- 1Laboratory of Immunoparasitology, Institute of Biomedical Sciences, Universidade Federal de Uberlândia, Uberlândia, Brazil
- 2Fertilizer Technology Laboratory, Institute of Agricultural Sciences, Universidade Federal de Uberlândia, Uberlândia, Brazil
- 3Laboratory of Plant Histopathology and Structural Biology of Plants, Center for Nuclear Energy in Agriculture, Universidade de São Paulo, Piracicaba, Brazil
- 4Mediplant, Swiss Research Centre on Medicinal and Aromatic Plants, Conthey, Switzerland
Artemisia annua is used as a source of artemisinin, a potent therapeutic agent used for the treatment of infectious diseases, chiefly malaria. However, the low concentration (from 0.01 to 1.4% of dried leaf matter) of artemisinin in the plant obtained with the traditional cropping system makes it a relatively expensive drug, especially in developing countries. Considering that artemisinin and silicon (Si) are both stored in A. annua glandular trichomes, and that Si accumulation has never been investigated, this study aimed to look into Si effects on A. annua trichome artemisinin concentration, and whether leaf infusion from Si-treated A. annua plants is able to control Toxoplasma gondii growth. T. gondii is the etiologic agent of toxoplasmosis, a zoonotic parasitic disease whose traditional treatment shows significant side effects. The experimental design consisted of A. annua seedlings randomly planted in soil treated with different doses of calcium/magnesium silicate (0, 200, 400, 800, and 1600 kg ha-1). Analysis of foliar macronutrients showed significant increases of nitrogen content only at the highest dose of silicate. Foliar micronutrients, Si concentrations, and plant height were not affected by any of the silicate doses. However, the dose of 400 kg ha-1 of silicate increased the trichome size, which in turn raised artemisinin concentration in leaves and the infusion. In contrast, the 800 and 1600 kg ha-1 doses dramatically decreased artemisinin concentration. HeLa cell treatment with the infusion of A. annua grown in soil treated with 400 kg ha-1 of silicate decreased parasite proliferation in a dose-dependent manner when the treatment was carried out after or along with T. gondii infection. However, this effect was similar to A. annua grown in soil without silicate treatment. Thus, it can be concluded that, even though Si applied to the soil at 400 kg ha-1 has a positive effect on the A. annua glandular trichome size and the artemisinin concentration, this outcome cannot be directly associated with the efficiency of A. annua infusion on T. gondii growth, suggesting that other components from A. annua leaves could be acting in synergy with artemisinin.
Introduction
Artemisinin is a sesquiterpene lactone endoperoxide, that is produced and stored in glandular trichomes of Artemisia annua; which are present especially on the surfaces of leaves and flowers. Trichomes also accumulate elements such as silicon (Si), which is present in cell wall in the form of silica. Therefore, knowing that the trichome is a specific site of artemisinin biosynthesis and sequestration, it may be useful to investigate the effect of Si on trichome density, its morphology and the accumulation of artemisinin in these plant structures, as it has already been analyzed for wheat trichomes (Duke and Paul, 1993; Wagner et al., 2004). A. annua infusion containing artemisinin has been used to treat malaria since its discovery in the Chinese herbal garden (Miller and Su, 2011). More recently, A. annua and its active components, such as artemisinin, have been used to treat other protozoan infections (Derda et al., 2016; Golami et al., 2016). However, one of the major handicaps is the low concentration of artemisinin in the infusion, justifying the quest for alternative pathways to increase the concentration of this active compound (Bryant et al., 2016; Kiani et al., 2016; Pandey and Pandey-Rai, 2016). In Brazil, studies with A. annua started with the initiative of a group of researchers who imported the plant, isolated the active ingredient and worked to obtain hybrid plants able to deliver higher levels of artemisinin (Magalhães et al., 1999).
Silicon is an abundant element in the soil, mainly found as silica and silicate minerals. It is considered a beneficial element, which can improve crop growth, production, and tolerance to biotic and abiotic stress factors (Datnoff et al., 2001; Epstein, 2009). Indeed, Si has been used for centuries to prevent diseases in agriculture. The deposition of Si in the cell wall of wheat leaves and awns is correlated with the localization of silica in the trichomes, giving the leaves their roughness and toughness; acting as a physical barrier by impeding the penetration of the epidermis by herbivores and pathogens (Epstein, 2009). Previous study using scanning electron microscopy (SEM) in addition to X-ray microanalysis clearly demonstrated the deposition of solid, amorphous silica, Si02 – nH20, or “opal phytoliths” in the trichomes of wheat plants (Epstein, 2009). However, to the best of our knowledge, there is no data elucidating the effect of Si on the A. annua physiology, particularly considering its effect on the artemisinin content in glandular trichomes.
Toxoplasmosis is caused by Toxoplasma gondii, an obligate intracellular protozoan whose life cycle involves Felidae definitive hosts and warm-blooded intermediate hosts, including domestic and wild animals, birds, and humans (Tenter et al., 2000). The hosts are infected by ingesting sporulated oocysts with water or food, previously contaminated by infected cats, or by ingesting bradyzoites within tissue cysts in raw or undercooked meat from infected animals. Fetuses can also be infected by vertical transmission of tachyzoites during primary maternal infection. Most infections are asymptomatic in immunocompetent subjects; however, they can be severe in immunocompromised patients, as opportunistic infections cause miscarriages or birth defects in pregnant non-immune animals and humans (Boothroyd and Grigg, 2002; Montoya and Liesenfeld, 2004).
This study aimed to explore the effects of Si on the A. annua physiology and artemisinin content, as well as the possibility to control T. gondii growth in cell culture with infusion from A. annua plants treated with various Si doses. Considering the clinical importance of toxoplasmosis for determined patients at risk, in addition to the substantial side effects of the currently available chemotherapy, which displays high toxicity (Haverkos, 1987; Leport et al., 1988), the effectiveness of artemisinin and its derivatives to control T. gondii growth constitutes a new pathway of treatment of T. gondii infection, due to its low toxicity and inhibitory effect on the parasite.
Materials and Methods
Experimental Site
Seeds of A. annua L. were provided by Dr. Pedro Melillo de Magalhães (University of Campinas-UNICAMP, Brazil). The soil was collected from the top 20 cm layer, air dried, sieved through a 5-mm screen and homogenized. The soil was classified as eutroferric red latosol (clayey Oxisol). Soil chemical analysis demonstrated the following results: pH H2O 4.4; P 3.4 mg dm-3 (extracted with H2SO4 0.0125 mol L-1 + HCl 0.05 mol L-1); Al 1.0 Cmolc dm-3; Ca 0.1 Cmolc dm-3;H+Al 6,90 Cmolc dm-3; Mg 0.1 Cmolc dm-3; K 0.17 Cmolc dm-3; Sum of bases 0.37 Cmolc dm-3; effective CEC 1.37 Cmolc dm-3; CEC (pH 7.0) 7.7 Cmolc dm-3; base saturation 5.0%; aluminum saturation 73%; and organic matter 33 g kg-1. The values for B, Cu, Fe, Mn, and Zn were 0.23, 0.6, 79, 3.3, and 0.6 mg dm-3, respectively. Si content in the initial soil was 7.8 mg kg-1.
The experimental design consisted of five randomized blocks with five replications. A. annua seedlings, each approximately 15 cm tall (4–8 weeks after planting), were transplanted to pots containing 10 kg of soil with different Si doses (an equivalent of 0, 200, 400, 800, and 1600 kg ha-1) of calcium and magnesium silicate (MgCaSiO3: 25% of Ca, 6% of Mg, and 10.5% of Si). Each pot was fertilized with: 8.33 g of superphosphate; 1.11 g of urea; 2.58 g of KCl; 0.5 g of FTE; and Recmix (Ca and Mg silicate: T1 = 0 g per pot, T2 = 9.52 g per pot, T3 = 19.05 g per pot, T4 = 38.10 g per pot, and T5 = 76.19 g per pot). Fertilizers were incorporated homogeneously to the entire volume of soil and the pots were randomly arranged in a greenhouse (ICIAG, UFU, Brazil). The plants were periodically irrigated. No herbicides or pesticides were applied to the plants.
Analysis of Macro and Micronutrients, and Si Content In A. annua
The analysis of macro and micronutrients in A. annua leaves was performed according to the protocol described by Embrapa, Centro Nacional de Pesquisa de Solos (2009). The Si analysis in A. annua plants were carried out according to the method described by Elliott and Snyder (1991) and adopted by Korndörfer et al. (2004). Accordingly, leaf samples were weighed (0.1 g) and placed into plastic tubes, then 2 ml of H2O2 (30 or 50%) and 3 ml of NaOH (1:1) were added. After stirring, the tubes were immediately autoclaved for 1 h at 123°C and 1.5 atm. The digested material was mixed with 2 mL of ammonium molybdate, 1:5 [(NH4)6 Mo7O24.4H2O: distilled water] forming the yellow complex of silicomolybdic acid [H4 (SiMo12O40)]. Formation of the silicomolybdic acid is maximum between pH 1.0 and 2.0. To lower the pH of the samples, the proportional volume of HCl (50%) was added to the aliquot. The 1-amino-2-naphthol-4-sulfonic acid (reducer) used to eliminate the interference of P and Fe was substituted by 2 mL of oxalic acid [75 g of (COOH)2. 2H2O in 200 mL of distilled water] per sample. Si concentrations in the extracts were read on a photocolorimeter at 410 nm wavelength. The results of foliar Si in relation to the dry weight of the leaves were expressed as mg kg-1 of dry weight of leaves.
Analysis of Glandular Trichomes In A. annua Leaves
As the artemisinin and Si are deposited in glandular trichomes, the experiment aimed to verify the effect of Si treatments on the size and integrity of A. annua glandular trichomes. The analysis of the trichomes in leaves was performed according to the method described by Kitajima and Leite (1999). First, a leaf from the middle third of each plant in all treatments was collected in the morning, washed, dried, cut into pieces, and immediately fixed using modified Karnowsky solution (2% glutaraldehyde, 0.05 M sodium cacodylate buffer, pH 7.2; Karnovsky, 1965). The post-fixation was performed with osmium tetroxide in 1% sodium cacodylate buffer 0.05 M, pH 7.2, for 1 h. Then, the samples were immersed three times in distilled water and dehydrated in increasing concentrations of alcohol (30, 50, 70, and 80%). Next, the samples were washed three times with 100% alcohol and dried to the critical point using liquid carbon dioxide. The dried samples were mounted on small metal cylinders and bathed in 20 nm gold in MED 010 evaporator (Balzer Union Ltd, Balzers, Liechtenstein). The images were obtained with Zeiss LEO 435 VP scanning electron microscope (Leo Electron Microscopy Ltd Cooperation Zeiss Leica, Cambridge, England) at 20 kV. Part of this material was covered with carbon for X-ray microanalysis by Zeiss 940 DSM-A scanning microscope (Carl Zeiss Inc, Oberkochen, Germany). The images obtained were used for the measurements of total and intact glandular trichomes with the aid of the ImageJ analysis software, as previously described (NIH, Bethesda, MD, USA; Cornelissen et al., 2003).
Determination of Artemisinin Content In A. annua Leaves
Artemisinin content was determined in A. annua leaves by thin layer chromatography (TLC), according to Quennoz et al. (2010). Fresh leaves were collected, dried, ground, and sent to Mediplant, Center for Research on Medicinal Plants, Conthey, Switzerland. Densitometric evaluation was performed with a CAMAG TLC scanner (CAMAG, Muttens, Switzerland). Artemisinin content was expressed in percentage (grams of artemisinin per 100 g of dry weight).
Preparation of A. annua Infusion
The leaves of A. annua were harvested before the flowering period for the best artemisinin content. Immediately after the harvest, the leaves were washed with distilled water, dried in a forced circulation oven at 45°C for 24 h, grounded in a Wiley mill, packed in labeled plastic bags and stored at low humidity and temperature. Subsequently, part of the material was subjected to the analysis of macro and micronutrients and the quantification of leaf Si, while the other part was infused and lyophilized for the analysis of artemisinin content.
The A. annua infusion was prepared by adding 10 g of dried leaves to 100 mL of boiling distilled water. The mixture was briefly stirred and covered for 10 min. Next, the plant material was removed by filtration and cooled down at room temperature (Räth et al., 2004). The A. annua L. infusion (100 mg mL-1) was distributed into 1 mL aliquots, lyophilized and stored at 4°C for the analysis by high performance liquid chromatography (HPLC). Part of this stock solution was filtered through syringe filters with 0.2 μm pore size (Millipore Corporation, Bedford, MA, USA) and immediately used in cytotoxicity assays.
Determination of Artemisinin Content In A. annua Infusion
The lyophilized stock solution of A. annua infusion was resuspended in 1 mL of acetonitrile (Sigma-Aldrich Corporation, St. Louis, MO, USA) and centrifuged at 13,400 g for 5 min. The supernatant was filtered through syringe filters with 0.2 μm pore size (Millipore Corporation, Bedford, MA, USA) prior to HPLC analysis. Artemisinin content was determined using the Shimadzu Prominence SPD-M20A HPLC PDA detector equipped with a UV-vis diode array detectors and LC Solution software (Shimadzu Co., Kyoto, Japan), and based on the standard calibration curve of pure artemisinin (Sigma-Aldrich Corporation, St. Louis, MO, USA) with twofold serial dilutions from 500 to 31.25 μg mL-1.
The twofold serial dilutions consisted of a test tube with the stock solution (500 μg of pure artemisinin + 1 mL of acetonitrile) and five other test tubes each containing 500 mL of acetonitrile (solvent). Using a micropipette, 500 mL of the stock solution was transferred to the first tube. After mixing, 500 mL from this tube was transferred to the next one. The same procedure was carried out until the last tube, which eventually contained 31.25 μg mL-1. Therefore, the twofold dilution reduced the concentration of the stock solution in the second test tube by a factor of two. In the following test tubes the concentration was further diluted reaching 31.25 μg mL-1 in the fifth tube.
The Shim-pack VP-ODS (150 mm × 4.6 mm, 5 μm) reverse phase column (Shimadzu Co, Kyoto, Japan) was used to separate the samples. Total chromatograms were recorded from 0 to 12.5 min by DAD detector system. The chromatographic peak density values were used to estimate the levels of artemisinin in the samples.
Cell Culture and Cytotoxicity Assays
HeLa cells were obtained from American Type Culture Collection (ATCC, Manassas, VA, USA) and cultured in RPMI-1640 medium supplemented with 25 mM HEPES, 2 mM L-glutamine, 100 U mL-1 penicillin, 100 μg mL-1 streptomycin (all reagents from Sigma-Aldrich Corporation, St. Louis, MO, USA), and 10% heat-inactivated fetal calf serum (FCS; Cultilab, Campinas, Brazil) in a humidified incubator at 37°C and 5% CO2.
The cytotoxicity of A. annua infusion samples and pure artemisinin were evaluated for cell viability with a MTT assay (Mosmann, 1983). The MTT cell proliferation assay is a method to determine the cell number using standard microplate absorbance readers. The reduction of tetrazolium salts is a reliable way to examine cell proliferation. It involves the conversion of the water-soluble yellow tetrazolium MTT (3-(4,5-dimethylthiazol-2-yl)-2,5-diphenyltetrazolium bromide) to the insoluble formazan by metabolically active cells. The resulting intracellular purple formazan is then solubilized, and the concentration determined by optical density at 570 nm.
HeLa cells were cultured in the presence of A. annua infusion (twofold serial dilutions from 10,000 to 156.25 μg mL-1), pure artemisinin (twofold serial dilutions from 400 to 3.125 μg mL-1), or medium alone (control). After 24 h of incubation, the cells were washed and incubated for 4 h with 0.5 mg mL-1. Thiazolyl Blue Tetrazolium Blue (MTT; Sigma-Aldrich Corporation, St Louis, MO, USA). The absorbance of the light emitted by purple formazan was measured at 570 nm using a microtiter plate reader. The results were expressed as percentage of cell viability when compared to controls.
Parasites
Toxoplasma gondii tachyzoites (2F1 RH strain) were propagated in HeLa cells maintained in RPMI-1640 medium supplemented with penicillin, streptomycin and 2% of FCS at 37°C and 5% CO2. The T. gondii 2F1 strain, which constitutively expresses cytoplasmic β-galactosidase, was provided by Dr. Vern Carruthers (University of Michigan, USA).
Infusion of A. annua on T. gondii Infection In vitro
In all experimental and infection conditions, a reference curve was prepared with double serial dilutions of T. gondii tachyzoites of 2F1 (RH) ranging from 1 × 106 to 1.6 × 104 parasites in 100 μL of a medium with 2% of fetal bovine serum (FBS). After 24 h of incubation at 37°C and 5% CO2, the plates were centrifuged for 5 min at 4°C. Next, the supernatant was discarded and the excess of the parasites was removed from the monolayers by washing with cold phosphate buffered saline (PBS, pH 7.2). Then, the cells were lysed with 100 μL of ice-cold lysis buffer (100 mM HEPES, pH 8.0, 1 mM MgSO4, 0.1% Triton X-100, 5 mM dithiothreitol) for 15 min. Next, the lysis buffer was removed and 160 μL per well of assay buffer was added (100 mM phosphate buffer, pH 7.3, 102 mM β-mercaptoethanol, and 9 mM MgCl2) and 40 μL of 3 mM CPRG chlorophenol red-β-D-galactopyranoside (Roche Diagnostics, Indianapolis, IN, USA). The enzyme activity of β-galactosidase was determined after 30 min at room temperature in the dark at 570 nm in a plate reader (Molecular Devices, Palo Alto, CA, USA). Results were expressed as the number of tachyzoites calculated in relation to the reference curve with 2F1 tachyzoites.
In the first set of the experiment (parasites pre-treated before the infection) 2F1 RH tachyzoites were pre-treated for 1 h at 37°C and 5% of CO2 with twofold serial dilutions of A. annua infusion (2500–156 μg mL-1) obtained from: (i) plants without (0 kg ha-1) or with (400 kg ha-1) calcium/magnesium silicate applied to the soil, (ii) pure artemisinin (100–1.25 μg mL-1), or (iii) medium alone (control). Parasites were then added to HeLa cell monolayers at 3:1 ratio (parasite:cell) and incubated for 24 h at 37°C and 5% of CO2. Parasite intracellular proliferation was determined by β-galactosidase colorimetric assay at 570 nm (Jones-Brando et al., 2006).
In the second set of the experiment (cells treated after the infection) HeLa cell monolayers were infected with 2F1 RH tachyzoites at 3:1 (parasite:cell) for 3 h at 37°C and 5% of CO2. After washing, infected cells were treated with different concentrations of A. annua infusion, artemisinin, or medium alone (control) as described above. Parasite proliferation was determined as described for the first experimental condition.
In the third set of the experiments (cells treated immediately after the infection) HeLa cell monolayers were infected with 2F1 RH tachyzoites at 3:1 (parasite:cell) and then immediately treated with different concentrations of A. annua infusion, artemisinin, or medium alone (control) as described above. Parasite proliferation was determined as described for the earlier experiments. Two independent experiments were performed with four replicates for each experimental condition.
Statistical Analysis
All data were expressed as mean ± mean standard error (MSE). Statistical analysis was performed using the GraphPad Prism version 5.0 software (GraphPad Software, Inc., San Diego, CA, USA). The differences in the total trichome density, percentage of intact trichome, trichome mean size, artemisinin contents, and parasite proliferation were determined by ANOVA and Bonferroni multiple comparison test. Significant differences were considered when p < 0.05.
Results
Effects of Si Treatment on A. annua Nutritional Status
The effect of different Si doses applied to the soil on the macronutrient content in A. annua leaves was significant only for nitrogen, which increased with the highest silicate dosage (1600 kg ha-1). The average N content after Si application varied from 19.04 g kg-1 for 0 Si kg ha-1 to 29.48 g kg-1 for the dose of 1600 kg ha-1, an increase by 64.6% relative to the control (data not shown). However, the increased N content induced by the highest silicate dose applied to the soil was neither correlated with plant height (Figure 1A) nor the artemisinin content (Tables 1 and 2). The content of micronutrients was not significantly affected by silicate doses (data not shown). Furthermore, the A. annua plant exhibited high tolerance to soil acidity, with adequate growth even in conditions of low soil fertility, as determined in its initial chemical characterization. In addition, the different Si doses applied to the soil did not significantly affect the Si content in A. annua leaves, which values ranged from 0.3 (control) to 0.4% (1600 kg ha-1; Figure 1B).
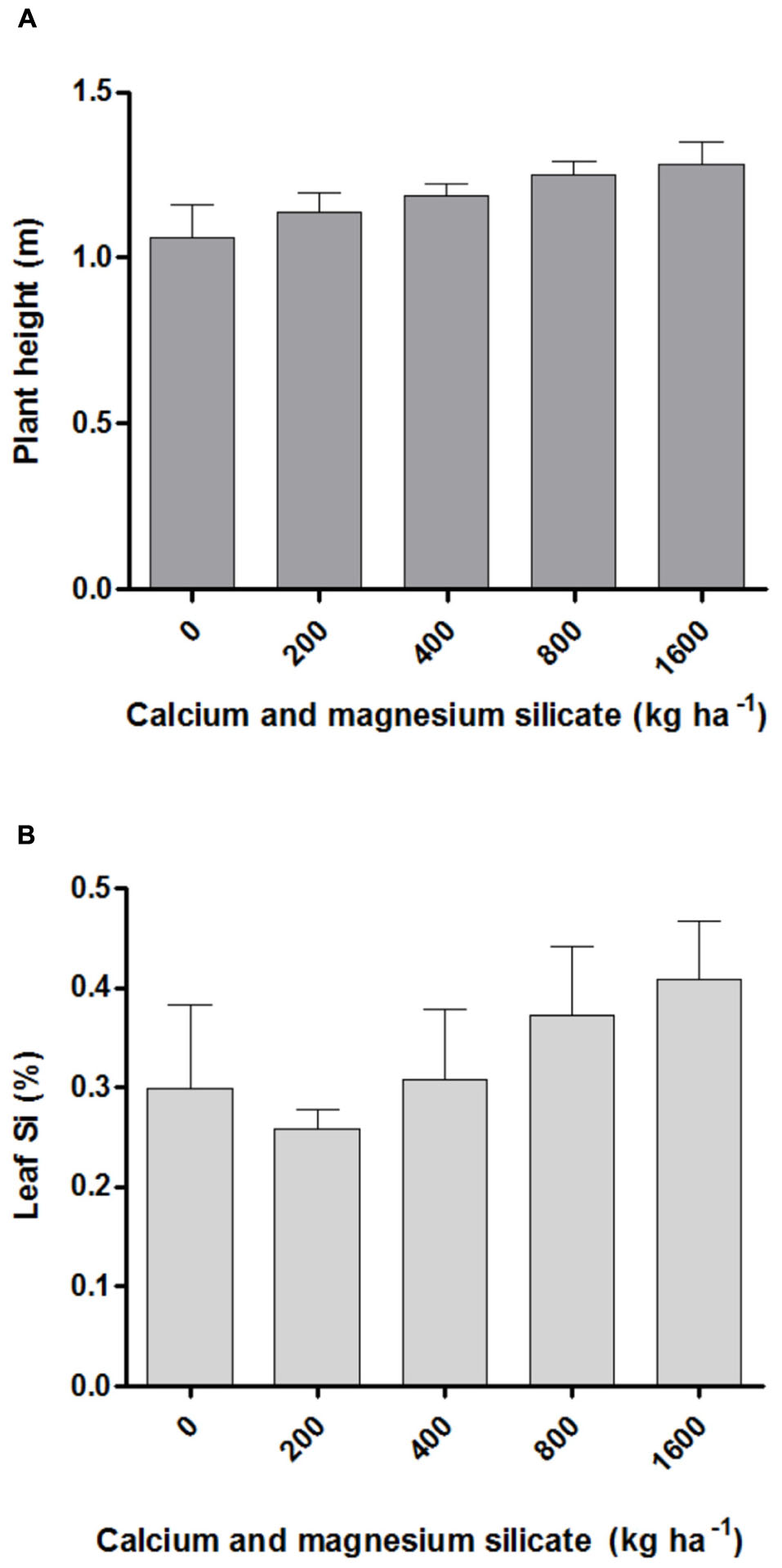
FIGURE 1. Plant height (A) and silicon content in leaves (B) of Artemisia annua after calcium/magnesium silicate application in the soil. Data represent mean ± mean standard error (MSE).
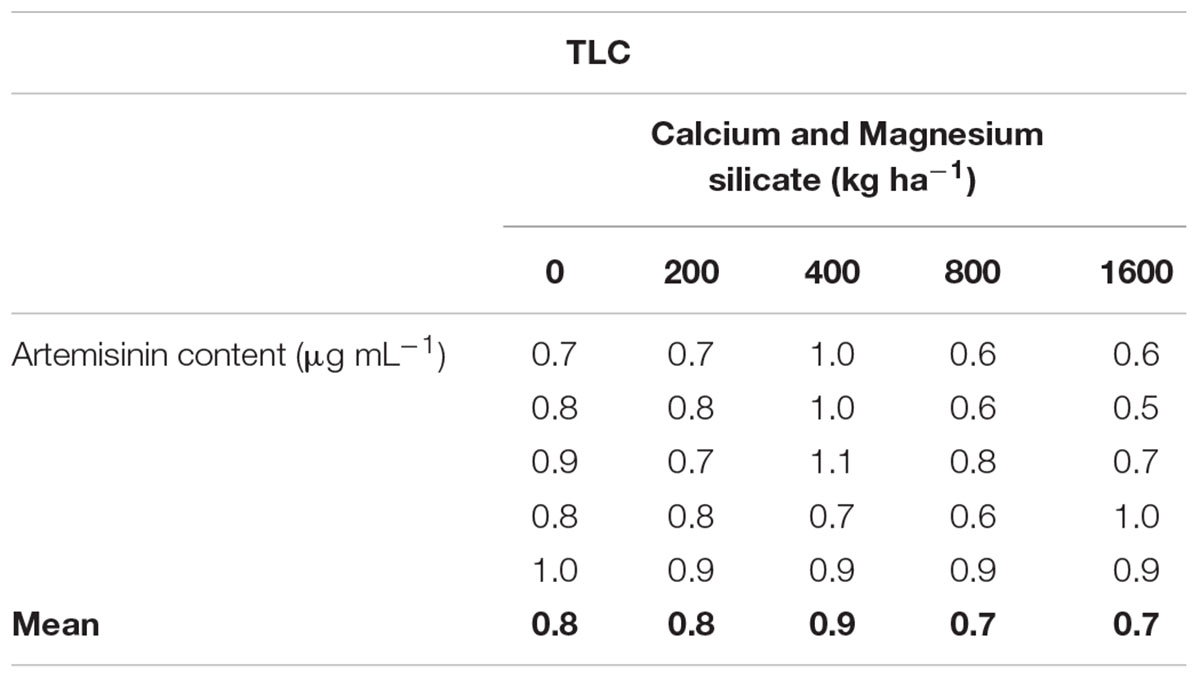
TABLE 1. Artemisinin concentrations, analyzed by TLC (thin layer chromatography), in leaves of Artemisia annua grown in soil treated with Si at doses of 200, 400, 800, or 1600 kg ha-1, in comparison with non-treated soil.
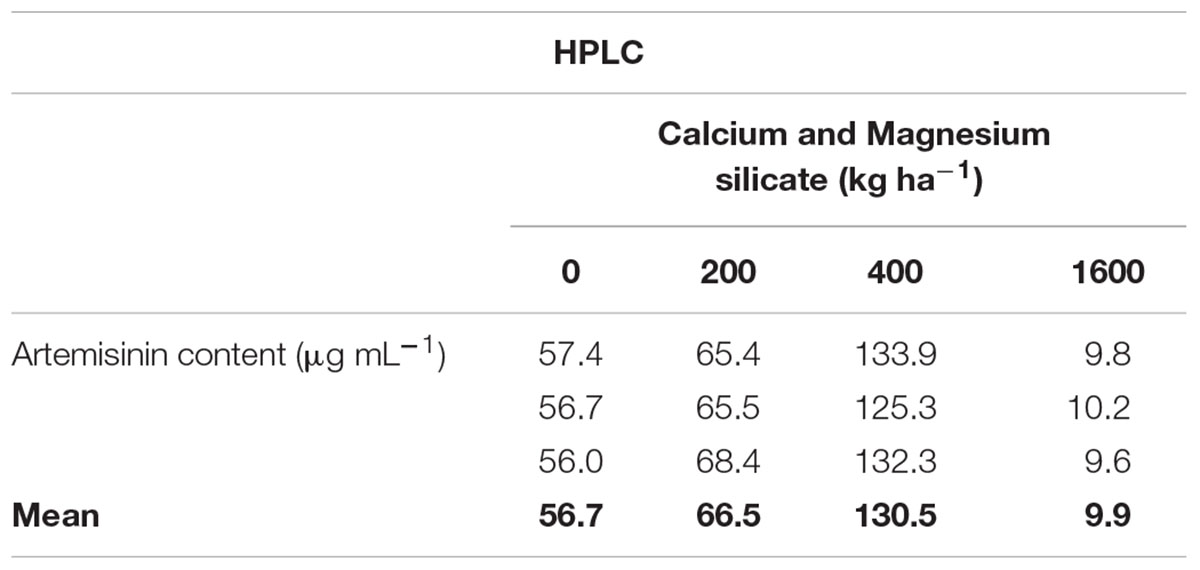
TABLE 2. Artemisinin concentrations, analyzed by HPLC (high performance liquid chromatography), in leaves of A. annua grown in soil treated with Si at doses of 200, 400, or 1600 kg ha-1, in comparison with non-treated soil.
Glandular Trichomes and Artemisinin Concentrations
The density of total glandular trichomes in A. annua did not increase with Si applications, when compared to the control (Figure 2A). Indeed, the soil without Si application revealed density mean value of 28.5 ± 1.2 of total trichomes per mm2; significantly higher than the values from Si-treated soil (∗∗p < 0.01). On the other hand, no significant differences were observed concerning the density of intact trichomes among all tested conditions, i.e., application of 0, 400, and 800 kg ha-1 of Si to the soil. In contrast, the percentages of intact glandular trichomes in A. annua leaves after Si application in the soil was significantly higher for the plants grown with 400 or 800 kg ha-1 of Si, which present around 100% of all intact glandular trichomes (∗p < 0.05; Figure 2B). Furthermore, the Si effects on trichome size were considerably distinct in the 400 and 800 treatments; both increased the sizes of total and intact glandular trichomes, relative to the mean size of total and intact trichomes in the control (Figure 3). These findings can be visualized in Figure 4, showing the disrupted glandular trichomes for plants without the application of silicate and the number of intact trichomes in plants with 200–1600 kg ha-1 of silicate (Figures 4B–E), mostly with 400 kg ha-1 of silicate (Figure 4C). These results indicate that the application of 400 kg ha-1 of silicate, corresponding to 32.5 mg kg-1 of Si, induced the highest density of intact glandular trichomes. These trichomes probably contained more plant metabolites including artemisinin, compared to the opened or disrupted trichomes, which were mostly found in A. annua leaves without the application of silicate.
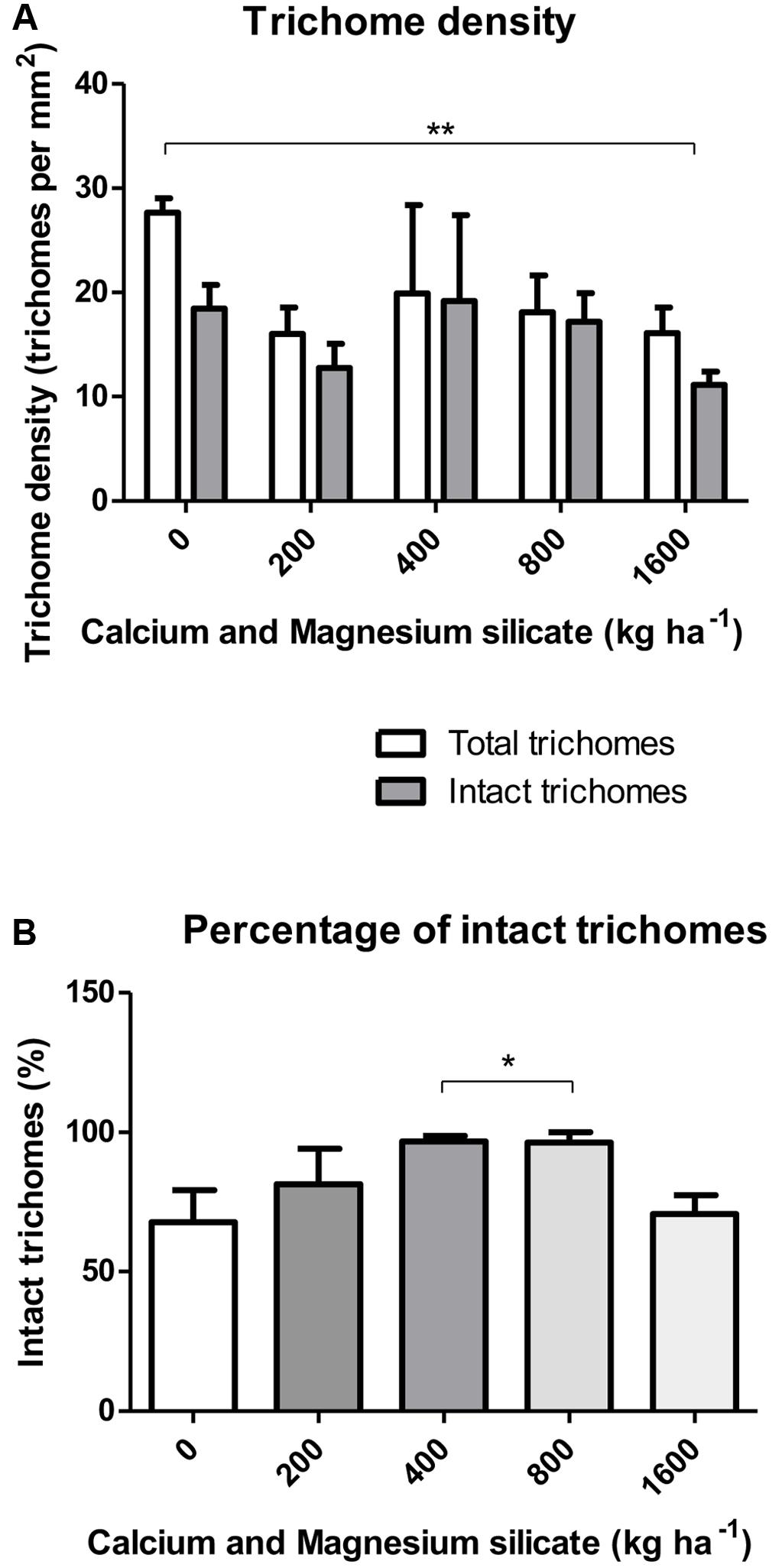
FIGURE 2. (A) Density of total and intact trichomes (B) Percentage of intact glandular trichomes in A. annua leaves after calcium/magnesium silicate application in the soil. Data represent mean ± MSE. ∗p < 0.05; ∗∗p < 0.01.
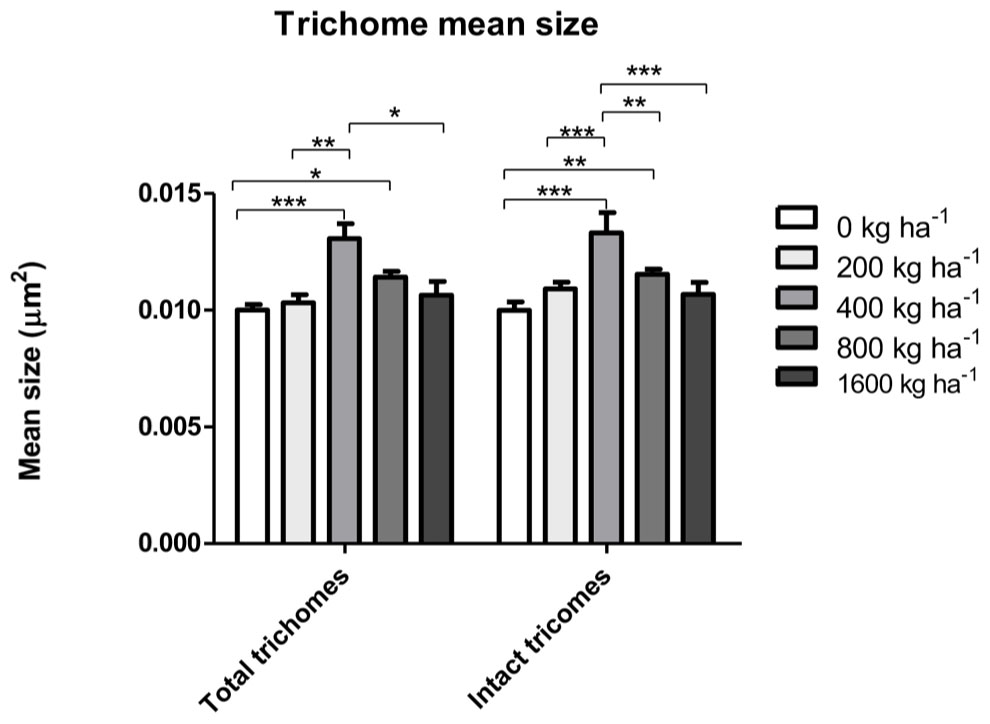
FIGURE 3. Total and intact trichome mean sizes in A. annua leaves with or without calcium/magnesium silicate applications in the soil. Data represent mean ± MSE. ∗p < 0.05; ∗∗p < 0.01; ∗∗∗p < 0.001.
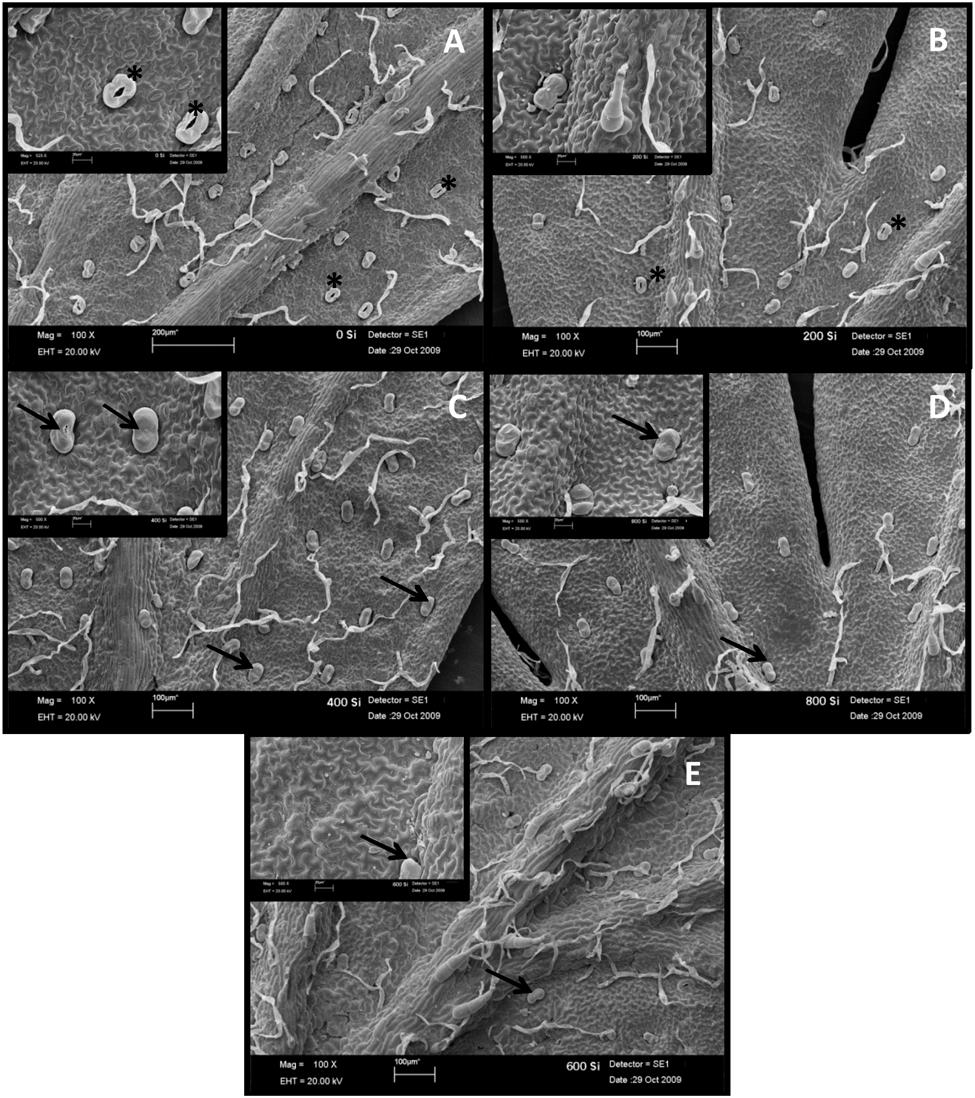
FIGURE 4. Scanning electron microscopy of the leaf abaxial surface from A. annua grown without (A) or with 200 (B), 400 (C), 800 (D), and 1600 kg ha-1 (E) of calcium/magnesium silicate application to the soil. Arrows indicate intact/not disrupted glandular trichomes and asterisks indicate disrupted glandular trichomes.
Similar results were obtained when the artemisinin concentrations in A. annua leaves were analyzed by TLC (Figure 5A; Table 1), showing a tendency of higher artemisinin concentration for 400 kg ha-1 of silicate, which was significantly higher in comparison with the dose of 800 kg ha-1 of silicate. The artemisinin concentration was also evaluated by HPLC in A. annua infusion obtained from plants grown under different doses of silicate (Figure 5B; Table 2). The mean concentration of artemisinin ranged from 9.9 μg mL-1 to 130.5 μg mL-1, except for the samples obtained with the application of 800 kg ha-1 of silicate, in which artemisinin could not be detected. The highest artemisinin concentration (130.5 μg mL-1) was detected for the dose of 400 kg ha-1 of silicate. Chromatograms displaying the peaks of artemisinin (5.7 min) for each plant infusion sample, obtained from the different silicate dosages applied to the soil, are shown in the Supplementary Figure S1.
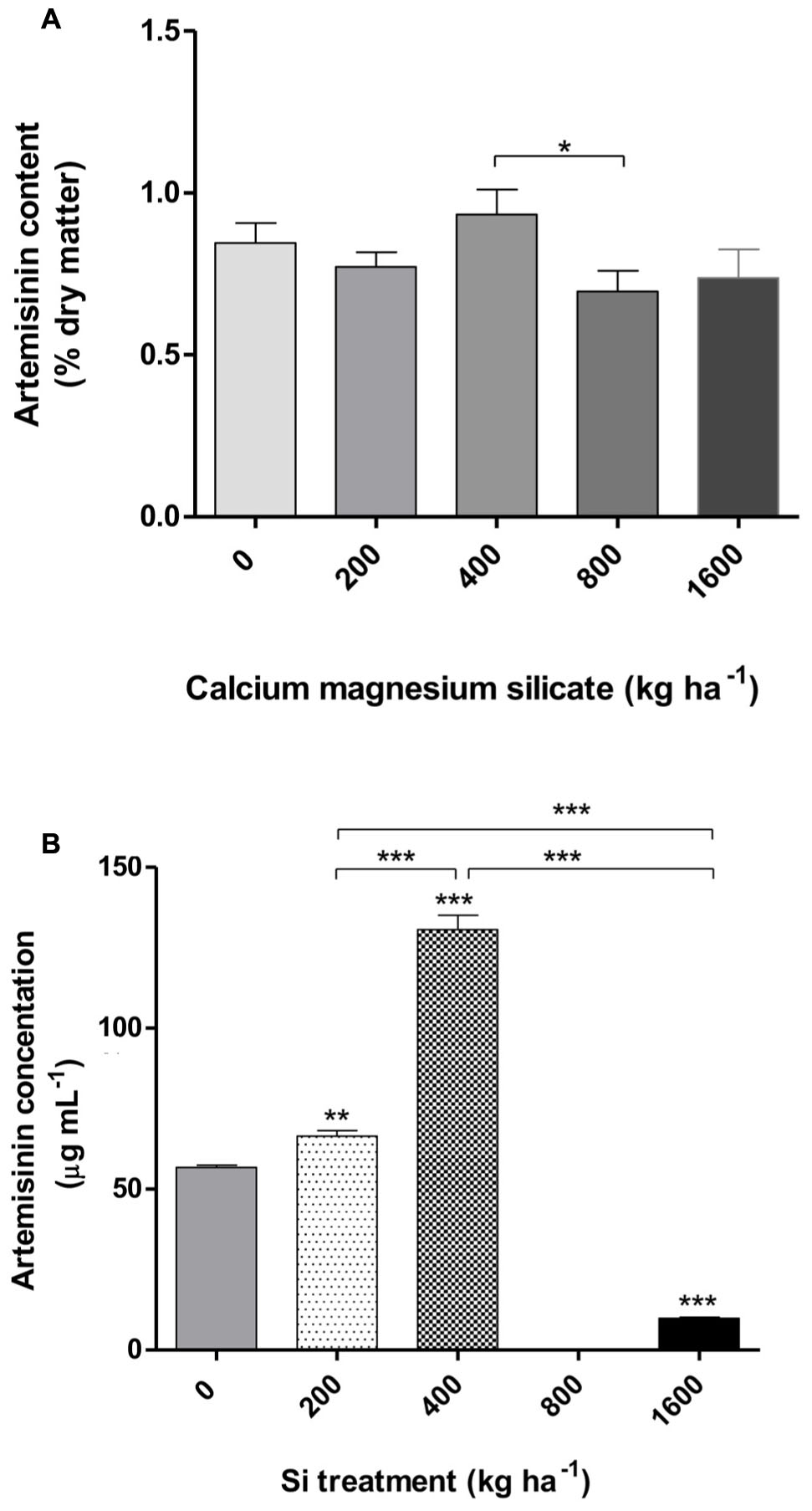
FIGURE 5. Artemisinin content in A. annua leaves determined by TLC (thin layer chromatography) (A) and plant infusion determined by HPLC (high performance liquid chromatography) (B) obtained after calcium/magnesium silicate application to the soil. Data represent mean ± MSE. ∗p < 0.05; ∗∗p < 0.01; ∗∗∗p < 0.001 in comparison with the control (0 kg ha-1 silicate) or as indicated.
Cytotoxicity Assay of A. annua Infusion
The cytotoxicity assay to host cells treated with A. annua infusion samples obtained from the application of 400 kg ha-1 of silicate was selected because this condition showed the highest level of artemisinin concentration. The comparison was carried out for the treatment with infusion samples without silicate application, as well as for pure artemisinin. The following cell viability rates were found: (i) higher than 80% for the plant infusion obtained without silicate application (Figure 6A); (ii) higher than 70% for the infusion obtained with the application of 400 kg ha-1 silicate (Figure 6B); and (iii) higher than 90% for pure artemisinin (Figure 6C) and all analyzed concentrations. The plant infusion concentrations ranging from 156 to 2.500 μg mL-1 were selected for further in vitro experiments of T. gondii infection, resulting in higher than 87% cell viability. As control, the artemisinin concentrations ranging from 1.25 to 100 μg mL-1 were selected, corresponding to higher than 90% cell viability.
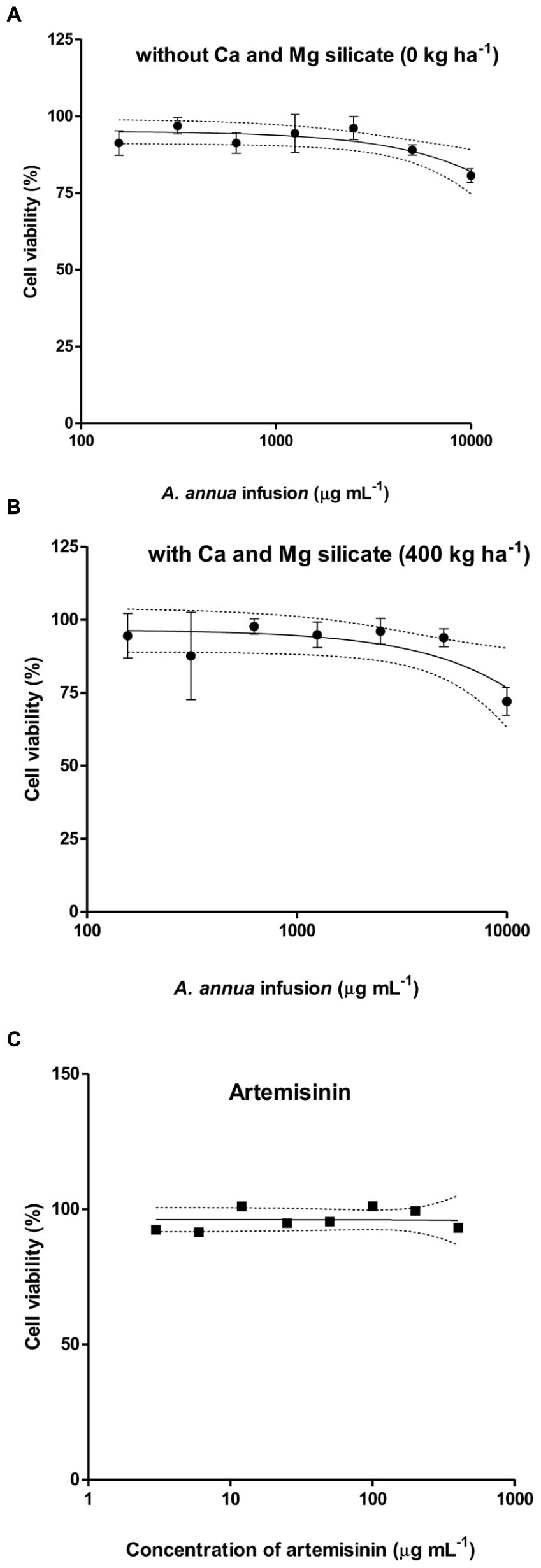
FIGURE 6. Cytotoxicity of A. annua infusion samples obtained without (A) or with 400 kg ha-1 (B) calcium/magnesium silicate applied to the soil, comparatively to pure artemisinin (C), determined by MTT assays. The results are expressed as percentage of viable cells compared to control.
Effect of Treatment with A. annua Infusion on T. gondii Growth
The effects of A. annua infusion samples obtained without or with 400 kg ha-1 of silicate, compared to pure artemisinin, on T. gondii proliferation were evaluated under three experimental conditions (Figure 7). Firstly, we analyzed the effects of the infusion treatment on the parasite before host cell infection (Figure 7A). The treatment with plant infusion without silicate application was able to decrease the parasite proliferation only in the highest concentration (2,500 μg mL-1), when compared to infected and untreated controls. In contrast, the treatment with plant infusion obtained from the application of 400 kg ha-1 of silicate was unable to change the parasite growth at any analyzed concentrations. The treatment with pure artemisinin decreased parasite proliferation at all examined concentrations, although without a dose-dependent response. Secondly, the effects of artemisinin and infusion treatments on cells already infected with T. gondii were analyzed (Figure 7B). In this case, the results showed that the parasite proliferation was reduced in a dose-dependent manner for both infusions, with and without silicate application, by the concentrations of 312 and 625 μg mL-1, respectively, when compared to the infected and untreated controls. Again, artemisinin treatment decreased the parasite growth, although without a dose-dependent response. Finally, the effects of artemisinin and infusion treatments on cell along with the infection were investigated (Figure 7C). Here, all the concentrations of the plant infusion obtained without silicate application were able to decrease parasite replication compared to the infected and untreated controls, but with a dose-dependent response from 312 μg mL-1 onward. For the plant infusion obtained from 400 kg ha-1 of silicate application, there was a dose-dependent reduction from 625 μg mL-1 onward. Artemisinin also decreased the parasite growth in a dose-dependent manner for all analyzed concentrations.
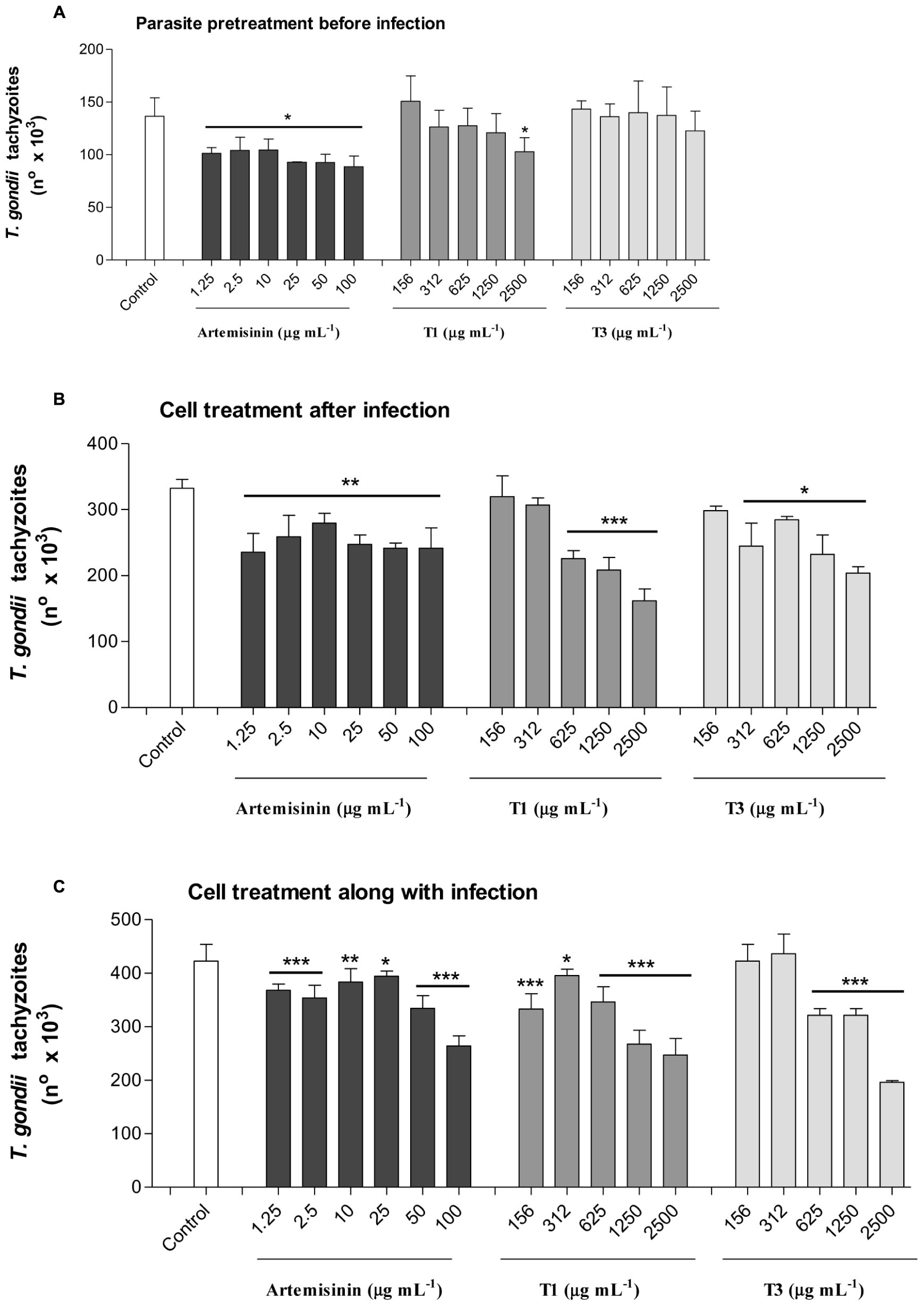
FIGURE 7. Toxoplasma gondii proliferation evaluated by a β-galactosidase colorimetric assay. (A) Pretreatment of parasites with A. annua infusion samples or pure artemisinin before infection; (B) Treatment of host cells with A. annua infusion samples or pure artemisinin after infection; (C) Treatment of host cells with A. annua infusion samples or pure artemisinin simultaneously to the infection. Two-fold serial dilutions of A. annua infusion (2500 to 156 μg mL-1) obtained from plants without (T1) or with (T3) calcium/magnesium silicate applied to the soil (400 kg ha-1), compared to pure artemisinin (100 to 1.25 μg mL-1) or medium alone (control). Data are expressed as mean ± MSE of the number of tachyzoites calculated in relation to a reference curve. ∗p < 0.05; ∗∗p < 0.01; ∗∗∗p < 0.001.
Discussion
Silicon is an abundant soil element but not all Si forms present in the soil are available for plant uptake. Usually, Si is found as silicate minerals, aluminum silicates, and various forms of silicon dioxide (SiO2). Used as a plant nutrient, Si fertilization improves crop productivity and plant health (Guntzer et al., 2012; Haynes, 2014).
Plants absorb Si in the form of monosilicic acid (H4SiO4), which is found in both liquid and adsorbed phases of Si in soils. The monosilicic acid behaves as a very weak acid, and even in pH 7.0, only 2 mg kg-1 are ionized (H3SiO4-); as pH increases the degree of ionization increases as well. Its concentration in soil solution varies according to clay content, iron and aluminum oxides or hydroxides, organic matter, and pH. Soil pH can be corrected with liming materials, modifying the solubility of the monosilicic acid. This fact is important for rice, once maximum silicon absorption occurs within the 4.7–7.6 pH range (Guntzer et al., 2012; Tubaña and Heckman, 2015).
Some soils as Oxisols (used in the research) and Ultisols demonstrate low levels of Si available to the plants in their native state. These soils are leached, acid, highly weathered, and low in base saturation (Foy, 1992). Thus, Ca and Mg silicate fertilization can improve fertility of such soils. Si can enhance the growth and development of several plant species including rice, sugarcane, most other cereals, and several dicotyledons (Jones and Handreck, 1967; Elawad and Green, 1979; Bélanger et al., 1995; Savant et al., 1997). In addition, Si amendments proved effective in controlling several important plant diseases. Nevertheless, in this research work soil amended with different Si doses demonstrated significant positive correlation only with N at the dose of 1600 kg ha-1(Table 3). There appeared to be a silicon-induced increase in leaf nitrogen concentration, but statistically significant only at the highest dose. In this study, urea and soil organic matter were the possible nitrogen sources. Therefore, this correlation remains to be explored. Nitrogen is an important component of amino acids, proteins, nucleic acids, and many other compounds. Other foliar macronutrients were not significantly affected by Si treatments. The role of macronutrients in A. annua leaves have already been investigated by Ferreira (2007) who showed that the nutrient least required for biomass accumulation per plant was K, followed by P, and N, and that the artemisinin concentration was significantly higher in -K plants when compared to plants under the complete treatment.
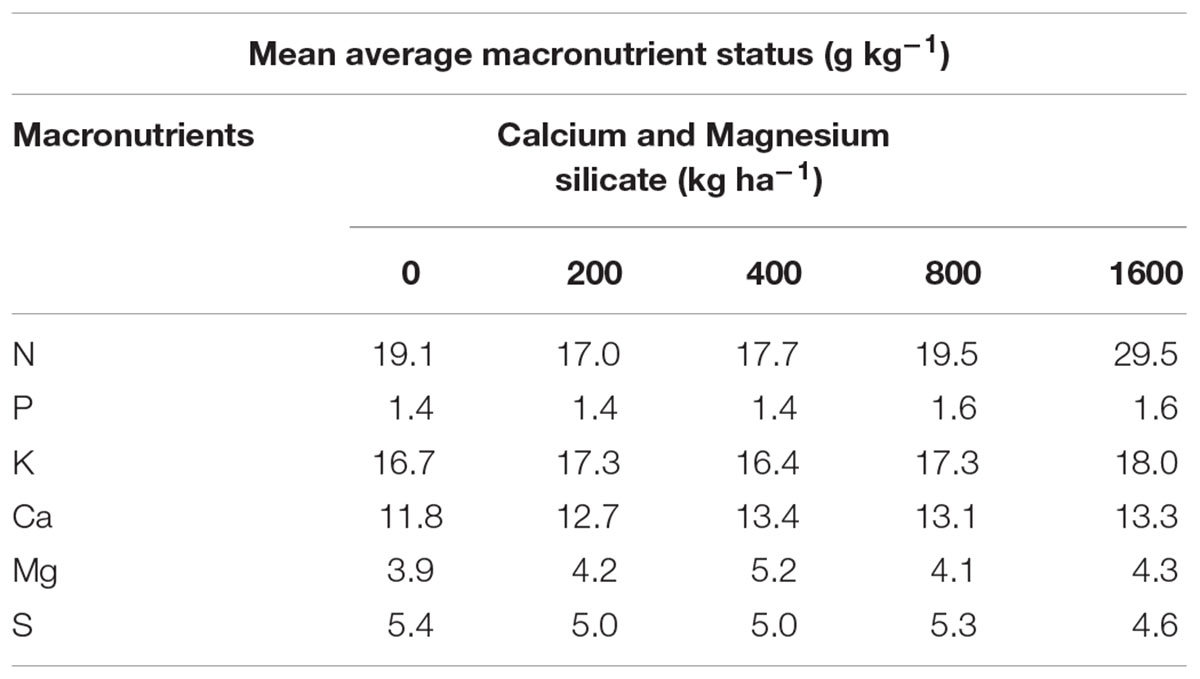
TABLE 3. Macronutients present in the leaves of A. annua grown in soil treated with Si at doses of 0, 200, 400, 800, or 1600 kg ha-1.
Although statistically insignificant, Si treatments increased the average foliar Si content by 0.1%, from 0.3% for 0 kg ha-1 to 0.4% for 1600 kg ha-1. According to criteria established by Ma and Takahashi (2002), plants with a Si content and Si/Ca ratio higher than 1.0% and 1.0, respectively, are defined as silicon accumulators. By contrast, plants with a Si content lower than 0.5% and Si/Ca ratio lower than 0.5 are defined as Si non-accumulating plants. For monocotyledons species, the Si content is high and the Ca content is low. For dicotyledon species, occurs the opposite. In this study, the Si/Ca ratio was 0.25 and 0.3 for 0 kg ha-1 and 1600 kg ha-1 treatments, respectively. Further, according to Mitani and Ma (2005) the low accumulation of Si in dicots is due to a lack of specific transporters to facilitate the radial transport and the xylem loading of Si and suggested that the transport of silicon across cells was accomplished via a passive diffusion mechanism. Thus, the analysis of foliar mineral composition indicates that A. annua is a Si non-accumulating plant and does not have an active Si-uptake mechanism. Still, Si application in our experiment was positively correlated with artemisinin content and trichome integrity perhaps due to the fact that the initial soil demonstrated low pH and low Si content.
Micronutrient status was also not significantly altered by any silicate doses applied to the soil (Table 4). Therefore, suggesting that A. annua plants had sufficient amounts of micronutrients for the growth and production of artemisinin. Previous studies evaluating the micronutrient content in A. annua, demonstrated that the amount of artemisinin decreased in the absence of copper (Cu) and especially of boron (B; Srivastava and Sharma, 1990). Moreover, in another research work, B-deficient plants did not bloom, leading to a reduction of artemisinin content by 50% (Brisibe et al., 2008).
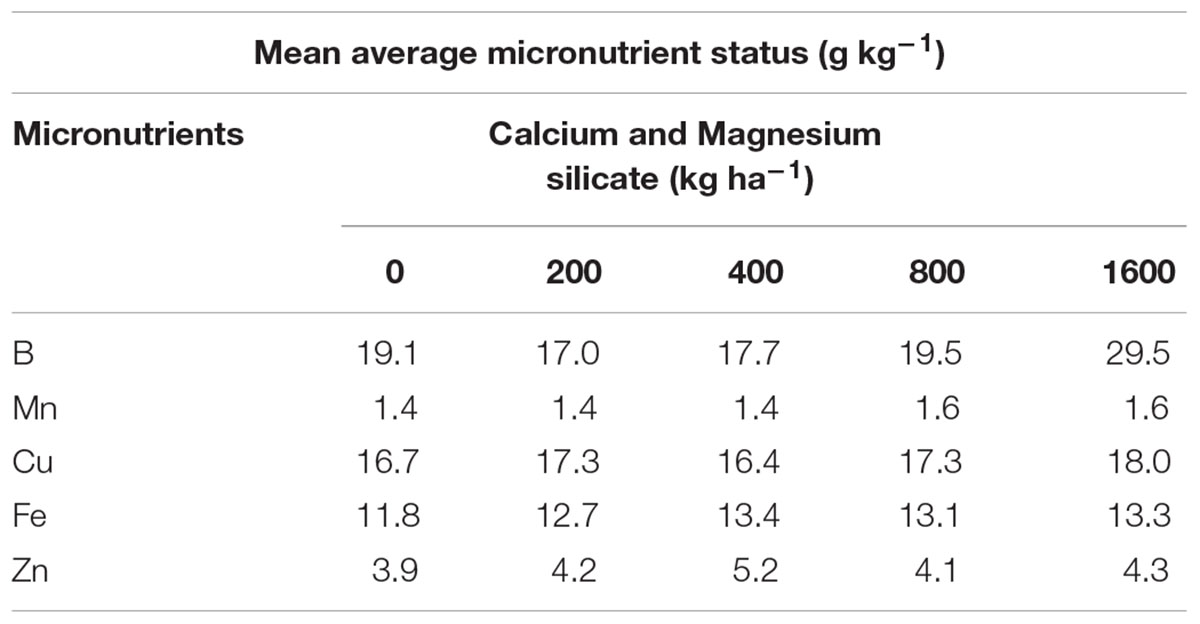
TABLE 4. Micronutrients present in the leaves of A. annua grown in soil treated with Si at doses of 0, 200, 400, 800, or 1600 kg ha-1.
The effects of Si on plant growth and productivity have already been investigated (Datnoff et al., 2001; Cooke and DeGabriel, 2016 and Coskun et al., 2016; Reynolds et al., 2016), but to date there is no information regarding Si effect on A. annua and the development the glandular trichome. Further, there is limited data regarding the use of A. annua infusion on T. gondii infection. In the present study, we investigated the response A. annua to Si signal, considering the content of the active principle, the artemisinin, in the inhibition of T. gondii infection. Four different Si doses and three different infusion assays were assessed. The 400 and 800 kg ha-1 Si doses notably increased the mean size of the intact glandular trichomes.
Artemisinin is stored in glandular trichomes in the aerial parts of A. annua (Duke and Paul, 1993). Trichomes had already been identified by SEM and X-ray microanalysis, showing the deposition of Si in the cell wall of wheat leaves and the location of silica acting as a physical barrier (Epstein, 2009). In this study, the effects of Si on the artemisinin content were investigated by analysis of trichomes and it was found that, even though the mean density of total glandular trichomes was higher without Si application, the percentages of intact glandular trichomes in A. annua leaves after Si applications to the soil was significantly higher for the plants grown with 400 or 800 kg ha-1 Si, which present about 100% of all intact glandular trichomes.
Artemisinin solubility was not appropriate for the analysis of the samples by TLC in the present study, since the artemisinin concentration was determined on dried leaves of A. annua and expressed as percentage of dry weight. Also, samples obtained from the treatment with 800 kg ha-1 of silicate showed lower artemisinin concentration compared to the treatment with400 kg ha-1 of silicate in the soil. However, using the HPLC it was possible to detect high concentrations of artemisinin in A. annua infusions, even in the control condition, when the plant was cultivated without the application of silicate (56.7 μg mL-1 of artemisinin). These results are higher than those found by Mueller et al. (2000) (24.5 μg mL-1) and Jansen (2006) (24.2 μg mL-1), using similar infusion preparations and analysis methods. Furthermore, the dose of 400 kg ha-1 of silicate induced higher concentrations of artemisinin than those previously reported in plants without the application of silicate (94.0 μg mL-1; Mueller et al., 2004; Räth et al., 2004). Clearly, these results show wide variation in the artemisinin content in A. annua infusion samples, indicating that many factors, such as temperature, contact time, percentage of material, solvent, and plant genetics can influence the solubility of artemisinin and its subsequent therapeutic effects (van der Kooy and Verpoorte, 2011; Rocha e Silva et al., 2012). In this context, it is likely that the unexpected very low concentrations of artemisinin in infusion samples, which were obtained from A. annua treated with 1600 kg ha-1 and, especially with 800 kg ha-1 of silicate, may have been affected by some of these factors, which may impact the solubility of artemisinin, resulting in almost undetectable levels.
To date, the infusion of A. annua is not considered as an option for the treatment of toxoplasmosis. Currently, conventional drugs, as sulfadiazine/pyrimethamine, are the first choice for treatment of the clinical presentations of toxoplasmosis (Petersen and Schmidt, 2003). Nevertheless, due to the severe side effects, including bone marrow suppression, this conventional procedure demands the concomitant administration of folinic acid to alleviate these effects (Martins-Duarte et al., 2006). Furthermore, this treatment is frequently not well tolerated, leading to the substitution of sulfadiazine by other drugs such as clindamycin (Montoya and Liesenfeld, 2004). These major concerns have stimulated the research toward an alternative and less toxic drug against T. gondii infection.
Although A. annua infusion is not commonly used to control toxoplasmosis, as it is for malaria, in the present study the effect on T. gondii intracellular proliferation was tested as an alternative therapy, since its ability to decrease the tachyzoite replication may be useful in the treatment of acute primary toxoplasmosis or reactivation of chronic infection. However, even though multiple phenolic constituents, as chlorogenic acids, play a role in enhancing artemisinin solubility and extractability in A. annua aqueous preparations (Carbonara et al., 2012), the low solubility of artemisinin when compared to pure artemisinin, which is soluble in distilled water and demonstrates fair stability in neutral solvents, still handicaps the usefulness of A. annua therapeutic infusion.
Considering all the results obtained from the A. annua infusion samples in the present study, there was a decrease in parasite proliferation achieved with lower doses of plant infusions obtained from the application of silicate. Still, the infusion obtained from Si treatments was as effective as the infusion obtained from –Si treatments, even though the concentration of artemisinin was higher in A. annua treated with 400 kg ha-1 of silicate. On the other hand, parasite pretreatment with infusion samples before infection was less effective in controlling parasite proliferation for both samples, with or without silicate application. As expected, the pure artemisinin was able to decrease parasite proliferation in all experimental conditions, as previously reported.
Jones-Brando et al. (2006) and Hencken et al. (2010) investigated four artemisinin derivatives, which showed an increase in anti-Toxoplasma activity and decreased cytotoxicity as compared to trimethoprim. Unsaturated synthetic derivatives, known as carba artemisinin, were also able to inhibit various stages of T. gondii, emerging as therapeutic agents for the prevention and treatment of toxoplasmosis in humans (D’Angelo et al., 2009).
In malaria parasite, the artemisinin triggers the inhibition of calcium-ATPase of the endoplasmic reticulum sarcoplasm (SERCA; Woodrow et al., 2005). Nagamune et al. (2007) evaluated chemically derived mutants of T. gondii resistant to artemisinin and concluded that the resistance was not due to molecular changes in the SERCA expression, but in the induction of microneme secretion, a calcium-dependent process that is triggered by artemisinin. These studies suggest a calcium homeostasis in the mechanism of action of artemisinin against parasites from Apicomplexa phylum. In the present study, however, it was observed no variation in Ca content in leaves treated with any Si concentration, when compared with untreated plant, excluding the role of this macronutrient in the results obtained in our experimental design.
The use of A. annua infusion in T. gondii infection was demonstrated for the first time in our previous study, showing that the plant infusion can be a potential therapeutic alternative to control the growth of the parasite in both in vitro and in vivo experimental conditions (Oliveira et al., 2009). In the present study, the application of silicate in the soil had a positive effect on the size of glandular trichomes and hence on the artemisinin content, but this result was not associated with enhanced efficacy of leaf infusion from A. annua grown in the soil with silicate application on T. gondii replication. These data suggest that other components in addition to artemisinin could contribute to the effect of the plant infusion. In this context, it is known that A. annua infusion is rich in phenolic antioxidants, especially flavonoids (Räth et al., 2004). As the artemisinin content in A. annua leaves is approximately 1%, it is questionable whether all the benefits of the traditional tea are uniquely assigned to artemisinin (Ferreira et al., 2010). Among the advantages of using the A. annua infusion, there is the possibility of producing and preparing plant medication without the need for purification, which causes the loss of other valuable compounds from plants, such as flavonoids, flavones, and coumarins.
Conclusion
The application of Si to the soil at concentration of 400 kg ha-1 had beneficial effects on the A. annua physiology, such as the size of total and intact glandular trichomes, which increased the capability of storage and content of artemisinin in A. annua leaves and infusion. The opposite effect, however, was observed when higher concentrations of Si, i.e., 800 or 1600 kg ha-1 of silicate were applied to the soil, resulting in almost undetectable levels of artemisinin in the A. annua infusion. Thus, further studies are necessary to investigate the extractability of artemisinin from leaves containing high-size trichomes. Also, considering that the Si treatment in the soil had little influence on the effect of A. annua infusion to control T. gondii growth, since both types of infusions (with or without the application of silicate) were able to reduce parasite proliferation, other components present in the leaves may be acting in synergy with the artemisinin, and should be investigated.
Author Contributions
CR, CM, and TO carried out the majority of the experiments with A. annua, from the plant cultivation to the extract assays in cell cultures. FS carried out the experiments related to the parasite maintenance in cell culture and the HPLC analysis. LO, GK, and RL performed the experiments concerning the analysis of macro and micronutrients, as well as the Si content in A. annua. MR and NN participated in the surface analysis of the glandular trichomes in A. annua leaves by scanning electron microscopy. XS performed the experiments to determine the artemisinin content in A. annua leaves by thin layer chromatography. CR, TM, DS, and JM participated in the experimental design throughout this research, as well as in the data analysis and revision of the manuscript.
Funding
This work was supported by Brazilian Research Agencies – FAPEMIG (Proc. #APQ-01313-14), CNPq (Proc. # 311787/2013-4 and 456650/2013-0), and CAPES (Proc. # AUXPE-02450/09-7).
Conflict of Interest Statement
The authors declare that the research was conducted in the absence of any commercial or financial relationships that could be construed as a potential conflict of interest.
Acknowledgments
We thank Dr. Pedro Melillo de Magalhães (Center of Chemical, Biological, and Agricultural Researches, Universidade Estadual de Campinas, Brazil), Dr. Carlos Machado dos Santos (Universidade Federal de Uberlândia, Brazil), Dr. Vern Carruthers (University of Michigan Medical School, USA), Dr. Elliot Watanabe Kitajima (NAP/MEPA/ESALQ/USP), and MSc.Tomasz Rostkowski (Warsaw University of Life Sciences – SGGW, Poland).
Supplementary Material
The Supplementary Material for this article can be found online at: http://journal.frontiersin.org/article/10.3389/fpls.2016.01430
FIGURE S1 | High performance liquid chromatography (HPLC) chromatograms determined for Artemisia annua infusion samples obtained after calcium/magnesium silicate application to the soil at different concentrations, as follow: (A) 0 kg ha-1; (B) 200 kg ha-1; (C) 400 kg ha-1; (D) 800 kg ha-1; (E) 1600 kg ha-1. Arrows indicate the peak of artemisinin (5.7 min) according to the reference curve.
References
Bélanger, R. R., Bowen, P. A., Ehret, D. L., and Menzies, J. G. (1995). Soluble silicon: its role in crop and disease management of greenhouse crops. Plant Dis. 79, 329–336. doi: 10.1094/PD-79-0329
Boothroyd, J. C., and Grigg, M. E. (2002). Population biology of Toxoplasma gondii and its relevance to human infection: do different strains cause different disease? Curr. Opin. Microbiol. 5, 438–442. doi: 10.1016/S1369-5274(02)00349-1
Brisibe, E. A., Uyoh, E. A., Brisibe, F., Magalhães, P. M., and Ferreira, J. F. S. (2008). Review: building a golden triangle for the production and use of artemisinin derivatives against falciparum malaria in Africa. Afr. J. Biotechnol. 7, 4884–4896.
Bryant, L., Patole, C., and Cramer, R. (2016). Proteomic analysis of the medicinal plant Artemisia annua: data from leaf and trichome extracts. Data Brief 23, 325–331. doi: 10.1016/j.dib.2016.02.038
Carbonara, T., Pascale, R., Argentieri, M. P., Papadia, P., Fanizzi, F. P., Villanova, L., et al. (2012). Phytochemical analysis of an herbal tea from Artemisia annua L. J. Pharm. Biomed. Anal. 25, 79–86. doi: 10.1016/j.jpba.2012.01.015
Cooke, J., and DeGabriel, J. L. (2016). Editorial: plant silicon interactions between organisms and the implications for ecosystems. Front. Plant Sci. 7:1001. doi: 10.3389/fpls.2016.01001
Cornelissen, J. H. C., Lavorel, S., Garnier, E., Diaz, S., Buchmann, N., Gurvich, D. E., et al. (2003). A handbook of protocols for standardized and easy measurement of plant functional traits worldwide. Aust. J. Bot. 51, 335–380. doi: 10.1071/BT02124
Coskun, D., Britto, D. T., Huynh, W. Q., and Kronzucker, H. J. (2016). The role of Silicon in higher plants under salinity and drought stress. Front. Plant Sci. 7:1072. doi: 10.3389/fpls.2016.01072
D’Angelo, J. G., Bordón, C., Posner, G. H., Yolken, R., and Jones-Brando, L. (2009). Artemisinin derivatives inhibit Toxoplasma gondii in vitro at multiple steps in the lytic cycle. J. Antimicrob. Chemother. 63, 146–150. doi: 10.1093/jac/dkn451
Datnoff, L. E., Seebold, K. W., and Correa-V, F. J. (2001). “The use of silicon for integrated disease management: reducing fungicide applications and enhancing host plant resistance,” in Silicon in Agriculture, eds L. E. Datnoff, G. H. Snyder, and G. H. Korndörfer (Amsterdam: Elsevier Science), 171–184.
Derda, M., Hadaś, E., Cholewiński, M., Skrzypczak,Ł., Grzondziel, A., and Wojtkowiak-Giera, A. (2016). Artemisia annua L. as a plant with potential use in the treatment of acanthamoebiasis. Parasitol. Res. 115, 1635–1639. doi: 10.1007/s00436-016-4902-z
Duke, S. O., and Paul, R. N. (1993). Development and fine structure of the glandular trichomes of Artemisia annua L. Int. J. Plant Sci. 154, 107–118. doi: 10.1086/297096
Elawad, S. H., and Green, V. E. Jr. (1979). Silicon and the rice plant environment: a review of recent research. Riv. Riso 28, 235–253. doi: 10.1111/j.1469-8137.2008.02716.x
Elliott, C. L., and Snyder, G. H. (1991). Autoclave-induced digestion for the colorimetric determination of silicon in rice straw. J. Agric. Food Chem. 39, 1118–1119. doi: 10.1021/jf00006a024
Embrapa, Centro Nacional de Pesquisa de Solos (2009). “Manual de métodos de análises químicas de solos, plantas e fertilizantes,” in Comunicação Para Transferência de Tecnologia, ed. F. C. Silva (Brasília: Embrapa), 370.
Epstein, E. (2009). Silicon: its manifold roles in plants. Ann. Appl. Biol. 155, 155–160. doi: 10.1111/j.1744-7348.2009.00343.x
Ferreira, J. F. S. (2007). Nutrient deficiency in the production of artemisinin, dihydroartemisinic acid, and artemisinic acid in Artemisia annua L. J. Agric. Food Chem. 55, 1686–1694. doi: 10.1021/jf063017v
Ferreira, J. F. S., Luthria, D. L., Sasaki, T., and Heyerick, A. (2010). Review: flavonoids from Artemisia annua L. as antioxidants and their potential synergism with artemisinin against malaria and cancer. Molecules 15, 3135–3170. doi: 10.3390/molecules15053135
Foy, C. D. (1992). Soil chemical factors limiting plant root growth. Adv. Soil Sci. 19, 97–149. doi: 10.1007/978-1-4612-2894-3_5
Golami, S., Rahimi-Esboei, B., Mousavi, P., Marhaba, Z., Youssefi, M. R., and Rahimi, M. T. (2016). Survey on efficacy of chloroformic extract of Artemisia annua against Giardia lamblia trophozoite and cyst in vitro. J. Parasit. Dis. 40, 88–92. doi: 10.1007/s12639-014-0453-3
Guntzer, F., Keller, C., and Meunier, J. (2012). Benefits of plant silicon for crops: a review. Agron. Sustain. Dev. 32, 201–213. doi: 10.1007/s13593-011-0039-8
Haverkos, H. W. (1987). Assessment of therapy for Toxoplasma encephalitis. The TE Study Group. Am. J. Med. 82, 907–914.
Haynes, R. J. (2014). A Contemporary Overview of Silicon Availability in Agricultural Soils. J. Plant Nutr. Soil Sci. 177, 831–844.
Hencken, C. P., Jones-Brando, L., Bordón, C., Stohler, R., Mott, B. T., Yolken, R., et al. (2010). Thiazole, oxadiazole, and carboxamide derivatives of artemisinin are highly selective and potent inhibitors of Toxoplasma gondii. J. Med. Chem. 53, 3594–3601. doi: 10.1021/jm901857d
Jansen, F. H. (2006). The herbal tea approach for artemisinin as a therapy for malaria? Trans. R. Soc. Trop. Med. Hyg. 100, 285–286. doi: 10.1016/j.trstmh.2005.08.004
Jones, J. H., and Handreck, K. A. (1967). Silica in soils, plants, and animals. Adv. Agron. 19, 107–149. doi: 10.1016/S0065-2113(08)60734-8
Jones-Brando, L., D’Angelo, J., Posner, G. H., and Yolken, R. (2006). In vitro inhibition of Toxoplasma gondii by four new derivatives of artemisinin. Antimicrob. Agents Chemother. 50, 4206–4208. doi: 10.1128/AAC.00793-06
Karnovsky, M. J. (1965). A formaldehyde-glutaraldehyde fixative of high osmolality for use in electron microscopy. J. Cell Biol. 27, 137–138.
Kiani, B. H., Suberu, J., and Mirza, B. (2016). Cellular engineering of Artemisia annua and Artemisia dubia with the rol ABC genes for enhanced production of potent anti-malarial drug artemisinin. Malar. J. 15, 252. doi: 10.1186/s12936-016-1312-8
Kitajima, E. W., and Leite, B. (1999). Curso Introdutório de Microscopia Eletrônica de Varredura. Núcleo de Apoio à Pesquisa em Microscopia Eletrônica Aplicada à Pesquisa Agropecuária. Piracicaba: ESALQ-USP.
Korndörfer, G. H., Pereira, H. S., and Nola, A. (2004). Análise de Silício: Solo, Planta e Fertilizante. Uberlândia: GPSi-ICIAG-UFU. Boletim Técnico 2, 34.
Leport, C., Raffi, F., Matheron, S., Katlama, C., Regnier, B., Saimot, A. G., et al. (1988). Treatment of central nervous system toxoplasmosis with pyrimethamine/sulfadiazine combination in 35 patients with the acquired immunodeficiency syndrome. Efficacy of long term continuous therapy. Am. J. Med. 84, 94–100. doi: 10.1016/0002-9343(88)90014-9
Ma, J. F., and Takahashi, E. (2002). Soil, Fertilizer, and Plant Silicon Research in Japan. Amsterdam: Elsevier Science.
Magalhães, P. M., Delabays, N., and Sartoratto, A. (1999). New hybrid lines of antimalarial species Artemisia annua L. Acta Hortic. 502, 377–381. doi: 10.17660/ActaHortic.1999.502.62
Martins-Duarte, E. S., Urbina, J. A., de Souza, W., and Vommaro, R. C. (2006). Antiproliferative activities of two novel quinuclidine inhibitors against Toxoplasma gondii tachyzoites in vitro. J. Antimicrob. Chemother. 58, 59–65. doi: 10.1093/jac/dkl180
Miller, L. H., and Su, X. (2011). Artemisinin: discovery from the Chinese herbal garden. Cell 146, 855–856. doi: 10.1016/j.cell.2011.08.024
Mitani, N., and Ma, J. F. (2005). Uptake system of silicon in different plant species. J. Exp. Bot. 56, 1255–1261. doi: 10.1093/jxb/eri121
Montoya, J. G., and Liesenfeld, O. (2004). Toxoplasmosis. Lancet 363, 1965–1976. doi: 10.1016/S0140-6736(04)16412-X
Mosmann, T. (1983). Rapid colorimetric assay for cellular growth and survival: application to proliferation and cytoxicity assays. J. Immunol. Methods 65, 55–63. doi: 10.1016/0022-1759(83)90303-4
Mueller, M. S., Karhagomba, I. B., Hirt, H. M., and Wemakor, E. (2000). The potential of Artemisia annua L. as a locally produced remedy for malaria in the tropics: agricultural, chemical and clinical aspects. J. Ethnopharmacol. 73, 487–493. doi: 10.1016/S0378-8741(00)00289-0
Mueller, M. S., Runyambo, N., Wagner, I., Borrmann, S., Dietz, K., and Heide, L. (2004). Randomized controlled trial of a traditional preparation of Artemisia annua L. (Annual Wormwood) in the treatment of malaria. Trans. R. Soc. Trop. Med. Hyg. 98, 318–321. doi: 10.1016/j.trstmh.2003.09.001
Nagamune, K., Moreno, S. N. J., and Sibley, L. D. (2007). Artemisinin-resistant mutants of Toxoplasma gondii have altered calcium homeostasis. Antimicrob. Agents Chemother. 51, 3816–3823. doi: 10.1128/AAC.00582-07
Oliveira, T. C., Silva, D. A., Rostkowska, C., Béla, S. R., Ferro, E. A., Magalhães, P. M., et al. (2009). Toxoplasma gondii: effects of Artemisia annua L. on susceptibility to infection in experimental models in vitro and in vivo. Exp. Parasitol. 122, 233–241. doi: 10.1016/j.exppara.2009.04.010
Pandey, N., and Pandey-Rai, S. (2016). Updates on artemisinin: an insight to mode of actions and strategies for enhanced global production. Protoplasma 253, 15–30. doi: 10.1007/s00709-015-0805-6
Petersen, E., and Schmidt, D. R. (2003). Sulfadiazine and pyrimethamine in the postnatal treatment of congenital toxoplasmosis: what are the options? Expert Rev. Anti Infect. Ther. 175–182. doi: 10.1586/14787210.1.1.175
Quennoz, M., Bastian, C., Simonnet, X., and Grogg, A. F. (2010). Quantification of the total amount of artemisinin in leaf samples by thin layer chromatography. Int. J. Chem. 64, 755–757. doi: 10.2533/chimia.2010.755
Räth, K., Taxis, K., Walz, G., Gleiter, C. H., Li, S.-M., and Heide, L. (2004). Pharmacokinetic study of artemisinin after oral intake of a traditional preparation of Artemisia annua L. (Annual wormwood). Am. J. Trop. Med. Hyg. 70, 128–132.
Reynolds, O. L., Padula, M. P., Zeng, R., and Gurr, G. M. (2016). Silicon: potential to promote direct and indirect effects on plant defense against arthropod pests in agriculture. Front. Plant Sci. 7:744. doi: 10.3389/fpls.2016.00744
Rocha e Silva, L. F., Magalhães, P. M., Costa, M. R. F., Alecrim, M. G. C., Chaves, F. C. C., Hidalgo, A. F., et al. (2012). In vitro susceptibility of Plasmodium falciparum Welch field isolates to infusions prepared from Artemisia annua L. cultivated in the Brazilian Amazon. Mem. Inst. Oswaldo Cruz 107, 859–866. doi: 10.1590/S0074-02762012000700004
Savant, N. K., Snyder, G. H., and Datnoff, L. E. (1997). “Silicon management and sustainable rice production,” in Advances in Agronomy, ed. D. L. SPARKS (Cambridge, MA: Academic Press), 151–199.
Srivastava, N. K., and Sharma, S. (1990). Influence of micronutrient imbalance on growth and artemisinin content of Artemisia annua. Indian J. Pharm. Sci. 52, 225–227.
Tenter, A. M., Heckeroth, A. R., and Weiss, L. M. (2000). Toxoplasma gondii: from animals to humans. Int. J. Parasitol. 30, 1217–1258. doi: 10.1016/S0020-7519(00)00124-7
Tubaña, B. S., and Heckman, J. R. (2015). “Silicon in soils and plants,” in Silicon and Plant Diseases, eds Rodrigues, A. Fabrício, Datnoff, and E. Lawrence (New York, NY: Springer International Publishing), 7–51. doi: 10.1007/978-3-319-22930-0_2
van der Kooy, F., and Verpoorte, R. (2011). The content of artemisinin in the Artemisia annua tea infusion. Planta Med. 77, 1754–1756. doi: 10.1055/s-0030-1271065
Wagner, G. J., Wang, E., and Shepherd, R. W. (2004). New approaches for studying and exploiting an old protuberance, the plant trichome. Ann. Bot. 93, 3–11. doi: 10.1093/aob/mch011
Keywords: Artemisia annua, artemisinin, silicon, Toxoplasma gondii, herbal medicine
Citation: Rostkowska C, Mota CM, Oliveira TC, Santiago FM, Oliveira LA, Korndörfer GH, Lana RMQ, Rossi ML, Nogueira NL, Simonnet X, Mineo TWP, Silva DAO and Mineo JR (2016) Si-Accumulation In Artemisia annua Glandular Trichomes Increases Artemisinin Concentration, but Does Not Interfere In the Impairment of Toxoplasma gondii Growth. Front. Plant Sci. 7:1430. doi: 10.3389/fpls.2016.01430
Received: 17 May 2016; Accepted: 08 September 2016;
Published: 23 September 2016.
Edited by:
Fernando Carlos Gómez-Merino, Colegio de Postgraduados, MexicoReviewed by:
Monika Wimmer, University of Bonn, GermanyVictoria Fernandez, Technical University of Madrid, Spain
Copyright © 2016 Rostkowska, Mota, Oliveira, Santiago, Oliveira, Korndörfer, Lana, Rossi, Nogueira, Simonnet, Mineo, Silva and Mineo. This is an open-access article distributed under the terms of the Creative Commons Attribution License (CC BY). The use, distribution or reproduction in other forums is permitted, provided the original author(s) or licensor are credited and that the original publication in this journal is cited, in accordance with accepted academic practice. No use, distribution or reproduction is permitted which does not comply with these terms.
*Correspondence: José R. Mineo, jrmineo@ufu.br