doi: 10.1016/j.mri.2004.10.018
Lou, W., Shi, L., Wang, D., Tam, C. W. C., Chu, W. C. W., Mok, V. C. T., et al. (2015). Decreased activity with increased background network efficiency in amnestic MCI during a visuospatial working memory task. Hum. Brain Mapp. 36, 3387–3403. doi: 10.1002/hbm.22851
Mitolo, M., Gardini, S., Fasano, F., Crisi, G., Pelosi, A., Pazzaglia, F., et al. (2013). Visuospatial memory and neuroimaging correlates in mild cognitive impairment. J. Alzheimer’s Dis. 35, 75–90. doi: 10.3233/JAD-121288
Miyake, A., Friedman, N. P., Rettinger, D. A., Shah, P., and Hegarty, M. (2001). How are visuospatial working memory, executive functioning, and spatial abilities related? A latent-variable analysis. J. Exp. Psychol. Gen. 130, 621–640. doi: 10.1037/0096-3445.130.4.621
Nakao, Y., Kodabashi, A., Yarita, M., and Fujimoto, T. (2012). “Temporal activities during P3 components on the working memory-related brain regions: N-back ERP study,” in Proceedings of the IEEE-EMBS International Conference on Biomedical and Health Informatics, Piscataway NJ, 424–427. doi: 10.1109/bhi.2012.6211606
Neuhaus, A. H., Popescu, F. C., Grozea, C., Hahn, E., Hahn, C., Opgen-Rhein, C., et al. (2011). Single-subject classification of schizophrenia by event-related potentials during selective attention. Neuroimage 55, 514–521. doi: 10.1016/j.neuroimage.2010.12.038
Newsome, R. N., Pun, C., Smith, V. M., Ferber, S., and Barense, M. D. (2013). Neural correlates of cognitive decline in older adults at-risk for developing MCI: evidence from the CDA and P300. Cogn. Neurosci. 4, 152–162. doi: 10.1080/17588928.2013.853658
Nunez, P. L., and Srinivasan, R. (2006). Electric Fields of the Brain : The Neurophysics of EEG. Oxford: Oxford University Press. doi: 10.1093/acprof:oso/9780195050387.001.0001
Olson, I. R., Moore, K. S., Stark, M., and Chatterjee, A. (2006a). Visual working memory is impaired when the medial temporal lobe is damaged. J. Cogn. Neurosci. 18, 1087–1097.
Olson, I. R., Page, K., Moore, K. S., Chatterjee, A., and Verfaellie, M. (2006b). Working memory for conjunctions relies on the medial temporal lobe. J. Neurosci 26, 4596–4601.
Pandey, A. K., Kamarajan, C., Tang, Y., Chorlian, D. B., Roopesh, B. N., Manz, N., et al. (2012). Neurocognitive deficits in male alcoholics: an ERP/sLORETA analysis of the N2 component in an equal probability Go/NoGo task. Biol. Psychol. 89, 170–182. doi: 10.1016/j.biopsycho.2011.10.009
Papageorgiou, C., Ventouras, E., Uzunoglu, N., Rabavilas, A., and Stefanis, C. (2002). Changes of P300 elicited during a working memory test in individuals with depersonalization-derealization experiences. Neuropsychobiology 46, 70–75. doi: 10.1159/000065415
Pascual-Marqui, R. D. (2002). Standardized low-resolution brain electromagnetic tomography (sLORETA): technical details. Methods Find. Exp. Clin. Pharmacol. 24, 5–12.
Petersen, R. C. (2004). Mild cognitive impairment as a diagnostic entity. J. Intern. Med. 256, 183–194. doi: 10.1111/j.1365-2796.2004.01388.x
Petersen, R. C., Jack, C. R. Jr., Xu, Y. C., Waring, S. C., O’Brien, P. C., Smith, G. E., et al. (2000). Memory and MRI-based hippocampal volumes in aging and AD. Neurology 54, 581–587. doi: 10.1212/WNL.54.3.581
Petersen, R. C., Smith, G. E., Waring, S. C., Ivnik, R. J., Tangalos, E. G., and Kokmen, E. (1999). Mild cognitive impairment: clinical characterization and outcome. Arch. Neurol. 56, 303–308. doi: 10.1001/archneur.56.3.303
Polich, J. (2007). Updating P300: an integrative theory of P3a and P3b. Clin. Neurophysiol. 118, 2128–2148. doi: 10.1016/j.clinph.2007.04.019
Reiman, E. M., Chen, K., Alexander, G. E., Caselli, R. J., Bandy, D., Osborne, D., et al. (2005). Correlations between apolipoprotein E epsilon4 gene dose and brain-imaging measurements of regional hypometabolism. Proc. Natl. Acad. Sci. U.S.A. 102, 8299–8302. doi: 10.1073/pnas.0500579102
Rusiniak, M., Lewandowska, M., Wolak, T., Pluta, A., Milner, R., Ganc, M., et al. (2013). A modified oddball paradigm for investigation of neural correlates of attention: a simultaneous ERP-fMRI study. Magma (New York, NY) 26, 511–526. doi: 10.1007/s10334-013-0374-7
Saunders, N., and Summers, M. J. (2011). Longitudinal deficits to attention, executive, and working memory in subtypes of mild cognitive impairment. Neuropsychology 25, 237–248. doi: 10.1037/a0021134
Seripa, D., Panza, F., Franceschi, M., D’Onofrio, G., Solfrizzi, V., Dallapiccola, B., et al. (2009). Non-apolipoprotein E and apolipoprotein E genetics of sporadic Alzheimer’s disease. Ageing Res. Rev. 8, 214–236. doi: 10.1016/j.arr.2008.12.003
Shi, F., Liu, B., Zhou, Y., Yu, C., and Jiang, T. (2009). Hippocampal volume and asymmetry in mild cognitive impairment and Alzheimer’s disease: Meta-analyses of MRI studies. Hippocampus 19, 1055–1064. doi: 10.1002/hipo.20573
Shishkin, S. L., Ganin, I. P., and Kaplan, A. Y. (2011). Event-related potentials in a moving matrix modification of the P300 brain-computer interface paradigm. Neurosci. Lett. 496, 95–99. doi: 10.1016/j.neulet.2011.03.089
Shu, H., Shi, Y., Chen, G., Wang, Z., Liu, D., Yue, C., et al. (2016). Opposite neural trajectories of apolipoprotein E 4 and 2 alleles with aging associated with different risks of Alzheimer’s Disease. Cereb. Cortex 26, 1421–1429. doi: 10.1093/cercor/bhu237
Shu, H., Yuan, Y., Xie, C., Bai, F., You, J., Li, L., et al. (2014). Imbalanced hippocampal functional networks associated with remitted geriatric depression and apolipoprotein E epsilon4 allele in nondemented elderly: a preliminary study. J. Affect. Disord. 164, 5–13. doi: 10.1016/j.jad.2014.03.048
Sokhadze, E., Stewart, C., Hollifield, M., and Tasman, A. (2008). Event-related potential study of executive dysfunctions in a speeded reaction task in cocaine addiction. J. Neurother. 12, 185–204. doi: 10.1080/10874200802502144
Su, F., Shu, H., Ye, Q., Wang, Z., Xie, C., Yuan, B., et al. (2017). Brain insulin resistance deteriorates cognition by altering the topological features of brain networks. Neuroimage Clin. 13, 280–287. doi: 10.1016/j.nicl.2016.12.009
Suchan, B., Pickenhagen, A., and Daum, I. (2005). Effect of working memory on evaluation-related frontocentral negativity. Behav. Brain Res. 160, 331–337. doi: 10.1016/j.bbr.2004.12.014
Twamley, E. W., Ropacki, S. A., and Bondi, M. W. (2006). Neuropsychological and neuroimaging changes in preclinical Alzheimer’s disease. J. Int. Neuropsychol. Soc 12, 707–735. doi: 10.1017/S1355617706060863
Ward, D. D., Summers, M. J., Saunders, N. L., Janssen, P., Stuart, K. E., and Vickers, J. C. (2014). APOE and BDNF Val66Met polymorphisms combine to influence episodic memory function in older adults. Behav. Brain Res. 271, 309–315. doi: 10.1016/j.bbr.2014.06.022
Watter, S., Geffen, G. M., and Geffen, L. B. (2001). The n -back as a dual-task: P300 morphology under divided attention. Psychophysiology 38, 998–1003. doi: 10.1111/1469-8986.3860998
Webster, S. J., Bachstetter, A. D., Nelson, P. T., Schmitt, F. A., and Van Eldik, L. J. (2014). Using mice to model Alzheimer’s dementia: an overview of the clinical disease and the preclinical behavioral changes in 10 mouse models. Front. Genet. 5:88. doi: 10.3389/fgene.2014.00088
Webster, S. J., Bachstetter, A. D., and Van Eldik, L. J. (2013). Comprehensive behavioral characterization of an APP/PS-1 double knock-in mouse model of Alzheimer’s disease. Alzheimer’s Res. Ther. 5, 28. doi: 10.1186/alzrt182
Wisdom, N. M., Callahan, J. L., and Hawkins, K. A. (2011). The effects of apolipoprotein E on non-impaired cognitive functioning: a meta-analysis. Neurobiol. Aging 32, 63–74. doi: 10.1016/j.neurobiolaging.2009.02.003
Wishart, H. A., Saykin, A. J., Rabin, L. A., Santulli, R. B., Flashman, L. A., Guerin, S. J., et al. (2006). Increased brain activation during working memory in cognitively intact adults with the APOE epsilon4 allele. Am. J. Psychiatry 163, 1603–1610. doi: 10.1176/ajp.2006.163.9.1603
Yu, R., and Zhang, P. (2014). Neural evidence for description dependent reward processing in the framing effect. Front. Neurosci. 8:56. doi: 10.3389/fnins.2014.00056
Keywords: apolipoprotein E, event-related potential, amnestic mild cognitive impairment, visuospatial working memory, P300
Citation: Gu L-H, Chen J, Gao L-J, Shu H, Wang Z, Liu D, Yan Y-N, Li S-J and Zhang Z-J (2017) The Effect of Apolipoprotein E ε4 (APOE ε4) on Visuospatial Working Memory in Healthy Elderly and Amnestic Mild Cognitive Impairment Patients: An Event-Related Potentials Study. Front. Aging Neurosci. 9:145. doi: 10.3389/fnagi.2017.00145
Received: 26 October 2016; Accepted: 30 April 2017;
Published: 17 May 2017.
Edited by:
Hanting Zhang, West Virginia University, United StatesReviewed by:
Susana Cid-Fernández, Universidade de Santiago de Compostela, SpainChaur-Jong Hu, Taipei Medical University, Taiwan
Copyright © 2017 Gu, Chen, Gao, Shu, Wang, Liu, Yan, Li and Zhang. This is an open-access article distributed under the terms of the Creative Commons Attribution License (CC BY). The use, distribution or reproduction in other forums is permitted, provided the original author(s) or licensor are credited and that the original publication in this journal is cited, in accordance with accepted academic practice. No use, distribution or reproduction is permitted which does not comply with these terms.
*Correspondence: Zhi-Jun Zhang, janemengzhang@vip.163.com Jiu Chen, ericcst@aliyun.com