- 1Dipartimento di Studi Umanistici, Università degli Studi di Napoli Federico II, Napoli, Italy
- 2IRCCS Fondazione Santa Lucia, Roma, Italy
- 3Centro Fermi - Museo storico della fisica e Centro studi e ricerche Enrico Fermi, Rome, Italy
- 4Università degli Studi di Roma Tor Vergata, Roma, Italy
- 5Clinica Neurologica III, Dipartimento di Scienze della Salute, Università degli Studi di Milano, Milan, Italy
Over the last 20 years, major advances in cognitive neuroscience have clearly shown that the language function is not restricted into the classical language areas but it involves brain regions, which had never previously considered. Indeed, recent lines of evidence have suggested that the processing of words associated to motor schemata, such as action verbs, modulates the activity of the sensorimotor cortex, which, in turn, facilitates its retrieval. To date, no studies have investigated whether the spinal cord, which is functionally connected to the sensorimotor system, might also work as an auxiliary support for language processing. We explored the combined effect of transcutaneous spinal direct current stimulation (tsDCS) and language treatment in a randomized double-blind design for the recovery of verbs and nouns in 14 chronic aphasics. During each treatment, each subject received tsDCS (20 min, 2 mA) over the thoracic vertebrae (10th vertebra) in three different conditions: (1) anodic, (2) cathodic and (3) sham, while performing a verb and noun naming tasks. Each experimental condition was run in five consecutive daily sessions over 3 weeks. Overall, a significant greater improvement in verb naming was found during the anodic condition with respect to the other two conditions, which persisted at 1 week after the end of the treatment. No significant differences were present for noun naming among the three conditions. The hypothesis is advanced that anodic tsDCS might have influenced activity along the ascending somatosensory pathways, ultimately eliciting neurophysiological changes into the sensorimotor areas which, in turn, supported the retrieval of verbs. These results further support the evidence that action words, due to their sensorimotor semantic properties, are partly represented into the sensorimotor cortex. Moreover, they also document, for the first time, that tsDCS enhances verb recovery in chronic aphasia and it may represent a promising new tool for language treatment.
Introduction
Since the late nineteenth century, it has long been assumed that the language function is hierarchically organized into specific cortical areas, the Broca’s and Wernicke’s areas (1). However, over the past decades, several lines of evidence have shown that the language faculty not only engages a number of cortical and subcortical regions that extend far beyond the classical areas [for reviews, see for example (2, 3)] but it is also represented within regions that had never been considered before to support language [for review see Ref. (4)]. Accordingly, instead of considering the language faculty as completely modularized, behavioral and neuroimaging results have shown that the network subserving the language function is largely distributed across the brain (5, 6). In the embodied cognition view, the representation of a concept is crucially dependent upon the sensory–motor properties belonging to that concept (7–9). Indeed, the hypothesis has been advanced that action verbs are mentally represented in different semantic representations among which the sensorimotor features to perform the action (10–12). This implies that the sensory–motor regions of the brain may also process action concepts. Several lines of evidence have already suggested that the sensorimotor cortex takes part in language processing, at least when speech is translated into sensorimotor acts (10, 13, 14). Indeed, much of this evidence comes from studies that used action verbs (individually presented or embedded in sentences) as stimuli [e.g., Ref. (13, 15, 16)]. Accordingly, when people listen to verbal description of actions, their somatosensory, motor, and premotor neural populations are activated as they are actually performing the corresponding actions [5–3, for reviews see Ref. (4, 17)]. Recently, slower hand motor responses have been shown during processing of nouns referring to hand-related objects [(11, 12); see also Ref. (18)].
Thus, the retrieval of words associated with motor schemata, like swimming, is thought to rely in part in sensorimotor regions of the brain. Similarly, manipulable nouns (i.e., a pen), which recruit motor representations (i.e., writing), are partly processed in the same regions (11, 12).
Together with this more interactive view of language processing, the traditional concept of the spinal cord as a hardwired system that automatically respond to motor commands from the brain and to sensory inputs from the periphery has changed over time. Indeed, a large body of evidence has shown that this structure not only produces a variety of specialized movements but also acquires and memorizes new behaviors [see Ref. (19) for review].
However, in spite of a remarkable energy in considering the spinal cord as an active system which possesses capacities for neuronal and synaptic plasticity for the recovery of chronic pain and motor deficits (20, 21), as far as we know, no studies have considered whether it might also contribute as an auxiliary support for language processing.
Actually, although some earlier studies on acute traumatic spinal cord injury (SCI) patients have documented the presence of cognitive deficits in different domains, such as attention, executive functioning, memory, and language (22–27), none of them reported sufficient evidence that the cognitive impairment was specifically related to SCI. Indeed, factors contributing to those deficits were varied. Some patients had concomitant traumatic brain injury at the time of their accidents (26). Others had a history of cerebral vascular insufficiency (27). In addition, the long-term cognitive effects of alcohol and substance abuse, which have been found to approach a prevalence of 50% in the SCI population, may have contributed to cognitive or behavioral disorders (24, 25).
Given the wide variability of cortical lesions among aphasic patients, it is not always easy to localize through non-invasive brain stimulation techniques, such as transcranial direct current stimulation (tDCS), the optimal stimulation cortical sites, unless we use additional very expensive methodologies, such as neuroimaging and/or modeling (28, 29). This points to the urgency of considering other vicarious systems, functionally connected to the brain, that, when stimulated, contribute to the recovery of language.
Indeed, it has been shown that the application of tDCS over the motor cortex influences brain excitability, and hence can also modulate the spinal cord (30–32). By developing a constant low-intensity current (1–2 mA) through two large electrodes located on the targeted areas, tDCS increases or reduces cortical excitability after anodal or cathodal stimulation, respectively, possibly by inducing depolarization or hyperpolarization of the neuronal membrane resting potential (33–36). Accordingly, in a series of experiments performed in healthy subjects, Roche et al. (37, 38) showed that anodal tDCS over the motor cortex modified spinal network excitability by increasing disynaptic inhibition of spinal motoneurons. The authors suggested that the increase of disynaptic inhibition relies on an increase of disynaptic interneuron excitability and that tDCS over the motor cortex in human subjects induces effects on the spinal network. Similarly, Di Lazzaro et al. (39) found that 20 min of anodal tDCS over the primary motor cortex lead to a pronounced increase of D wave. Since the D wave is produced by direct activation of corticospinal axons (39), the authors concluded that anodal tDCS induced changes in excitability in corticospinal projections. Indeed, in patients with SCI, spinal plasticity induced by anodal tDCS over the motor cortex may promote the effects of locomotor training through modulation of spinal interneurons (32). On the other hand, it has also been shown that spinal manipulation, in stroke patients who are recovering from muscle degrading dysfunctions, leads to changes in cortical excitability, as measured by significant larger movement related cortical potential amplitudes after the treatment (40).
Therefore, given this strong reciprocal connections between the cortex and the spinal cord, we might assume that the stimulation of the spinal cord could influence activity into the sensorimotor cortex, through the ascending spinal pathways, which, in turn, might facilitate language processing (4, 16–18, 41).
To our knowledge, the impact of transcutaneous spinal direct current stimulation (tsDCS) on language recovery has not been investigated so far. A number of studies have already used tsDCS for modulating spinal cord activity in humans along the lemniscal pathway and nociceptive spinal system [(42–44), see Ref. (45) for review]. In most of these studies, the active electrode was placed over the thoracic vertebrae (T10–T12) and the reference electrode above the right arm while the current (2–3 mA) was delivered for 20–30 min (42–44, 46). Different from tDCS studies in which the anode applied over the cortical areas increases cortical excitability (30, 39, 47), in those works, anodal tsDCS had an overall inhibitory effect on spinal cord activity, while cathodal tsDCS did not produce polarity-specific effects (42, 43, 48). Interestingly for our study, it has also been shown that anodal tsDCS might elicit neurophysiological changes into the brain, through the activation of tonic afferent systems to the cortex (46–49). Indeed, Bocci et al. (46) assessed changes in intracortical excitability in 10 healthy subjects following tsDCS (2 mA, 20 min) over the thoracic vertebrae (T9–T11 level) by evaluating changes in motor-evoked potentials (MEPs) recorded from the first digital interosseus and the tibialis anterior muscles. Results showed that tsDCS was able to modulate intracortical excitability in a polarity-specific manner. Anodal tsDCS decreased MEP amplitudes, while cathodal tsDCS elicited opposite effects. Similarly, the same authors found that anodal tsDCS (T9–T11 level, 2 mA, 20 min) increased transcallosal conduction time and interhemispheric delay in motor connectivity, leading to a functional disconnection between hemispheres (49).
Therefore, given that one of the main function of the spinal cord is to translate sensory information into motor output and that tsDCS exerts its influence also into the brain (46–49), it is possible that tsDCS contributes to the recovery of language, in particular for those words characterized by motor properties, such as action verbs (i.e., to bite). Conversely, nouns not typically related to specific action (i.e., the cloud) would not activate the motor pathways and, therefore, should not benefit of this facilitation (11, 12).
In the present study, 14 aphasic participants underwent a daily language treatment for their word retrieval difficulties while delivering tsDCS. To ensure that the effects were specific for spinal cord stimulation and, therefore, that we were acting as far as possible from the cortex, we chose to stimulate the thoracic level. Based on previous results (11, 12), we expected to find a beneficial effect on response accuracy and vocal reaction times only for verbs.
Materials and Methods
Participants
Fourteen chronic aphasic subjects (nine males and five females) who had suffered a single left hemisphere stroke were included in the study. Inclusion criteria were native Italian proficiency, pre-morbid right handedness, a single left hemispheric stroke at least 6 months prior to the investigation, and a mild comprehension impairment score equal to or greater than 14/36 (50). Subjects more than 75 years old with epileptic seizures, previous brain lesions, possible spinal cord comorbidities, and with any type of implanted electronic device (e.g., pacemaker) were excluded. None of the participants have received structured language therapy for at least 6 months before the time of inclusion in the study in order to prevent confounding therapy effects.
Ethical Approval
The data analyzed in the current study were collected in accordance with the Helsinki Declaration and the Institutional Review Board of the IRCCS Fondazione Santa Lucia, Rome, Italy. Prior to participation, all patients signed informed consent forms.
Clinical Data
In all patients, the MRI revealed an ischemic lesion involving the left hemisphere (see Figure 1). The aphasic disorders were assessed using standardized language tests [the Battery for the Analysis of Aphasic Disorders, BADA test (51)]; Token Test (50). In order to ensure good cooperation during the treatment, subjects were recruited only if their score at the Token test revealed mild comprehension skills (score equal to or greater than 14/36). Subjects were also administered a memory test [i.e., digit span (52)] and a computerized Battery for Attentional Performance (53), which excluded the presence of working memory and attention deficits that might have confounded the data. The 14 subjects were classified as non-fluent aphasics because of their reduced spontaneous speech with short sentences and frequent word-finding difficulties. They had no articulatory deficits with preserved word repetition and reading. In a naming task, all patients had lexical retrieval difficulties [BADA test (51)] (see Table 1).
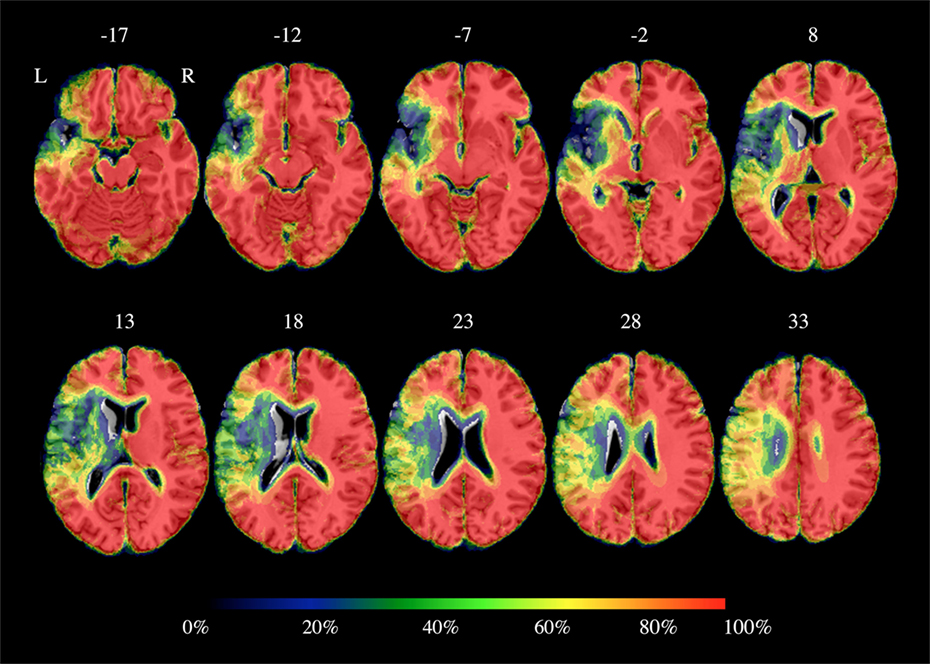
Figure 1. Percentage brain parenchyma overlap across patients. Color bar refers to the amount of spared voxels: 0% corresponds to total loss of cortical gray matter and 100% to preserved cortical gray matter. As shown, the highest damage was located into the left inferior frontal gyrus, the left temporal lobe and the left insula and it partially included the left precentral and postcentral gyri. Axial coordinates refer to the standard space (MNI152).
In summary, all patients were right handed before stroke, in a chronic phase, with a single left hemispheric stroke and no epileptic seizures. They were all classified as non-fluent aphasics with mild comprehension skills and no articulatory deficits.
Materials
45 action verbs [15 related to hand (e.g., to knock), 15 to mouth (e.g., to bite), and 15 to body actions (e.g., to dance)] and 45 non-manipulable nouns (e.g., clouds) were used. Nouns and actions were matched for number of letters, surface frequency (54), imageability (estimated on the basis of a sample of 20 normal participants along a seven-point scale) and age of acquisition [estimated on the basis of a sample of 20 normal participants along a nine-point scale (55)]. Both imageability and age-of-acquisition ratings were collected by asking volunteers to judge printed words.
Procedure
Transcutaneous Spinal Direct Current Stimulation
Transcutaneous spinal direct current stimulation was delivered using a battery driven Eldith (neuroConn GmbH Programmable Direct Current Stimulator, Germany) with a pair of surface-soaked sponge electrodes (5 cm × 7 cm). As in previous studies (46–49), a constant current of 2 mA intensity was applied through the active electrode on the 10th thoracic vertebra (spanned from the ninth to the 11th thoracic vertebrae) for 20 min while the reference electrode was placed over the right shoulder on the deltoid muscle (48). Indeed, several studies investigating corticospinal excitability (56–61) have suggested that longer-lasting robust effects are usually found with higher intensities (2 mA) (61) and longer (≥10 min) durations (61). Three different stimulation conditions were carried out: (1) anodic, (2) cathodic, and (3) sham. Sham stimulation was performed exactly like the other two conditions but the stimulator was turned off after 30 s (61). All patients underwent the three stimulation conditions whose order was randomized across subjects. To ensure the double-blind procedure, both the experimenter and the patient were blinded regarding the stimulation condition and the stimulator was turned on/off by another person. For each category (verbs vs. nouns), stimuli were subdivided into three lists of 15 items each matched for frequency, length, imaginability, and age of acquisition. For the three lists of verbs, each list included five hand-, five mouth-, and five body-related actions matched for the different variables. Both for the noun and the verb naming task, the assignment of each list to each stimulation condition (anodic vs. cathodic vs. sham) was randomized across conditions.
Word Retrieval Training
Once the electrodes were placed, subjects performed the naming task while they received 20 min of tsDCS. For each treatment, subjects were asked to name each picture that appeared on the PC screen (screen size 15″, viewing distance 1 m) for 20 s preceeded by a fixation point, which lasted 800 ms [see also Ref. (59) for similar procedure]. Only if the subject spontaneously correctly named the picture, the examiner manually recorded the response type on a separate sheet. If the subject failed or did not answer within 20 s, the corresponding written name was presented below the picture for 5 s and the subject was asked to read the word aloud. The pair of stimuli remained on the screen until the subject read the word or 5 s elapsed. In all cases, subjects were able to correctly read the word. Vocal reaction times were calculated from the presentation of the picture to the pronunciation of the first phoneme through Audacity 2.1.2 Software. Only the correctly pronounced words were considered. Each stimulation condition was performed in five consecutive daily sessions over three weeks with 6 days of intersession interval. The order of items presentation was randomized across sessions. To measure baseline performance, for each condition, three days before the training each subject was asked to name the pictures, one at a time, without help. At 1 week after each stimulation condition, all subjects were again shown the corresponding list of items and asked to name them without help. As before, the examiner manually recorded the answers (see Figure 2).
Data Analysis
Data were analyzed with SPSS 17.0 software. Statistical analyses were performed with two separate analyses of variances for response accuracy and vocal reaction times with three within-subject factors [WORDS CATEGORY (nouns vs. verbs)], TIME [baseline (T0) vs. end of training (T5) vs. follow-up (FU)] and CONDITION (anodal vs. cathodal vs. sham). If the analysis of variance (ANOVA) showed significant effects, respective post hoc Bonferroni tests were conducted. For all analyses, p-values < 0.05 were considered as statistically significant. All subjects well tolerated the experiment and none reported side effects or adverse reaction to the protocol.
Results
Accuracy
The analysis showed a significant effect of WORD CATEGORY [F(1, 13) = 36.60, p < 0.001], TIME [F(2, 26) = 102.92, p < 0.001], and CONDITION [F(2, 26) = 6.61, p = 0.005]. Overall, the percentage of correct responses for nouns was greater compared to verbs (nouns: mean = 59%, SD = 27 vs. verbs: mean = 47%, SD = 25, p < 0.001) and the percentage of correct responses was greater at the end of training (T5) compared to the baseline (T0) (T5: mean = 66%, SD = 24 vs. T0: mean = 32%, SD = 19, p < 0.001) and in the anodal tsDCS condition compared to the other two conditions (anodal: mean = 57%, SD = 28 vs. cathodal: mean = 51%, SD = 26 vs. sham: mean = 51%, SD = 26, p = 0.005).
The interaction WORD CATEGORY × CONDITION × TIME was also significant [F(4, 52) = 4.66, p = 0.003]. Indeed, while no significant differences between nouns and verbs were found for all experimental conditions at T0 (differences between nouns vs. verbs, anodal = 6%, p = 1; cathodal = 2%, p = 1; sham = 6%, p = 1), verbs significantly improved in all conditions at the end of treatment (T5) with respect to the baseline (T0) (difference between T5 and T0 anodal = 42%, p < 0.001; cathodal = 20%, p < 0.001; sham = 22%, p < 0.001) but the improvement was greater only after the anodal tsDCS condition compared to the other two conditions, which did not differ from each other (anodal vs. cathodal = 18%, p < 0.001; anodal vs. sham = 18%, p < 0.001; cathodal vs. sham = 0%, p = 1). No specific trend was found due to the properties of the verb itself. Indeed, at the end of treatment, the mean number of correct responses produced in the anodal condition did not reveal any significant differences among the different verb categories (hand vs. mouth vs. body actions) [F(2, 26) = 0.02, p = 0.98].
On the contrary, although the percentage of correct responses for nouns significantly improved in all conditions at the end of treatment (T5) with respect to baseline (T0) (difference between T5 and T0 anodal = 44%, p < 0.001; cathodal = 40%, p < 0.001; sham = 39%, p < 0.001), no significant differences between the three conditions were found (anodal vs. cathodal = 4%, p = 1; anodal vs. sham = 3%, p = 1; cathodal vs. sham = −1%, p = 1).
Moreover, the improvement reached for verbs in the anodal condition persisted at 1 week after the treatment (anodal FU-T5 = −3%, p = 1) and it remained greater compared to the other two conditions (anodal vs. cathodal at FU = 14%, p < 0.001; anodal vs. sham at FU = 18%, p < 0.001; cathodal vs. sham at FU = 4%, p = 1) (see Table 2; Figure 3).
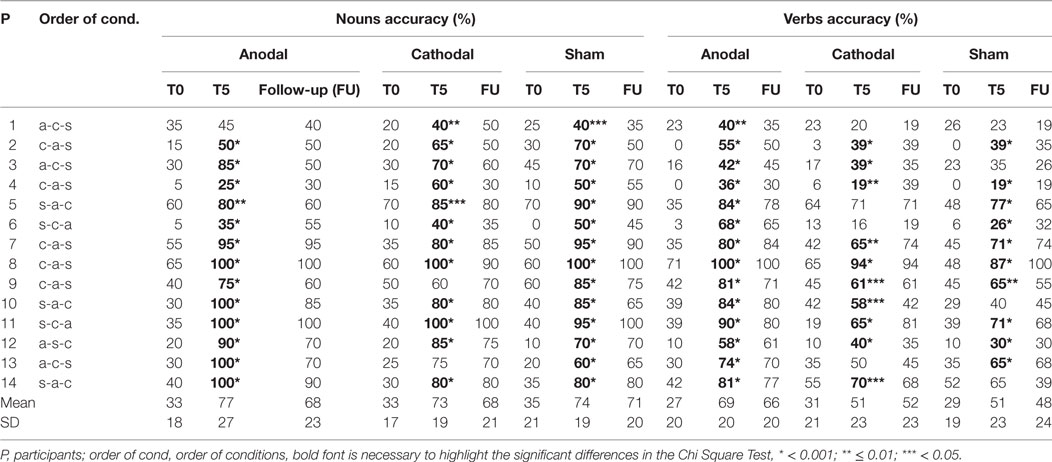
Table 2. Mean percentage of correct responses in noun and verb naming for anodal, cathodal, and sham condition for each patient.
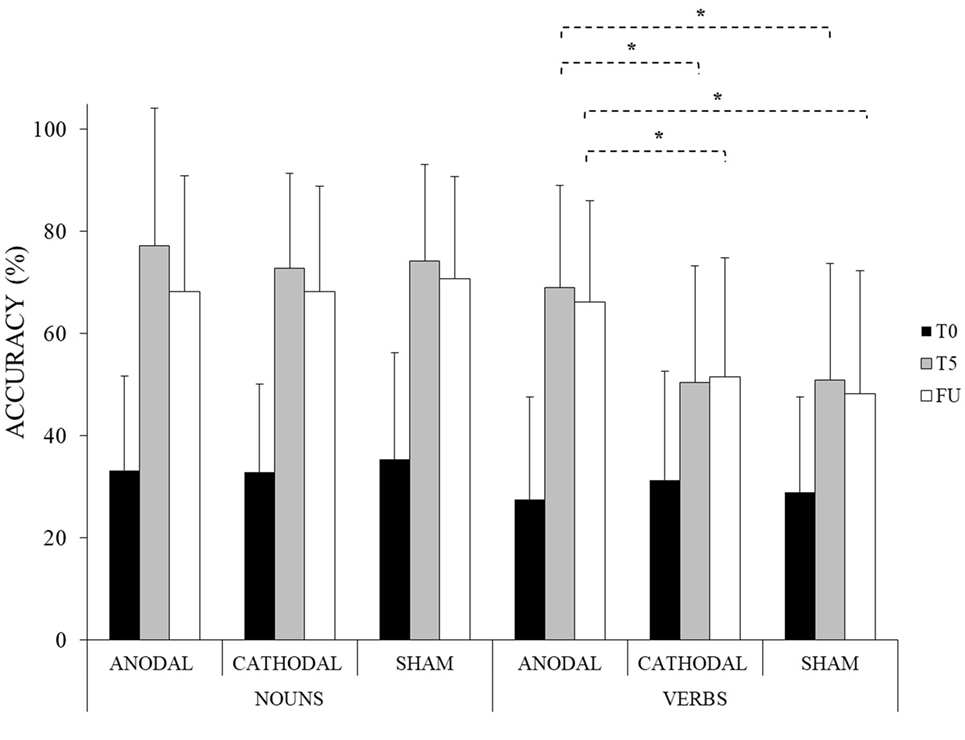
Figure 3. Mean percentage of response accuracy for nouns and verbs at baseline (T0), at the end of the treatment (T5) and at follow-up (FU) for the anodal, cathodal and sham condition, respectively (*<0.001). Error bars represent SD.
We further explored whether the group statistical patterns hold true for all individuals in the study. Thus, for each subject, we measured the amount of improvement at the end of treatment in all conditions (anodal vs. cathodal vs. sham) for the verb and noun category, respectively. For the verb category, the pattern resulted consistent in 11 subjects out of 14 showing a greater improvement in the anodal condition compared to the other two (P1-2-4-5-6-7-9-10-12-13-14). The two remaining subjects showed a greater improvement in the anodal condition compared to sham but not compared to cathodal condition (P3 and 11). Only one subject (P8) showed no significant differences between all conditions. For the noun category, the pattern resulted consistent in 8 subjects out of 14 showing no significant differences between the three conditions (P1-2-5-7-8-11-12-14). Four out of 14 subjects (P3-9-10-13) showed a greater improvement for the anodal condition compared to the sham and/or cathodal condition while, the two remaining subjects showed a greater improvement for the sham and/or cathodal condition compared to the anodal one (P4 and 6) (Chi square tests: p < 0.05 for all significant comparisons). Thus, the case series analysis mostly confirmed the group analysis results showing a behavioral pattern more homogenous and consistent across subjects for the verb than for the noun category.
In order to investigate if tDCS had a different impact on the subject’s response, we classified the errors made by each subject in all experimental conditions. As shown in Table 3, errors were (1) no responses, (2) semantic paraphasias, and (3) unrelated verb responses but, at baseline (T0), for both the noun and the verb naming, errors were predominantly “no responses.” Thus, we conducted an ANOVA on the number of “no responses” with three within-subject factors: TASK (noun naming vs. verb naming), CONDITION (anodal vs. cathodal vs. sham), and TIME [baseline (T0) vs. end of the treatment (T5)]. The analysis revealed a significant interaction TASK × CONDITION × TIME [F(2, 26) = 6.66, p = 0.005]. Indeed, although all experimental conditions led to a lower number of “no responses” at the end of treatment (T5) compared to the baseline (T0) (noun naming: difference between T5 and T0 anodal = 5, p < 0.001; cathodal = 5, p < 0.001; sham = 6, p < 0.001; verb naming: difference between T5 and T0 anodal = 6, p < 0.001; cathodal = 4, p < 0.001; sham = 4, p < 0.001), at the end of treatment (T5), only in the verb naming task the number of “no responses” was lower after anodal stimulation compared to the other two conditions which did not differ from each other (anodal vs. cathodal = −2, p < 0.001; anodal vs. sham = −2, p < 0.001; cathodal vs. sham = 0, p = 1). Thus, these results resembled those previously found for the accuracy data.

Table 3. Mean number of errors in noun and verb naming task at baseline (T0) and at the end of treatment (T5) for each transcutaneous spinal direct current stimulation conditions (±SD).
Vocal Reaction Times
The analysis showed a significant effect of WORD CATEGORY [F(1, 13) = 33.05, p < 0.001] and TIME [F(2, 26) = 48.74, p < 0.001]. Overall, participants were significantly faster to name nouns compared to verbs (nouns: mean = 10,656 ms, SD = 5,327 vs. verbs: mean = 13,106 ms, SD = 4441, p < 0.001) and vocal reaction times were faster at the end of training (T5) compared to baseline (T0) (T5: mean = 10,139 ms, SD = 5,204 vs. T0: mean = 15,132 ms, SD = 2905, p < 0.001).
The interaction WORD CATEGORY × CONDITION × TIME was also significant [F(4, 52) = 3.49, p = 0.01]. Indeed, while no significant differences were found between the two categories for all experimental conditions at T0 (differences between nouns vs. verbs, anodal = −1,104 ms; p = 1; cathodal = −837 ms, p = 1; sham = −1,092 ms, p = 1), vocal reaction times were significantly faster for verbs in all conditions at the end of treatment (T5) with respect to the baseline (T0) (difference between T5 and T0 anodal = −5,195 ms, p < 0.001; cathodal = −2,856 ms, p < 0.001; sham = −3,258 ms, p < 0.001) but the improvement was greater only after the anodal tsDCS condition compared to the other two conditions, which did not differ from each other (anodal vs. cathodal = −2,269 ms, p < 0.001; anodal vs. sham = −2,026 ms, p = 0.001; cathodal vs. sham = 243 ms, p = 1). On the contrary, although vocal reaction times were significantly faster for nouns in all conditions at the end of treatment (T5) with respect to the baseline (T0) (difference between T5 and T0 anodal = −6,218 ms, p < 0.001; cathodal = −5,574 ms, p < 0.001; sham = −6,854 ms, p < 0.001), no significant differences between the three conditions were found (anodal vs. cathodal = −841 ms, p = 1; anodal vs. sham = 535 ms, p = 1; cathodal vs. sham = 1,376 ms, p = 0.23).
Moreover, the improvement reached for verbs in the anodal condition persisted at 1 week after the treatment (anodal FU-T5 = −28 ms, p = 1). Indeed, vocal reaction times were still significantly faster compared to the other two conditions (anodal vs. cathodal at FU = −2,276 ms, p < 0.001; anodal vs. sham at FU = −1,936 ms, p = 0.003; cathodal vs. sham at FU = 304 ms, p = 1) (see Figure 4).
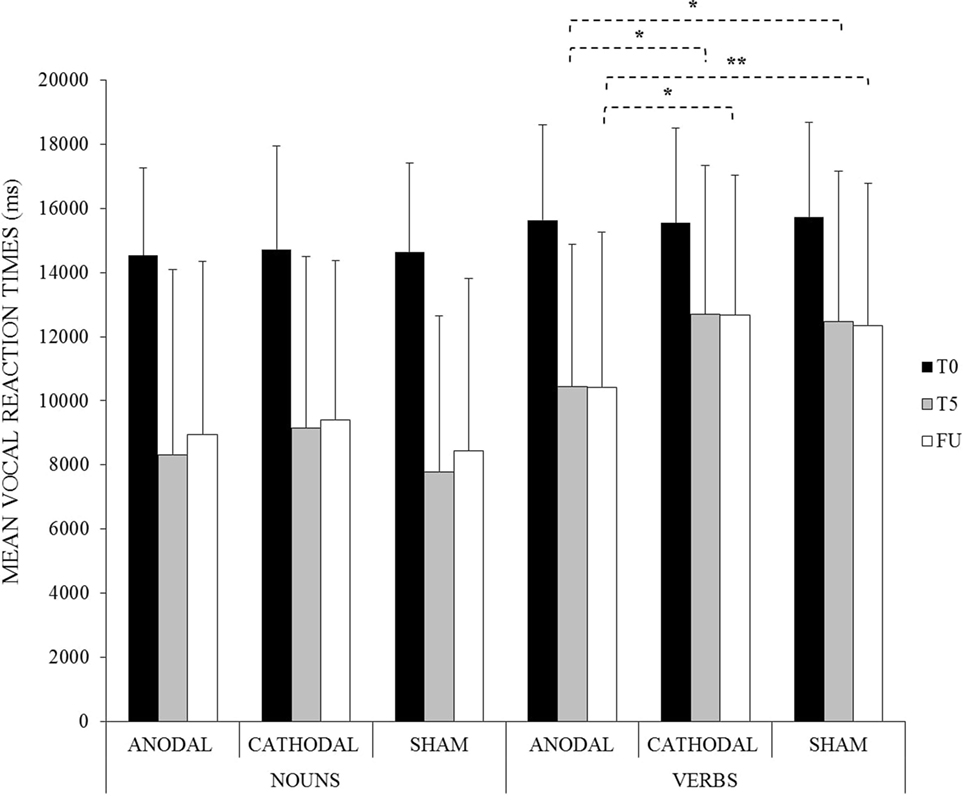
Figure 4. Mean vocal reaction times for noun and verb naming at baseline (T0), at the end of the treatment (T5) and at follow-up (FU) for the anodal, cathodal, and sham condition, respectively (*<0.001, **<0.01). Error bars represent SD.
In order to control for possible influence of age, educational level, and time post-onset variables on the amount of improvement found for verb naming, correlational analyses were also conducted. Both for accuracy and reaction time data, no significant correlations were observed (p > 0.05). No differences between males and females emerged as well (unpaired t-test, p > 0.05).
Further Analysis
It may be argued that the observed results were an artifact of treatment efficacy, which was greater for nouns than for verbs. In this case, in the sham condition, subjects would have enough time to reach the same amount of improvement found in the anodal condition, resulting in no significant differences between the two conditions for the noun category. Thus, we reasoned that if this would be the case, we should have found different treatment effects across sessions (T0, T1, T2, T3, T4, T5) in the sham condition for nouns and verbs, respectively. However, statistical analyses revealed no significant differences (mean percentage of improvement for nouns: T1–T0 = 9%, T2–T1 = 9%, T3–T2 = 8%, T4–T3 = 6%, T5–T4 = 6% mean percentage of improvement for verbs: T1–T0 = 7%, T2–T1 = 6%, T3–T2 = 5%, T4–T3 = 1%, T5–T4 = 4%, t-tests, p > 0.05).
Discussion
This study assessed whether tsDCS coupled with language treatment improves word retrieval in persons with chronic non-fluent aphasia. Our findings showed that anodal tsDCS differently affected the amount of improvement in noun and verb naming. Indeed, while noun and verb naming significantly improved in all patients for each condition at the end of training due to language treatment, anodal tsDCS boosted recovery only for verbs. Moreover, FU testing showed that these effects lasted over 1 week after the intervention. This specificity argues against an effect simply due to enhanced cognitive arousal which should have influenced both verb and noun naming.
Although this finding seems surprising, it suggests that the spinal cord takes part in verb processing acting as a “bridge” for conveying tsDCS induced changes into brain networks. Thereby inducing neuromodulation effects into brain areas involved in verb naming.
As previously stated, opposite excitability changes induced by cortical tDCS (60, 61) and spinal tDCS (42, 44) of the same polarity have been reported. Indeed, while anodal tDCS is generally facilitatory to the cortex (30, 39, 47), anodal tsDCS was found to decrease the amplitude of the somatosensory evoked potentials (42), laser evoked potentials (44) and motor responses evoked by transcranial magnetic stimulation (46) due to an hyperpolarization of the axons running along the spinal columns, while cathodal stimulation did not exert any influence (42, 46). It has also been shown that thoracic tsDCS elicits intracortical changes through the thalamus and the reticular systems (46, 49). Indeed, recent modeling studies have proved that, despite some inter-individual differences, the electric field induced by thoracic tsDCS is longitudinally directed along the vertebral column, especially when the return electrode is placed over the right arm (62, 63). Yet, the electric field induced by thoracic tsDCS is maximum at thoracic level and it can increase the somatosensory activity from the spinal cord to the brain (62, 63).
Even if the exact underlying tsDCS mechanisms over the corticospinal system, in our study, remain largely speculative, the hypothesis might be advanced that anodal tsDCS has inhibited the tonic ascending system to the cortex, ultimately decreasing the activity into the sensorimotor areas. Paradoxically, the resulting reduction could have potentiated their function. Indeed, the hypothesis has been advanced that inhibitory current might decrease the excitability of cortical inhibitory interneurons (64, 65), thus improving the efficacy of their related areas. Following previous suggestion, we might also hypothesize that anodal tsDCS has increased the interhemispheric delay in motor connectivity (49), thus, enhancing the functionality of the left sensorimotor cortices through inhibition of its right homologs (66). Indeed, the model of interhemispheric competition between the residual language areas in the left-damaged hemisphere and the intact right hemisphere (akin to models of motor recovery after stroke) proposes that in patients with left hemispheric damage, the homotopic contralateral right hemispheric areas may be in a state of abnormally high activation and may exert an inhibitory effect over the damaged hemisphere (67, 68). Thus, improvement may be possible either by increasing the output of the perilesional left hemisphere through excitatory stimulation (59, 69–71) or decreasing the inhibition from the intact right hemisphere by applying inhibitory current over the contralesional cortex (72–75). Therefore, in our study, we might hypothesize that tsDCS has inhibited the right hemisphere areas increasing the left hemisphere activity and, in particular, the left sensorimotor areas, making them more efficient. Indeed, all patients had preserved motor cortex and the amount of improvement obtained for verbs in the anodal condition was similar across patients with a partial damage over the somatosensory areas (see Table 1) and the rest of the group [five damaged subjects: mean = 49% (SD = 12) p < 0.001 vs. nine undamaged subjects: mean = 38% (SD = 11) p < 0.001; difference between the two groups: mean = 11%, unpaired t-test p = 0.14].
An effect of tsDCS on pharmacologically defined systems cannot also be ruled out. Indeed, it has been shown that the mechanisms of action underlying tDCS could also involve receptors and neurotransmitters. For instance, neurotransmitters such as GABA and glutamate undergo substantial changes into the brain after cortical tDCS over the motor cortex (47, 76). Accordingly, Stagg et al. (76) found that excitatory (anodal) tDCS reduced GABA levels within the sensorimotor cortices while inhibitory (cathodal) stimulation reduced glutamatergic neuronal activity with a highly correlated reduction in GABA over the same region, due to the close biochemical relationship between the two neurotransmitters. A local decrease of GABA levels in the sensorimotor cortex is strongly related with synaptic plasticity and it is positively correlated with an improvement in motor learning (77–79). Although this effect might be task specific, one further hypothesis to consider is that, in our study, the inhibitory current delivered through anodal tsDCS has decreased both glutamate and GABA levels into the sensorimotor cortices leading to an improvement of their function (76, 78, 79).
All of the above mentioned hypotheses explain the specificity of anodal tDCS for verbs. As stated in the Introduction, several lines of evidence have already shown that action words are partly represented into the sensorimotor cortex due to their sensorimotor semantic properties which, in turn, are involved in action understanding and naming (4, 10–13, 15–18, 41). This implies that the sensorimotor cortex may also process action concepts (6). Yet, Repetto et al. (80) found that processing hand-related action words, but not abstract words, was impaired after repetitive TMS to the left primary motor cortex [see also Ref. (81)]. Similarly, very recently, Meinzer et al. (82) have shown that anodal tDCS delivered over the left motor cortex with concomitant language training improved naming abilities in a group of 26 patients with chronic aphasia.
Thus, we hypothesize that anodal tDCS has influenced neural activity along the ascending spinal pathways, ultimately modulating activity in the sensory–motor cortex. This has, in turn, facilitated verb retrieval. Indeed, the improvement found in verb naming was not specific for any type of action. Yet, the current delivered over the thoracic vertebrae going up to the brain also resulted in an improvement on verbs (i.e., hand and mouth actions) whose corresponding muscles are innervated above the stimulated region. This last result further confirms our hypothesis that action verbs were ultimately processed by the sensorimotor areas. On the contrary, since nouns do not express a motor content they did not benefit from stimulation.
In conclusion, although other studies will further elucidate our understanding on the role of the spinal cord in language processing, we believe that our results are promising since, for the first time, they suggest that spinal tDCS significantly affects the sensorimotor system removing the need to establish which part of this system should be targeted with tDCS. Since verbs play a crucial role in sentence construction which is essential to enhance speech production in persons with aphasia, we believe that this finding is important for treatment outcomes.
Ethics Statement
This study was carried out in accordance with the recommendations of the Helsinki Declaration and the Institutional Review Board of the IRCCS Fondazione Santa Lucia, Rome, Italy with written informed consent from all subjects. All subjects gave written informed consent in accordance with the Declaration of Helsinki. The protocol was approved by the Institutional Review Board of the IRCCS Fondazione Santa Lucia, Rome, Italy.
Author Contributions
Conceived and designed the experiment: PM, VF, and AP. Performed the experiment: VF and JS. Analyzed the data: VF, TG, and GC. Wrote the paper: PM. Edited the manuscript: PM, CC, and AP.
Conflict of Interest Statement
The authors declare that the research was conducted in the absence of any commercial or financial relationships that could be construed as a potential conflict of interest.
References
1. Wernicke C. The Symptom Complex of Aphasia: A Psychological Study on an Anatomical Basis. Boston Studies in the Philosophy of Science. Dordrecht: D. Reidel Publishing Company (1874/1969). 34 p.
2. Crosson B. Thalamic mechanisms in language: a reconsideration based on recent findings and concepts. Brain Lang (2013) 126:73–88. doi:10.1016/j.bandl.2012.06.011
3. Price CJ. The anatomy of language: a review of 100 fMRI studies published in 2009. Ann N Y Acad Sci (2010) 1191:62–88. doi:10.1111/j.1749-6632.2010.05444.x
4. Willems RM, Hagoort P. Neural evidence for the interplay between language, gesture, and action: a review. Brain Lang (2007) 101:278–89. doi:10.1016/j.bandl.2007.03.004
5. Binkofski F, Buccino G. The role of ventral premotor cortex in action execution and action understanding. J Physiol Paris (2006) 99:396–405. doi:10.1016/j.jphysparis.2006.03.005
6. Gili T, Fiori V, De Pasquale G, Sabatini U, Caltagirone C, Marangolo P. Right sensory-motor functional networks subserve action observation therapy in aphasia. Brain Imaging Behav (2016):1–15. doi:10.1007/s11682-016-9635-1
7. Barsalou LW. Perceptual symbol systems. Behav Brain Sci (1999) 22:560–77. doi:10.1017/S0140525X99002149
8. Gallese V, Lakoff G. The brain’s concepts: the role of the sensorimotor system in conceptual knowledge. Cogn Neuropsychol (2005) 21:455–79. doi:10.1080/02643290442000310
9. Rizzolatti G, Craighero L. The mirror neuron system. Annu Rev Neurosci (2004) 27:169–92. doi:10.1146/annurev.neuro.27.070203.144230
10. Marangolo P, Bonifazi S, Tomaiuolo F, Craighero L, Coccia M, Altoè G, et al. Improving language without words: first evidence from aphasia. Neuropsychologia (2010) 48:3824–33. doi:10.1016/j.neuropsychologia.2010.09.025
11. Marino BF, Sirianni M, Volta RD, Magliocco F, Silipo F, Quattrone A, et al. Viewing photos and reading nouns of natural graspable objects similarly modulate motor responses. Front Hum Neurosci (2014) 8:968. doi:10.3389/fnhum.2014.00968
12. Marino BF, Gallese V, Buccino G, Riggio L. Language sensorimotor specificity modulates the motor system. Cortex (2012) 48:849–56. doi:10.1016/j.cortex.2010.12.003
13. Pulvermüller F, Shtyrov Y, Ilmoniemi R. Brain signatures of meaning access in action word recognition. J Cogn Neurosci (2005) 17:884–92. doi:10.1162/0898929054021111
14. Rizzolatti G, Fabbri-Destro M, Cattaneo L. Mirror neurons and their clinical relevance. Nat Clin Pract Neurol (2009) 5:24–34. doi:10.1038/ncpneuro0990
15. Hauk O, Pulvermuller F. Neurophysiological distinction of action words in the fronto-central cortex. Hum Brain Mapp (2004) 21:191–201. doi:10.1002/hbm.10157
16. Tettamanti M, Buccino G, Saccuman MC, Gallese V, Danna M, Scifo P, et al. Listening to action-related sentences activates fronto-parietal motor circuits. J Cogn Neurosci (2005) 17:273–81. doi:10.1162/0898929053124965
17. Fischer MH, Zwaan RA. Embodied language: a review of the role of the motor system in language comprehension. Q J Exp Psychol (Hove) (2008) 61:825–50. doi:10.1080/17470210701623605
18. Sato M, Mangarelle M, Riggio L, Gallese V, Bucciino G. Task related modulation of the motor system during language processing. Brain Lang (2008) 105:83–90. doi:10.1016/j.bandl.2007.10.001
19. Wolpaw JR, Tennissen AM. Activity-dependent spinal cord plasticity in health and disease. Annu Rev Neurosci (2001) 24:807–43. doi:10.1146/annurev.neuro.24.1.807
20. Bregman BS, McAtee M, Dai HN, Kuhn PL. Neurotrophic factors increase axonal growth after spinal cord injury and transplantation in the adult rat. Exp Neurol (1997) 148:475–94. doi:10.1006/exnr.1997.6705
21. Tuszynski M, Edgerton R, Dobkin B. Recovery of locomotion after experimental spinal cord injury: axonal regeneration or modulation of intrinsic spinal cord walking circuitry? J Spinal Cord Med (1999) 22:143–143. doi:10.1080/10790268.1999.11719563
22. Kreutzer JS, Barth J, Ellwood MS, Gideon D, Stutts M, Wegner ST, et al. Occult neuropsychological impairments in spinal cord injured patients. Arch Phys Med Rehabil (1988) 69:764–5.
23. Roth E, Davidoff G, Thomas P, Doljanac R, Dijkers M, Berent S, et al. A controlled study of neuropsychological deficits in acute spinal cord injury patients. Spinal Cord (1989) 27(6):480–9. doi:10.1038/sc.1989.75
24. Davidoff GN, Roth EJ, Richards JS. Cognitive deficits in spinal cord injury: epidemiology and outcome. Arch Phys Med Rehabil (1992) 73(3):275–84.
25. Murray RF, Asghari A, Egorov DD, Rutkowski SB, Siddall PJ, Soden RJ, et al. Impact of spinal cord injury on self-perceived pre- and postmorbid cognitive, emotional and physical functioning. Spinal Cord (2007) 45:429–36. doi:10.1038/sj.sc.3102022
26. Davidoff G, Morris J, Roth E, Bleiberg J. Cognitive dysfunction and mild closed head injury in traumatic spinal cord injury. Arch Phys Med Rehabil (1985) 66:489–91.
27. Colachis SC, Fugate LP. Autonomic dysreflexia associated with transient aphasia. Spinal Cord (2002) 40(3):142–4. doi:10.1038/sj.sc.3101251
28. Galletta EE, Cancelli A, Cottone C, Simonelli I, Tecchio F, Bikson M, et al. Use of computational modeling to inform tDCS electrode montages for the promotion of language recovery in post-stroke aphasia. Brain Stimul (2015) 8:1108–15. doi:10.1016/j.brs.2015.06.018
29. Marangolo P, Fiori V, Sabatini U, De Pasquale G, Razzano C, Caltagirone C, et al. Bilateral transcranial direct current stimulation language treatment enhances functional connectivity in the left hemisphere: preliminary data from aphasia. J Cogn Neurosci (2016) 28:724–38. doi:10.1162/jocn_a_00927
30. Di Lazzaro V, Ranieri F, Profice P, Pilato F, Mazzone P, Capone F, et al. Transcranial direct current stimulation effects on the excitability of corticospinal axons of the human cerebral cortex. Brain Stimul (2013) 6:641–3. doi:10.1016/j.brs.2012.09.006
31. Ngernyam N, Jensen MP, Arayawichanon P, Auvichayapat N, Tiamkao S, Janjarasjitt S, et al. The effects of transcranial direct current stimulation in patients with neuropathic pain from spinal cord injury. Clin Neurophysiol (2015) 126:382–90. doi:10.1016/j.clinph.2014.05.034
32. Yamaguchi T, Fujiwara T, Tsai YA, Tang SC, Kawakami M, Mizuno K, et al. The effects of anodal transcranial direct current stimulation and patterned electrical stimulation on spinal inhibitory interneurons and motor function in patients with spinal cord injury. Exp Brain Res (2016) 234:1469–78. doi:10.1007/s00221-016-4561-4
33. Priori A, Hallett M, Rothwell JC. Repetitive transcranial magnetic stimulation or transcranial direct current stimulation? Brain Stimul (2009) 2:241–5. doi:10.1016/j.brs.2009.02.004
34. Nitsche MA, Paulus W. Excitability changes induced in the human motor cortex by weak transcranial direct current stimulation. J Physiol (2000) 527:633–9. doi:10.1111/j.1469-7793.2000.t01-1-00633.x
35. Nitsche MA, Paulus W. Sustained excitability elevations induced by transcranial DC motor cortex stimulation in humans. Neurology (2001) 57:1899–901. doi:10.1212/WNL.57.10.1899
36. Lang N, Siebner HR, Ward NS, Lee L, Nitsche MA, Paulus W, et al. How does transcranial DC stimulation of the primary motor cortex alter regional neuronal activity in the human brain? Eur J Neurosci (2005) 22:495–504. doi:10.1111/j.1460-9568.2005.04233.x
37. Roche N, Lackmy A, Achache V, Bussel B, Katz R. Impact of transcranial direct current stimulation on spinal network excitability in humans. J Physiol (2009) 587:5653–64. doi:10.1113/jphysiol.2009.177550
38. Roche N, Lackmy A, Achache V, Bussel B, Katz R. Effects of anodal transcranial direct current stimulation over the leg motor area on lumbar spinal network excitability in healthy subjects. J Physiol (2011) 589:2813–26. doi:10.1113/jphysiol.2011.205161
39. Di Lazzaro V, Profice P, Ranieri F, Capone F, Dileone M, Oliviero A, et al. I-wave origin and modulation. Brain Stimul (2012) 5:512e25. doi:10.1016/j.brs.2011.07.008
40. Haavik H, Niazi IK, Jochumsen M, Sherwin D, Flavel S, Türker KS. Impact of spinal manipulation on cortical drive to upper and lower limb muscles. Brain Sci (2016) 7:2. doi:10.3390/brainsci7010002
41. Buccino G, Riggio L, Melli G, Binkofski F, Gallese V, Rizzollatti G. Listening to action related sentences modulates the activity of the motor system: a combined TMS and behavioral study. Brain Res Cogn Brain Res (2005) 24:355–63. doi:10.1016/j.cogbrainres.2005.02.020
42. Cogiamanian F, Vergari M, Pulecchi F, Merceglia S, Priori A. Effect of spinal transcutaneous direct current stimulation on somatosensory evoked potentials in humans. Clin Neurophysiol (2008) 119:2636–40. doi:10.1016/j.clinph.2008.07.249
43. Cogiamanian F, Vergari M, Schiaffi E, Merceglia S, Ardolino G, Barbieri S, et al. Transcutaneous spinal cord direct current stimulation inhibits the lower limb nociceptive flexion reflex in human beings. Pain (2011) 152:370–5. doi:10.1016/j.pain.2010.10.041
44. Truini A, Vergari M, Biasiotta A, La Cesa S, Gabriele M, Di Stefano G, et al. Transcutaneous spinal direct current stimulation inhibits nociceptive spinal pathway conduction and increases pain tolerance in humans. Eur J Pain (2011) 15:1023–7. doi:10.1016/j.ejpain.2011.04.009
45. Priori A, Ciocca M, Parazzini M, Vergari M, Ferrucci R. Transcranial cerebellar direct current stimulation and transcutaneous spinal cord direct current stimulation as innovative tools for neuroscientists. J Physiol (2014) 592:3345–69. doi:10.1113/jphysiol.2013.270280
46. Bocci T, Barloscio D, Vergari M, Di Rollo A, Rossi S, Priori A, et al. Spinal direct current stimulation modulates short intracortical inhibition. Neuromodulation (2015) 18:686–93. doi:10.1111/ner.12298
47. Nitsche M, Seeber A, Frommann K, Klein CC, Rochford C, Nitsche MS, et al. Modulating parameters of excitability during and after transcranial direct current stimulation of the human motor cortex. J Physiol (2005) 568:291–303. doi:10.1113/jphysiol.2005.092429
48. Bocci T, Marceglia S, Vergari M, Cognetto V, Cogiamanian F, Sartucci F, et al. Transcutaneous spinal direct current stimulation modulates human corticospinal system excitability. J Neurophysiol (2015) 114:440–6. doi:10.1152/jn.00490.2014
49. Bocci T, Caleo M, Vannini B, Vergari M, Cogiamanian F, Rossi S, et al. An unexpected target of spinal direct current stimulation: interhemispheric connectivity in humans. J Neurosci Methods (2015) 254:18–26. doi:10.1016/j.jneumeth.2015.07.012
50. De Renzi E, Vignolo LA. The Token Test: a sensitive test to detect receptive disturbances in aphasia. Brain (1962) 85:665–78. doi:10.1093/brain/85.4.665
51. Miceli G, Laudanna A, Burani C, Capasso R. Batteria per l’analisi dei deficit afasici BADA. Roma: CEPSAG, Policlinico Gemelli, Università Cattolica del Sacro Cuore (1994).
52. Spinnler H, Tognoni G. Taratura e Standardizzazione Italiana di Test Neuropsicologici. Ital J Neurol Sci (1987) 6(Suppl 8):12–120.
54. De Mauro T, Mancini F, Vedovelli M, Voghera M. Lessico di frequenza dell’italiano parlato. Milano: Etaslibri (1993).
55. Lotto L, Dell’Acqua R, Job R. Le figure PD/DPSS. Misure di accordo sul nome, tipicità, familiarità, età di acquisizione e tempi di denominazione per 266 figure. Giornale Italiano di Psicologia (2001) 1:193–210.
56. Furubayashi T, Terao Y, Arai N, Okabe S, Mochizuki H, Hanajima R, et al. Short and long duration transcranial direct current stimulation (tDCS) over the human hand motor area. Exp Brain Res (2008) 185:279–86. doi:10.1007/s00221-007-1149-z
57. Kidgell DJ, Goodwill AM, Frazer AK, Robin MD. Induction of cortical plasticity and improved motor performance following unilateral and bilateral transcranial direct current stimulation of the primary motor cortex. BMC Neurosci (2013) 14:64. doi:10.1186/1471-2202-14-64
58. Fricke K, Seeber AA, Thirugnanasambandam N, Paulus W, Nitsche MA, Rothwell JC. Time course of the induction of homeostatic plasticity generated by repeated transcranial direct current stimulation of the human motor cortex. J Neurophysiol (2011) 105:1141–9. doi:10.1152/jn.00608.2009
59. Fiori V, Cipollari S, Di Paola M, Razzano C, Caltagirone C, Marangolo P. tDCS stimulation segregates words in the brain: evidence from aphasia. Front Hum Neurosci (2013) 7:269. doi:10.3389/fnhum.2013.00269
60. Liebetanz D, Nitsche MA, Tergau F, Paulus W. Pharmacological approach to the mechanisms of transcranial DC-stimulation-induced aftereffects of human motor cortex excitability. Brain (2002) 125:2238–47. doi:10.1093/brain/awf238
61. Nitsche MA, Liebetanz D, Antal A, Lang N, Tergau F, Paulus W. Modulation of cortical excitability by weak direct current stimulation – technical, safety and functional aspects. Suppl Clin Neurophysiol (2003) 56:255–76. doi:10.1016/S1567-424X(09)70230-2
62. Fiocchi S, Ravazzani P, Priori A, Parazzini M. Cerebellar and spinal direct current stimulation in children: computational modeling of the induced electric field. Front Hum Neurosci (2016) 10:522. doi:10.3389/fnhum.2016.00522
63. Parazzini M, Fiocchi S, Liorni I, Rossi E, Cogiamanian F, Vergari M, et al. Modeling the current density generated by transcutaneous spinal direct current stimulation (tsDCS). Clin Neurophysiol (2014) 125:2260–70. doi:10.1016/j.clinph.2014.02.027
64. Lang N, Nitsche MA, Paulus W, Rothwell JC, Lemon R. Effects of transcranial DC stimulation over the human motor cortex on corticospinal and transcallosal excitability. Exp Brain Res (2004) 156:439–43. doi:10.1007/s00221-003-1800-2
65. Monti A, Cogiamanian F, Marceglia S, Ferrucci R, Mameli F, Mrakic-Sposta S, et al. Improved naming after transcranial direct current stimulation in aphasia. J Neurol Neurosurg Psychiatry (2008) 79:451–3. doi:10.1136/jnnp.2007.142356
66. Ward NS, Cohen LG. Mechanisms underlying recovery of motor function after stroke. Arch Neurol (2004) 61:1844–8. doi:10.1001/archneur.61.12.1844
67. Belin P, Van Eeckhout P, Zilbovicius M, Remy P, Francois C, Guillaume S, et al. Recovery from nonfluent aphasia after melodic intonation therapy: a PET study. Neurology (1996) 47:1504–11. doi:10.1212/WNL.47.6.1504
68. Murase N, Duque J, Mazzocchio R, Cohen LG. Influence of interhemispheric interactions on motor function in chronic stroke. Ann Neurol (2004) 55:400–9. doi:10.1002/ana.10848
69. Baker JM, Rorden C, Fridriksson J. Using transcranial direct-current stimulation to treat stroke patients with aphasia. Stroke (2010) 41:1229–36. doi:10.1161/STROKEAHA.109.576785
70. Fiori V, Coccia M, Marinelli CV, Vecchi V, Bonifazi S, Ceravolo MG. Transcranial direct current stimulation (tDCS) improves word retrieval in healthy and nonfluent aphasic subjects. J Cogn Neurosci (2011) 23:2309–23. doi:10.1162/jocn.2010.21579
71. Marangolo P, Fiori V, Di Paola M, Cipollari S, Razzano C, Oliveri M, et al. Differential processing of the left frontal and temporal regions in verb naming: a tDCS treatment study. Restor Neurol Neurosci (2013) 31(1):63–72. doi:10.3233/RNN-120268
72. Kang EK, Kim YK, Sohn HM, Cohen LG, Paik NJ. Improved picture naming in aphasia patients treated with cathodal tDCS to inhibit the right Broca’s homologue area. Restor Neurol Neurosci (2011) 29:141–52. doi:10.3233/RNN-2011-0587
73. Jung IY, Lim JY, Kang EK, Sohn HM, Paik NJ. The factors associated with good responses to speech therapy combined with transcranial direct current stimulation in post-stroke aphasic patients. Ann Rehabil Med (2011) 35:460–9. doi:10.5535/arm.2011.35.4.460
74. Naeser MA, Martin PI, Nicholas M, Baker EH, Seekins H, Kobayashi M, et al. Improved picture naming in chronic aphasia after TMS to part of right Broca’s area: an open-protocol study. Brain Lang (2005) 93:95–105. doi:10.1016/j.bandl.2004.08.004
75. Naeser MA, Martin PI, Nicholas M, Baker EH, Seekins H, Helm-Estabrooks N, et al. Improved naming after TMS treatments in a chronic, global aphasia patient: a case report. Neurocase (2005) 1:182–93. doi:10.1080/13554790590944663
76. Stagg CJ, Best JG, Stephenson MC, O’Shea J, Wylezinska M, Kincses ZT, et al. Polarity-sensitive modulation of cortical neurotransmitters by transcranial stimulation. J Neurosci (2009) 29:5202–6. doi:10.1523/JNEUROSCI.4432-08.2009
77. Stagg CJ, Nitsche MA. Physiological basis of transcranial direct current stimulation. Neuroscientist (2011) 17:37–53. doi:10.1177/1073858410386614
78. Stagg CJ, Bachtiar V, Johansen-Berg H. The role of GABA in humanmotor learning. Curr Biol (2011) 21:480–4. doi:10.1016/j.cub.2011.01.069
79. Kim S, Stephenson MC, Morris PG, Jackson SR. tDCS-induced alterations in GABA concentration within primary motor cortex predict motor learning and motor memory: a 7 T magnetic resonance spectroscopy study. Neuroimage (2014) 99:237–43. doi:10.1016/j.neuroimage.2014.05.070
80. Repetto C, Colombo B, Cipresso P, Riva G. The effects of rTMS over the primary motor cortex: the link between action and language. Neuropsychologia (2013) 51:8–13. doi:10.1016/j.neuropsychologia.2012.11.001
81. Liuzza MT, Candidi M, Aglioti SM. Do not resonate with actions: sentence polarity modulates cortico-spinal excitability during action-related sentence reading. PLoS One (2011) 6:e16855. doi:10.1371/journal.pone.0016855
Keywords: transcutaneous spinal direct current stimulation, spinal cord, neurostimulation, aphasia, stroke, verb recovery
Citation: Marangolo P, Fiori V, Shofany J, Gili T, Caltagirone C, Cucuzza G and Priori A (2017) Moving Beyond the Brain: Transcutaneous Spinal Direct Current Stimulation in Post-Stroke Aphasia. Front. Neurol. 8:400. doi: 10.3389/fneur.2017.00400
Received: 15 March 2017; Accepted: 25 July 2017;
Published: 08 August 2017
Edited by:
Pavel Lindberg, Université Paris Descartes, FranceReviewed by:
Holly Robson, University of Reading, United KingdomRoy H. Hamilton, University of Pennsylvania, United States
Copyright: © 2017 Marangolo, Fiori, Shofany, Gili, Caltagirone, Cucuzza and Priori. This is an open-access article distributed under the terms of the Creative Commons Attribution License (CC BY). The use, distribution or reproduction in other forums is permitted, provided the original author(s) or licensor are credited and that the original publication in this journal is cited, in accordance with accepted academic practice. No use, distribution or reproduction is permitted which does not comply with these terms.
*Correspondence: Paola Marangolo, paola.marangolo@gmail.com