- 1Department of Clinical Laboratory, The First Affiliated Hospital of Zhengzhou University, Zhengzhou, China
- 2Department of Clinical Laboratory, Children's Hospital of Zhengzhou, Zhengzhou, China
- 3Center for Gene Diagnosis, Zhongnan Hospital of Wuhan University, Wuhan, China
- 4Center of Clinical Laboratory, Wuhan Asia Heart Hospital, Wuhan, China
Inhibition of poly(ADP-ribose) polymerase (PARP) may protect against coronary artery disease (CAD) in animal models, and rs1136410, a non-synonymous single nucleotide polymorphism (SNP) in PARP-1, has a potential impact on PARP activities in vitro. This two-stage case-control study, involving 2803 CAD patients and 2840 controls, aimed to investigate the associations of PARP-1 rs1136410 with CAD development, lipid levels, PARP activities, 8-hydroxy-2′-dexyguanosine (8-OHdG), and interleukin (IL)-6 levels in a Chinese Han population. Assuming a recessive model, the variant genotype GG of SNP rs1136410 showed a significantly inverse association with CAD risk (adjusted odds ratio (OR) = 0.73, P < 0.001), left main coronary artery (LMCA) lesions (P = 0.003), vessel scores (P = 0.003), and modified Gensini scores (P < 0.001). There were significant correlations of SNP rs1136410 with higher levels of total cholesterol (TC) and lower levels of high-density lipoprotein cholesterol (HDL-c). In gene-environment interaction analyses, participants with the variant genotype GG, but without smoking habit, type 2 diabetes mellitus, and hyperlipidemia, conferred an 84% (P < 0.001) decreased risk of CAD. The genotype-phenotype correlation analyses further supported the functional roles of SNP rs1136410 in decreasing PARP activities and 8-OHdG levels. Taken together, our data suggest that SNP rs1136410 may confer protection against CAD through modulation of PARP activities and gene-environment interactions in a Chinese Han population.
Introduction
Coronary artery disease (CAD), the major contributor to death and disability worldwide (Mozaffarian et al., 2016), is a multifactorial disease associated with predisposing genes, metabolic risk factors, and their interactions (Lanktree and Hegele, 2009). Atherosclerosis, the main pathogenesis of CAD, is an oxidative inflammatory process leading to cumulative deposition of lipoproteins, focal intimal thickening, and ultimately myocardial infarction (MI) (He and Zuo, 2015). Oxidative stress, induced by extensive generation of reactive oxygen species (ROS), is a fundamental step of atherosclerosis (Harrison et al., 2003), which triggers multiple DNA lesions of coronary endothelial cells, including modified bases, single- and double- strand breaks (SSB and DSB), and chromosomal aberrations (Shah and Mahmoudi, 2015). In response to these DNA lesions, poly(ADP-ribose) polymerase 1 (PARP-1), a DNA repair sensor, is activated to initiate the base excision repair (BER) pathway by modifying PARP-1 itself and recruiting downstream BER enzymes (El-Khamisy et al., 2003; Altmeyer et al., 2009). However, hyperactivation of PARP-1 by uncontrolled DNA damage consumes excessive NAD+ and prevents ATP production, thus causing a cellular energy crisis and cell death (Berger et al., 1983; Xu et al., 2014).
A growing body of evidence pinpointed the critical roles of PARP-1 in the development of CAD. First, in clinical studies, PARP-1 overexpression was observed in human atherosclerotic plaques (Martinet et al., 2002), and increased the circulating levels of proinflammatory cytokines in patients with unstable angina (Huang et al., 2008). Second, catalytic inhibition of PARP selectively promoted sensitization of foam cells to oxidative damage while protecting against oxidant-induced cell death in endothelial and smooth muscle cell (SMC) lines (Hans et al., 2008). Finally, PARP-1 deletion in mice could ameliorate lipid profiles and endothelium-dependent relaxation (Hans et al., 2009a), reduce expression of proinflammatory factors (von Lukowicz et al., 2008), and consequently block atherosclerotic plaque regression (von Lukowicz et al., 2008; Hans et al., 2009a).
The PARP-1 gene, located on chromosome 1q41-42, has a well-characterized common single nucleotide polymorphism (SNP), Val762Ala (rs1136410), which is a non-synonymous A-to-G mutation at codon 762, resulting in the conversion of valine to alanine in the catalytic domain of PARP-1 (Yu et al., 2012). In vitro, SNP rs1136410 has been reported to exert a dose-dependent impact on kinetics and poly (ADP-ribosyl)ation of PARP (Wang et al., 2007; Beneke et al., 2010).
Taken together, we speculated that SNP rs1136410 might influence PARP activities, and further modulate oxidative DNA damage and CAD risk. Hence, we performed this two-stage case-control study to test whether SNP rs1136410 was associated with CAD risk, CAD severity, lipid levels, 8-hydroxy-2′-dexyguanosine (8-OHdG), interleukin (IL)-6, and PARP activities in a Chinese Han population, followed by multifactor dimensionality reduction (MDR) and classification and regression tree (CART) analyses to identify the high-order gene-environment interactions between SNP rs1136410 and traditional CAD risk factors.
Materials and Methods
Study Subjects
This two-stage case-control study, involving 2803 CAD patients and 2840 age- and sex-matched controls, contained two data sets: the discovery set including 1266 cases and 1296 controls from Wuhan Asia Heart Hospital between March 2011 and February 2017, and the replication set with 1537 cases and 1544 controls from Zhongnan Hospital of Wuhan University and The First Affiliated Hospital of Zhengzhou University (between July 2013 and August 2017). The diagnosis of CAD was based on ≥50% of lumen obstruction in at least one major coronary artery or their main branches by coronary angiography. CAD severity was evaluated by the presence of left main coronary artery (LMCA) lesions, vessel scores, and modified Gensini scores (Montorsi et al., 2006; Weintraub et al., 2011; Bing et al., 2015; Wang et al., 2016; Supplementary Materials and Methods). CAD patients were divided into three groups according to the following clinical presentation: (1) stable angina pectoris (SAP); (2) unstable angina pectoris (UAP); (3) MI (Wang et al., 2016; Supplementary Materials and Methods). The controls were subjects without atherosclerotic lesions and history of CAD, as confirmed by angiography, electrocardiographic test, and physical examination. We collected the following demographics for each participant: (1) traditional CAD risk factors including smoking habit, alcohol drinking habit, and histories of hyperlipidemia, type 2 diabetes mellitus (T2DM), and hypertension; (2) clinical data such as lipid levels, fasting plasma glucose (FPG) levels, blood pressure, and body mass index (BMI) (Supplementary Materials and Methods). We excluded subjects with the following diseases: (1) cardiac diseases such as valvular or congenital heart diseases, myocardial bridge, and coronary spasm; (2) systemic diseases including cancers, hepatic or renal diseases, and autoimmune diseases. This study complied with the Declaration of Helsinki, and was approved by local Ethics Committees. All subjects signed written informed consents accordingly.
Genotyping for SNP Rs1136410
Genomic DNA of peripheral blood leukocytes was extracted by a phenol-chloroform method. Genotyping for SNP rs1136410 was performed on a LightScanner 96 system (Idaho Technology, Salt Lake City, UT, USA) using high-resolution melting (HRM) analyses (Figure S1; Li et al., 2014). PCR amplification for HRM was conducted with an annealing temperature of 56°C, in 10 μL of PCR mixtures containing 1.5 mM of Mg2+, 200 μM of each dNTP, 0.5 μM of each primer (forward primer: 5′-GGG GGC TTT CTT TTG CTC-3′; reverse primer: 5′-TGT CCA GCA GGT TGT CAAG-3′), 1 U of Taq DNA polymerase, 1 μL of LC Green, and 50 ng of genomic DNA. Genotyping results of HRM were verified by direct sequencing and repeated assays in 10% of the whole samples.
Measurement of PARP Activities, 8-OHdG Levels, and IL-6 Levels in Peripheral Blood Mononuclear Cells (PBMCs)
In PBMCs, PARP activities, combined with two well-known biomarkers for oxidative inflammatory response, 8-OHdG and IL-6, were measured with competitive ELISA kits, as described in Supplementary Materials and Methods (Cui et al., 2017). CV values for inter- and intra- assays were 6.4 and 5.5% for PARP activities, 7.2 and 5.1% for 8-OHdG levels, and 4.5 and 6.7% for IL-6 levels, respectively.
High-Order Gene-Environment Interactions between SNP Rs1136410 and Traditional CAD Risk Factors
To evaluate the high-order gene-environment interactions between SNP rs1136410 and traditional CAD risk factors, MDR and CART analyses were conducted by MDR 2.0 (UPenn, Philadelphia, PA, USA) and Clementine 12.0 (SPSS Inc., Chicago, IL, USA) programs, respectively. Briefly, in MDR analyses (Velez et al., 2007), the predictive accuracy for all combinations of included variables was analyzed by 1,000-time permutation and 100-time cross-validation tests. The best interaction model in predicting CAD risk was identified with the maximum cross-validation consistency (CVC) and the optimal testing accuracy.
According to the relative significance of included variables, CART analyses hierarchically subdivided data to produce a binary recursive-partitioning tree, which identified the best interaction model to predict CAD risk using logistic regression analyses (Barnholtz-Sloan et al., 2011). In CART analyses, each split was based on the Gini index, and required a minimal node size of 50 (Gu et al., 2006; Barnholtz-Sloan et al., 2011).
Statistical Analyses
The differences in demographics between cases and controls were compared by the Student's t-test (for quantitative variables) and the Pearson χ2 test (for qualitative variables). Hardy-Weinberg equilibrium (HWE) for SNP rs1136410 was evaluated by the Pearson χ2 test. Allelic and genotypic (additive, recessive, dominant) associations of SNP rs1136410 with CAD risk were tested by logistic regression analyses before and after adjusting for age, sex, smoking status, alcohol drinking status, BMI, and histories of hyperlipidemia, T2DM, and hypertension. The 100,000-time Monte-Carlo permutation test was used to control for multiple testing (Jiang et al., 2014). The homogeneity of ORs between two sets was analyzed by the Breslow-Day test. In subgroup analyses, we used the multiplicative likelihood ratio test to examine the potential gene-environment interactions in CAD risk. The effect of SNP rs1136410 on CAD severity was appraised by the linear-by-linear association χ2 test. Multivariable linear regression analyses were conducted to test the associations of SNP rs1136410 with lipid levels, IL-6 levels, 8-OHdG levels, and PARP activities, as well as the effects of IL-6 levels, 8-OHdG levels, or PARP activities on CAD risk and vessel scores. We used the Pearson (for normal distributed data) or Spearman (for skewed data) correlation test to assess the correlations between IL-6 levels, 8-OHdG levels, PARP activities, and modified Gensini scores. All these analyses set P < 0.05 (two-tailed) as a significant level in SPSS 17.0 (SPSS Inc., Chicago, IL, USA) and PLINK programs. The statistical power was estimated by PS 3.0 software (Vanderbilt University, Nashville, TN, USA).
Results
Population Demographics
In both discovery and replication sets (Table S1), CAD patients had higher frequencies of smoking habit, alcohol drinking habit, hyperlipidemia, T2DM, and hypertension, higher levels of BMI, blood pressure, FPG, triglyceride (TG), total cholesterol (TC), and low-density lipoprotein cholesterol (LDL-c), and lower levels of high-density lipoprotein cholesterol (HDL-c) than controls. The genotype distributions of SNP rs1136410 fulfilled expectations of HWE in merged controls (PHWE = 0.228).
Allelic and Genotypic Associations between SNP Rs1136410 and CAD Risk
In the discovery set, the minor allele G of SNP rs1136410 conferred an 11% reduced risk of CAD [odds ratio (OR) = 0.89, P = 0.040, Table 1]. This allelic association was successfully verified in the replication set, with an OR of 0.87 and a P-value of 0.006 (Table 1). Since the allelic ORs between discovery and replication sets were homogenous (P = 0.733), we performed a meta-analysis of these two sets, which identified a more significant allelic association between SNP rs1136410 and CAD risk, with an OR of 0.88 and a P-value of 6.29 × 10−4 (Table 1).
In both discovery and replication sets, genotypic association analyses consistently found significant effects of SNP rs1136410 on CAD risk under both additive and recessive models (Table 1). In a meta-analysis of these two sets, the recessive model was identified as the best-fitting model, with the smallest OR of 0.75 and the most significant P-value of 6.53 × 10–5 (Table 1). Assuming a recessive OR of 0.75 and a type I error of 0.05, the entire population could offer a statistical power of 97.5% to address the association.
In subtype analyses based on a recessive model (Table S2), the variant genotype GG of SNP rs1136410 was significantly associated with the decreased risk of UAP and MI, with ORs of 0.71 (P = 0.002) and 0.61 (P = 6.07 × 10–6), respectively. No significant association was found between SNP rs1136410 and SAP.
All significant results remained unchanged after the permutation test for multiple testing and adjustment for covariates (Table 1 and Table S2).
Gene-Environment Interactions between SNP Rs1136410 and Traditional CAD Risk Factors
We first used subgroup analyses to seek two-way gene-environment interactions in CAD risk. Assuming a recessive model (Table 2), the variant genotype GG of SNP rs1136410 constantly conferred a reduced risk of CAD in all subgroups, except for subjects with smoking habit, T2DM, and hyperlipidemia. The multiplicative likelihood ratio test further found the two-way interactions of SNP rs1136410 with smoking status (Pinter = 0.031), history of T2DM (Pinter = 0.025), and history of hyperlipidemia (Pinter = 0.010) in decreasing CAD risk.
To further identify the high-order gene-environment interactions in CAD risk, data on SNP rs1136410 and eight traditional CAD risk factors (age >60, male, smoking habit, alcohol drinking habit, BMI >25, hypertension, T2DM, and hyperlipidemia) were included in the MDR analyses. As presented in Table 3, the four-factor model containing “smoking status,” “history of T2DM,” “history of hyperlipidemia,” and “SNP rs1136410” variables were selected as the best interaction model for predicting CAD risk, with the optimal testing accuracy of 0.6773, the maximal CVC of 100/100, and the smallest P < 0.0001 in the permutation test. Subsequent CART analyses including these four variables showed that individuals with the variant genotype GG but without smoking habit, T2DM, and hyperlipidemia, were associated with an 84% (OR = 0.16, P < 0.001) decreased risk of CAD, compared with the reference group at the greatest risk (Figure 1). Taken together, we suggested the high-order gene-environment interactions among SNP rs1136410, smoking status, history of T2DM, and history of hyperlipidemia in decreasing CAD risk.
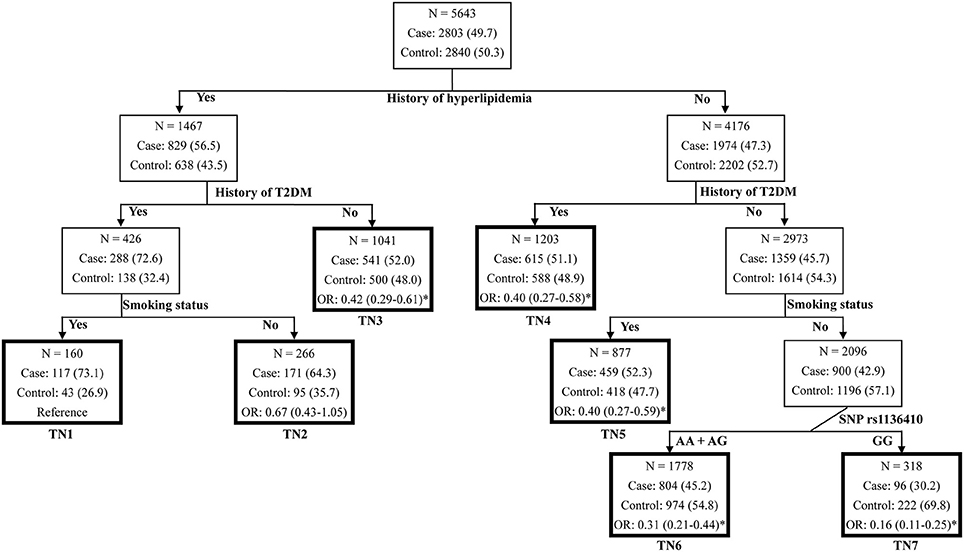
Figure 1. Classification and regression tree for history of hyperlipidemia, history of T2DM, smoking status, and SNP rs1136410 in the merged set. Terminal nodes (TNs) are thick bordered. ORs and 95% CIs were calculated by logistic regression after adjusting for age, sex, smoking status, alcohol drinking status, BMI, and histories of hypertension, hyperlipidemia and T2DM. *P < 0.05.
Effects of SNP Rs1136410 on CAD Severity
First, in CAD patients from discovery (P = 0.044), replication (P = 0.023) and merged sets (P = 0.003, Figure 2 and Table S3), the variant genotype GG of SNP rs1136410 was significantly associated with decreased frequencies of LMCA lesions under a recessive model. Then, in replication (P = 0.015) and merged sets (P = 0.003, Figure 2 and Table S3), the frequencies of the genotype GG showed a decreasing trend from the one-vessel CAD group to the two-vessel CAD group, and ultimately to the three-vessel CAD group. Finally, along with the increasing quartiles (Q1-Q4) of modified Gensini scores, the frequencies of the genotype GG were gradually decreased in patients from all sets (Figure 2 and Table S3).
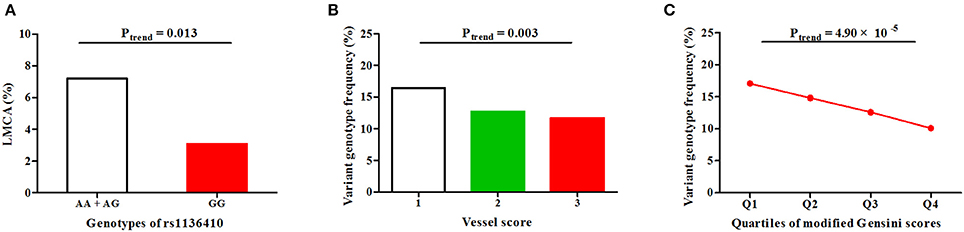
Figure 2. Associations of SNP rs1136410 with CAD severity under a recessive model in the merged set. (A) Association of SNP rs1136410 with LMCA lesions; (B) Associations between SNP rs1136410 and vessel scores; (C) Association of SNP rs1136410 with quartiles of modified Gensini scores. The linear-by-linear association χ2 test was used to assess statistical significance.
Associations of SNP Rs1136410 with Lipid Levels
In controls from the replication set, linear regression analyses using a recessive model observed a significant association between SNP rs1136410 and TC levels, with a 0.139 mmol/L (P = 0.032, Table 4) decrease in TC levels per minor allele. This correlation became more significant in merged controls, with a P-value of 0.005 and a β value of −0.132 (Table 4). Furthermore, the genotype GG was consistently associated with increased HDL-c levels in both cases and controls from all sets (Table 4). In merged controls, the variances of TC and HDL-c levels explained by SNP rs1136410 were 0.40 and 0.82%, respectively.
Effects of PARP Activities, 8-OHdG Levels, and IL-6 Levels on CAD Risk and Severity
In two sets of our study, 120 CAD patients and 120 controls were randomly selected to detect PARP activities, 8-OHdG levels, and IL-6 levels in PBMCs (Table S4). As presented in Figures 3A,B, PARP activities and 8-OHdG levels in CAD patients were significantly higher than those in controls (P < 0.001). A positive correlation was also found between PARP activities and 8-OHdG levels (r = 0.244, P < 0.001, Figure 3C). In subtype analyses (Table S5), patients with UAP and MI had increased PARP activities compared with controls; 8-OHdG levels in all CAD subtypes were significantly higher than those in controls.
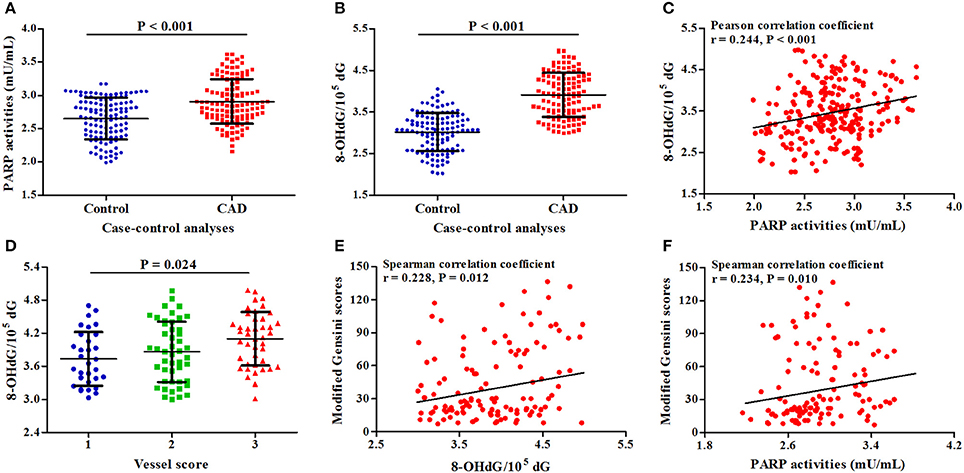
Figure 3. Associations of PARP activities and 8-OHdG levels with CAD risk and severity. (A) Difference in PARP activities between cases and controls; (B) Difference in 8-OHdG levels between cases and controls; (C) Correlation between PARP activities and 8-OHdG levels; (D) Association of 8-OHdG levels with vessel scores; (E) Correlation between 8-OHdG levels and modified Gensini scores; (F) Correlation between 8-OHdG levels and modified Gensini scores.
In CAD patients, along with the increasing levels of 8-OHdG, vessels scores (P = 0.024, Figure 3D) and modified Gensini scores (r = 0.228, P = 0.012, Figure 3E) were gradually increased; there was a positive correlation between PARP activities and modified Gensini scores (r = 0.234, P = 0.010, Figure 3F).
In PBMCs stimulated by lipopolysaccharide (LPS) (Qin et al., 2016a), IL-6 levels were significantly correlated with CAD risk (P < 0.001), 8-OHdG levels (r = 0.318, P < 0.001), PARP activities (r = 0.516, P < 0.001), and modified Gensini scores (r = 0.226, P = 0.013), but not with vessel scores and SNP rs1136410 (Figure S2).
Associations of SNP Rs1136410 with PARP Activities and 8-OHdG Levels
As presented in Figure 4 and Table S5, PARP activities and 8-OHdG levels were gradually decreased from the genotype AA to the heterozygous variant genotype AG and ultimately to the homozygous variant genotype GG in both cases and controls.
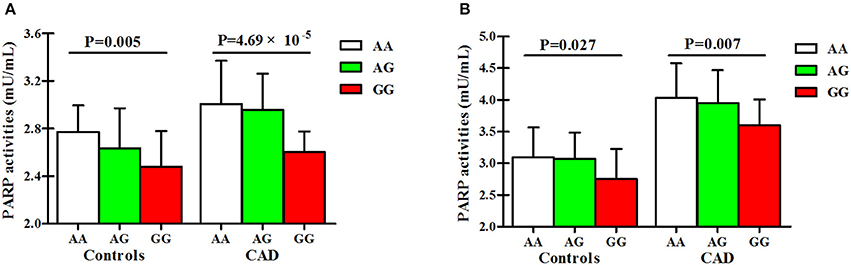
Figure 4. Associations of SNP rs1136410 with PARP activities and 8-OHdG levels. (A) Associations of SNP rs1136410 with PARP activities in controls and patients with UAP and MI; (B) Associations of SNP rs1136410 with 8-OHdG levels in controls and patients with UAP and MI. P-values were obtained from multivariable linear regression models after adjusting for covariates.
Discussion
In SMCs and endothelial cells, hyperactivation of PARP-1 by uncontrolled oxidative DNA damage could trigger a cellular energy crisis and irreversible cell death, which involved the pathogenesis of atherosclerosis (Oumouna-Benachour et al., 2007; Xu et al., 2014). SNP rs1136410 is a non-synonymous mutation, leading to an alanine for valine substitution in the catalytic domain of PARP-1 (Yu et al., 2012). This domain is located in an evolutionarily conserved region, containing a “PARP-signature” motif (Amé et al., 2004; Rouleau et al., 2010; Langelier et al., 2012) which accounts for more than 90% of PARP activities (Aguilar-Quesada et al., 2007). A previous study has suggested a dose-dependent effect of SNP rs1136410 on PARP activities in vitro (Wang et al., 2007). In the current study with more than 5,600 participants, we first reported that the variant genotype GG of SNP rs1136410 was associated with decreased CAD risk, reduced CAD severity, lower TC levels, and higher HDL-c levels in a Chinese Han population. Then, MDR and CART analyses further observed the high-order gene-environment interactions among SNP 1136410, smoking status, history of T2DM, and history of hyperlipidemia in decreasing CAD risk. Finally, the genotype-phenotype correlation analyses supported the functional roles of SNP rs1136410 in decreasing PARP activities and 8-OHdG levels. Taken together, we suggest that the minor allele G of SNP rs1136410 may protect against CAD through gene-environment interactions and regulation of PARP activities.
In population studies, Cottet et al. first introduced SNP rs1136410, which was not associated with cellular poly(ADP-ribosyl)ation capacity of PARP-1 in 95 centenarians (Cottet et al., 2000). Then, a larger cohort involving 354 subjects reported an allele dose-dependent impact of SNP rs1136410 on PARP activities (Lockett et al., 2004). Subsequent in vitro analyses also suggested functional roles of SNP rs1136410 in maximum velocity and KM values of poly(ADP-ribosyl)ation by PARP-1 (Beneke et al., 2010). In this study, PARP activities were gradually decreased from the genotype AA to the heterozygous variant genotype AG and ultimately to the homozygous variant genotype GG in both cases and controls. Moreover, by integrating the mRNA expression data from ArrayExpress database (https://www.ebi.ac.uk/arrayexpress/experiments/E-MTAB-264/) and the genotyping data from HapMap Project in 160 East Asians (ftp://ftp.ncbi.nlm.nih.gov/hapmap/) (Stranger et al., 2012), we found that carriers not only with the homozygous variant GG, but with the heterozygous variant AG, had significantly lower levels of PARP-1 mRNA expression than the homozygous wild-type (Table S6). All the evidence above suggests a cumulative effect of SNP rs1136410 on PARP-1 function.
CAD is a continuous process from SAP to UAP and ultimately to MI, which mainly results from the progression of plaque dynamics (Andersson and Vasan, 2014). In functional studies, PARP inhibition or deletion could lead to an increase in collagen content (Oumouna-Benachour et al., 2007), the prevention of dyslipidemia-induced endothelial dysfunction (Hans et al., 2009a), and the reduction in plaque sizes and numbers (Oumouna-Benachour et al., 2007; von Lukowicz et al., 2008; Hans et al., 2009a), suggesting the key roles of PARP activities in plaque progression (Oumouna-Benachour et al., 2007). However, a case-control study from India failed to find a significant association between SNP rs1136410 and MI in patients with T2DM (Narne et al., 2012). Considering the relatively small sample size of Narne et al.'s study (73 MI patients and 121 controls) and the different genetic background between Indians and Chinese (a minor allele frequency of rs1136410: 23.1% in Narne et al.'s controls; 42.7% in our controls), we performed subtype analyses in two sets of our study, and found the protective effects of SNP rs1136410 on MI and UAP. Then, patients with MI and UAP had higher PARP activities than controls. Finally, the variant genotype GG of SNP rs1136410 showed a significantly inverse association with CAD severity, as determined by LMCA lesions, vessel scores, and modified Gensini scores. All these results together indicate that SNP rs1136410 and PARP activities may involve the progression of CAD, and exert greater effects on UAP and MI than on SAP in the Chinese Han population.
In mice, PARP-1 ablation or treatment of PARP inhibitors could dramatically reduce TC (Hans et al., 2009a; Bai et al., 2011) and LDL-c levels (Hans et al., 2009a,b), elevate HDL-c levels (Hans et al., 2009a), and consequently ameliorate the atherogenic index (Hans et al., 2009a) on either regular or high fat diet. This evidence, combined with the decreased effect of SNP rs1136410 on PARP activities, promoted us to test whether SNP rs1136410 was associated with lipid levels in our population study. We found a constantly strong association between SNP rs1136410 and increased HDL-c levels in both cases and controls, and a modest effect of SNP rs1136410 on decreased TC levels only in controls. Recently, functional studies further showed that PARP inhibition in macrophages enhanced ABCA1-induced cholesterol efflux (Shrestha et al., 2016), and increased the detoxification of free cholesterol by down-regulation of ACAT-1 expression (Hans et al., 2008) (a gene suppressing cholesterol efflux (Hongo et al., 2009). Considering all the evidence above, it is reasonable to speculate that SNP rs1136410 may regulate HDL-c metabolism by reducing PARP activities and subsequently enhancing cholesterol efflux.
In the present study, participants with the variant genotype GG but without smoking habit, T2DM, and hyperlipidemia were associated with an 84% decreased risk of CAD, suggesting the existence of gene-environment interactions. The following evidence may help to interpret this finding. First, as a well-established risk factor for CAD, smoking habit could contribute to oxidative DNA damage and atheroma formation, either directly by invoking peroxidation, or indirectly by consuming endogenous antioxidants (Siasos et al., 2014). Its enhanced effects on oxidative stress may weaken the protective role of SNP rs1136410 in CAD development. Second, PARP-1 mRNA was overexpressed in T2DM patients (Adaikalakoteswari et al., 2007), and PARP inhibition protected cardiomyocytes from endothelium dysfunction (Choi et al., 2012; Zakaria et al., 2016), glucose stimulation (Choi et al., 2012; Qin et al., 2016b), and apoptosis (Qin et al., 2016b) in mice with T2DM or diabetic cardiomyopathy. So, by reducing PARP activities, SNP rs1136410 may protect against dysglycemia in cardiomyocytes, and further reduce CAD risk. Third, the current study found significant associations of SNP rs1136410 with TC and HDL-c levels, suggesting a potential role of SNP rs1136410 in hyperlipidemia. Taking all the evidence together, we suggest that the protective effect of SNP rs1136410 on CAD risk may greatly enhance in subjects without smoking habit, T2DM, and hyperlipidemia.
Limitations of this study merit further consideration. First, the ELISA kit used for PARP activities only measures newly formed PAR, but not the PAR already presented in the sample, hampering the interpretation of these data. Second, although we analyzed the association between PARP activities and SNP rs1136410, other biomarkers for PARP enzymes, including PARP-1 protein levels and PAR structure, are encouraged to detect. Finally, despite a sufficient statistical power of this study, the Spearman correlation test only showed modest effects of 8-OHdG levels and PARP activities on modified Gensini scores. This finding needs further replication.
In summary, this two-stage case-control study, involving 2803 cases and 2840 controls, shows that the minor allele G of SNP rs1136410 may protect against CAD (risk and severity), reduce TC levels, and increase HDL-c levels through regulation of PARP activities. We also identify the gene-environment interactions among SNP rs1136410, smoking status, history of T2DM, and history of hyperlipidemia in modulating CAD risk. Future studies are warranted to validate these findings and determine the molecular mechanism.
Author Contributions
XW and LM conceived, designed, and performed the study; NC and SG analyzed the data; XW, SZ, and ZL contributed samples/materials/reagents; XW and LM wrote and drafted the manuscript.
Conflict of Interest Statement
The authors declare that the research was conducted in the absence of any commercial or financial relationships that could be construed as a potential conflict of interest.
Acknowledgments
This study was supported by grants from the National Basic Research Program of China (81300154) and the Key Project on Science and Technology Research provided by Henan Province, China (No. 152102410067; No: 162102310142).
Supplementary Material
The Supplementary Material for this article can be found online at: https://www.frontiersin.org/articles/10.3389/fphys.2017.00916/full#supplementary-material
References
Adaikalakoteswari, A., Rema, M., Mohan, V., and Balasubramanyam, M. (2007). Oxidative DNA damage and augmentation of poly(ADP-ribose) polymerase/nuclear factor-kappa B signaling in patients with type 2 diabetes and microangiopathy. Int. J. Biochem. Cell Biol. 39, 1673–1684. doi: 10.1016/j.biocel.2007.04.013
Aguilar-Quesada, R., Muñoz-Gámez, J. A., Martín-Oliva, D., Peralta-Leal, A., Quiles-Pérez, R., Rodríguez-Vargas, J. M., et al. (2007). Modulation of transcription by PARP-1: consequences in carcinogenesis and inflammation. Curr. Med. Chem. 14, 1179–1187. doi: 10.2174/092986707780597998
Altmeyer, M., Messner, S., Hassa, P. O., Fey, M., and Hottiger, M. O. (2009). Molecular mechanism of poly(ADP-ribosyl)ation by PARP1 and identification of lysine residues as ADP-ribose acceptor sites. Nucleic Acids Res. 37, 3723–3738. doi: 10.1093/nar/gkp229
Amé, J. C., Spenlehauer, C., and de Murcia, G. (2004). The PARP superfamily. Bioessays 26, 882–893. doi: 10.1002/bies.20085
Andersson, C., and Vasan, R. S. (2014). Compiling the complement of genes implicated in coronary artery disease. Circ. Cardiovasc. Genet. 7, 738–740. doi: 10.1161/CIRCGENETICS.114.000909
Bai, P., Cantó, C., Oudart, H., Brunyánszki, A., Cen, Y., Thomas, C., et al. (2011). PARP-1 inhibition increases mitochondrial metabolism through SIRT1 activation. Cell Metab. 13, 461–468. doi: 10.1016/j.cmet.2011.03.004
Barnholtz-Sloan, J. S., Guan, X., Zeigler-Johnson, C., Meropol, N. J., and Rebbeck, T. R. (2011). Decision tree-based modeling of androgen pathway genes and prostate cancer risk. Cancer Epidemiol. Biomarkers Prev. 20, 1146–1155. doi: 10.1158/1055-9965.EPI-10-0996
Beneke, S., Scherr, A. L., Ponath, V., Popp, O., and Bürkle, A. (2010). Enzyme characteristics of recombinant poly(ADP-ribose) polymerases-1 of rat and human origin mirror the correlation between cellular poly(ADP-ribosyl)ation capacity and species-specific life span. Mech. Ageing Dev. 131, 366–369. doi: 10.1016/j.mad.2010.04.003
Berger, N. A., Sims, J. L., Catino, D. M., and Berger, S. J. (1983). Poly(ADP-ribose) polymerase mediates the suicide response to massive DNA damage: studies in normal and DNA-repair defective cells. Princess Takamatsu Symp. 13, 219–226.
Bing, R., Yong, A. S., and Lowe, H. C. (2015). Percutaneous transcatheter assessment of the left main coronary artery: current status and future directions. JACC Cardiovasc. Interv. 8, 1529–1539. doi: 10.1016/j.jcin.2015.07.017
Choi, S. K., Galán, M., Kassan, M., Partyka, M., Trebak, M., and Matrougui, K. (2012). Poly(ADP-ribose) polymerase 1 inhibition improves coronary arteriole function in type 2 diabetes mellitus. Hypertension 59, 1060–1068. doi: 10.1161/HYPERTENSIONAHA.111.190140
Cottet, F., Blanché, H., Verasdonck, P., Le Gall, I., Schächter, F., Bürkle, A., et al. (2000). New polymorphisms in the human poly(ADP-ribose) polymerase-1 coding sequence: lack of association with longevity or with increased cellular poly(ADP-ribosyl)ation capacity. J. Mol. Med. 78, 431–440. doi: 10.1007/s001090000132
Cui, N. H., Qiao, C., Chang, X. K., and Wei, L. (2017). Associations of PARP-1 variant rs1136410 with PARP activities, oxidative DNA damage, and the risk of age-related cataract in a Chinese Han population: a two-stage case-control analysis. Gene 600, 70–76. doi: 10.1016/j.gene.2016.11.019
El-Khamisy, S. F., Masutani, M., Suzuki, H., and Caldecott, K. W. (2003). A requirement for PARP-1 for the assembly or stability of XRCC1 nuclear foci at sites of oxidative DNA damage. Nucleic Acids Res. 31, 5526–5533. doi: 10.1093/nar/gkg761
Gu, D., Su, S., Ge, D., Chen, S., Huang, J., Li, B., et al. (2006). Association study with 33 single-nucleotide polymorphisms in 11 candidate genes for hypertension in Chinese. Hypertension 47, 1147–1154. doi: 10.1161/01.HYP.0000219041.66702.45
Hans, C. P., Feng, Y., Naura, A. S., Zerfaoui, M., Rezk, B. M., Xia, H., et al. (2009a). Protective effects of PARP-1 knockout on dyslipidemia-induced autonomic and vascular dysfunction in ApoE mice: effects on eNOS and oxidative stress. PLoS ONE 4:e7430. doi: 10.1371/journal.pone.0007430
Hans, C. P., Zerfaoui, M., Naura, A. S., Catling, A., and Boulares, A. H. (2008). Differential effects of PARP inhibition on vascular cell survival and ACAT-1 expression favouring atherosclerotic plaque stability. Cardiovasc. Res. 78, 429–439. doi: 10.1093/cvr/cvn018
Hans, C. P., Zerfaoui, M., Naura, A. S., Troxclair, D., Strong, J. P., Matrougui, K., et al. (2009b). Thieno[2,3-c]isoquinolin-5-one, a potent poly(ADP-ribose) polymerase inhibitor, promotes atherosclerotic plaque regression in high-fat diet-fed apolipoprotein E-deficient mice: effects on inflammatory markers and lipid content. J. Pharmacol. Exp. Ther. 329, 150–158. doi: 10.1124/jpet.108.145938
Harrison, D., Griendling, K. K., Landmesser, U., Hornig, B., and Drexler, H. (2003). Role of oxidative stress in atherosclerosis. Am. J. Cardiol. 91, 7a−11a. doi: 10.1016/S0002-9149(02)03144-2
He, F., and Zuo, L. (2015). Redox roles of reactive oxygen species in cardiovascular diseases. Int. J. Mol. Sci. 16, 27770–27780. doi: 10.3390/ijms161126059
Hongo, S., Watanabe, T., Arita, S., Kanome, T., Kageyama, H., Shioda, S., et al. (2009). Leptin modulates ACAT1 expression and cholesterol efflux from human macrophages. Am. J. Physiol. Endocrinol. Metab. 297, E474–E482. doi: 10.1152/ajpendo.90369.2008
Huang, D., Yang, C. Z., Yao, L., Wang, Y., Liao, Y. H., and Huang, K. (2008). Activation and overexpression of PARP-1 in circulating mononuclear cells promote TNF-alpha and IL-6 expression in patients with unstable angina. Arch. Med. Res. 39, 775–784. doi: 10.1016/j.arcmed.2008.09.003
Jiang, Y., Zhang, L., Kong, F., Zhang, M., Lv, H., Liu, G., et al. (2014). MCPerm: a Monte Carlo permutation method for accurately correcting the multiple testing in a meta-analysis of genetic association studies. PLoS ONE 9:e89212. doi: 10.1371/journal.pone.0089212
Langelier, M. F., Planck, J. L., Roy, S., and Pascal, J. M. (2012). Structural basis for DNA damage-dependent poly(ADP-ribosyl)ation by human PARP-1. Science 336, 728–732. doi: 10.1126/science.1216338
Lanktree, M. B., and Hegele, R. A. (2009). Gene-gene and gene-environment interactions: new insights into the prevention, detection and management of coronary artery disease. Genome Med. 1:28. doi: 10.1186/gm28
Li, M., Zhou, L., Palais, R. A., and Wittwer, C. T. (2014). Genotyping accuracy of high-resolution DNA melting instruments. Clin. Chem. 60, 864–872. doi: 10.1373/clinchem.2013.220160
Lockett, K. L., Hall, M. C., Xu, J., Zheng, S. L., Berwick, M., Chuang, S. C., et al. (2004). The ADPRT V762A genetic variant contributes to prostate cancer susceptibility and deficient enzyme function. Cancer Res. 64, 6344–6348. doi: 10.1158/0008-5472.CAN-04-0338
Martinet, W., Knaapen, M. W., De Meyer, G. R., Herman, A. G., and Kockx, M. M. (2002). Elevated levels of oxidative DNA damage and DNA repair enzymes in human atherosclerotic plaques. Circulation 106, 927–932. doi: 10.1161/01.CIR.0000026393.47805.21
Montorsi, P., Ravagnani, P. M., Galli, S., Rotatori, F., Veglia, F., Briganti, A., et al. (2006). Association between erectile dysfunction and coronary artery disease. Role of coronary clinical presentation and extent of coronary vessels involvement: the COBRA trial. Eur. Heart J. 27, 2632–2639. doi: 10.1093/eurheartj/ehl142
Mozaffarian, D., Benjamin, E. J., Go, A. S., Arnett, D. K., Blaha, M. J., Cushman, M., et al. (2016). Executive summary: heart disease and stroke statistics−2016 update: a report from the american heart association. Circulation 133, 447–454. doi: 10.1161/CIR.0000000000000366
Narne, P., Ponnaluri, K. C., Singh, S., Siraj, M., and Ishaq, M. (2012). Relationship between NADPH oxidase p22phox C242T, PARP-1 Val762Ala polymorphisms, angiographically verified coronary artery disease and myocardial infarction in South Indian patients with type 2 diabetes mellitus. Thromb. Res. 130, e259–e265. doi: 10.1016/j.thromres.2012.09.012
Oumouna-Benachour, K., Hans, C. P., Suzuki, Y., Naura, A., Datta, R., Belmadani, S., et al. (2007). Poly(ADP-ribose) polymerase inhibition reduces atherosclerotic plaque size and promotes factors of plaque stability in apolipoprotein E-deficient mice: effects on macrophage recruitment, nuclear factor-kappaB nucleartranslocation, and foam cell death. Circulation 115, 2442–2450. doi: 10.1161/CIRCULATIONAHA.106.668756
Qin, J., Li, L., Zhang, D., Yu, H., Tan, H., Zhang, J., et al. (2016a). Analysis of receptor tyrosine kinase genetics identifies two novel risk loci in GAS6 and PROS1 in Behcet's disease. Sci. Rep. 6:26662. doi: 10.1038/srep26662
Qin, W. D., Liu, G. L., Wang, J., Wang, H., Zhang, J. N., Zhang, F., et al. (2016b). Poly(ADP-ribose) polymerase 1 inhibition protects cardiomyocytes from inflammation and apoptosis in diabetic cardiomyopathy. Oncotarget 7, 35618–35631. doi: 10.18632/oncotarget.8343
Rouleau, M., Patel, A., Hendzel, M. J., Kaufmann, S. H., and Poirier, G. G. (2010). PARP inhibition: PARP1 and beyond. Nat. Rev. Cancer 10, 293–301. doi: 10.1038/nrc2812
Shah, N. R., and Mahmoudi, M. (2015). The role of DNA damage and repair in atherosclerosis: a review. J. Mol. Cell. Cardiol. 86, 147–157. doi: 10.1016/j.yjmcc.2015.07.005
Shrestha, E., Hussein, M. A., Savas, J. N., Ouimet, M., Barrett, T. J., Leone, S., et al. (2016). Poly(ADP-ribose) polymerase 1 represses liver X receptor-mediated ABCA1 expression and cholesterol efflux in macrophages. J. Biol. Chem. 291, 11172–11184. doi: 10.1074/jbc.M116.726729
Siasos, G., Tsigkou, V., Kokkou, E., Oikonomou, E., Vavuranakis, M., Vlachopoulos, C., et al. (2014). Smoking and atherosclerosis: mechanisms of disease and new therapeutic approaches. Curr. Med. Chem. 21, 3936–3948. doi: 10.2174/092986732134141015161539
Stranger, B. E., Montgomery, S. B., Dimas, A. S., Parts, L., Stegle, O., Ingle, C. E., et al. (2012). Patterns of cis regulatory variation in diverse human populations. PLoS Genet. 8:e1002639. doi: 10.1371/journal.pgen.1002639
Velez, D. R., White, B. C., Motsinger, A. A., Bush, W. S., Ritchie, M. D., Williams, S. M., et al. (2007). A balanced accuracy function for epistasis modeling in imbalanced datasets using multifactor dimensionality reduction. Genet. Epidemiol. 31, 306–315. doi: 10.1002/gepi.20211
von Lukowicz, T., Hassa, P. O., Lohmann, C., Borén, J., Braunersreuther, V., Mach, F., et al. (2008). PARP1 is required for adhesion molecule expression in atherogenesis. Cardiovasc. Res. 78, 158–166. doi: 10.1093/cvr/cvm110
Wang, L., Chen, J., Zeng, Y., Wei, J., Jing, J., Li, G., et al. (2016). Functional variant in the SLC22A3-LPAL2-LPA gene cluster contributes to the severity of coronary artery disease. Arterioscler. Thromb. Vasc. Biol. 36, 1989–1996. doi: 10.1161/ATVBAHA.116.307311
Wang, X. G., Wang, Z. Q., Tong, W. M., and Shen, Y. (2007). PARP1 Val762Ala polymorphism reduces enzymatic activity. Biochem. Biophys. Res. Commun. 354, 122–126. doi: 10.1016/j.bbrc.2006.12.162
Weintraub, W. S., Karlsberg, R. P., Tcheng, J. E., Boris, J. R., Buxton, A. E., Dove, J. T., et al. (2011). ACCF/AHA 2011 key data elements and definitions of a base cardiovascular vocabulary for electronic health records: a report of the American College of Cardiology Foundation/American Heart Association Task Force on Clinical Data Standards. J. Am. Coll. Cardiol. 58, 202–222. doi: 10.1016/j.jacc.2011.05.001
Xu, S., Bai, P., Little, P. J., and Liu, P. (2014). Poly(ADP-ribose) polymerase 1 (PARP1) in atherosclerosis: from molecular mechanisms to therapeutic implications. Med. Res. Rev. 34, 644–675. doi: 10.1002/med.21300
Yu, H., Ma, H., Yin, M., and Wei, Q. (2012). Association between PARP-1 V762A polymorphism and cancer susceptibility: a meta-analysis. Genet. Epidemiol. 36, 56–65. doi: 10.1002/gepi.20663
Keywords: PARP-1 rs1136410, PARP activities, coronary artery disease, severity of coronary atherosclerosis, gene-environment interactions
Citation: Wang X, Cui N, Zhang S, Guo S, Liu Z and Ming L (2017) PARP-1 Variant Rs1136410 Confers Protection against Coronary Artery Disease in a Chinese Han Population: A Two-Stage Case-Control Study Involving 5643 Subjects. Front. Physiol. 8:916. doi: 10.3389/fphys.2017.00916
Received: 07 September 2017; Accepted: 30 October 2017;
Published: 14 November 2017.
Edited by:
John D. Imig, Medical College of Wisconsin, United StatesReviewed by:
Sascha Beneke, University of Konstanz, GermanySergei Noskov, University of Calgary, Canada
Copyright © 2017 Wang, Cui, Zhang, Guo, Liu and Ming. This is an open-access article distributed under the terms of the Creative Commons Attribution License (CC BY). The use, distribution or reproduction in other forums is permitted, provided the original author(s) or licensor are credited and that the original publication in this journal is cited, in accordance with accepted academic practice. No use, distribution or reproduction is permitted which does not comply with these terms.
*Correspondence: Liang Ming, mingliang3072@163.com