- 1School of Life Science and Technology, University of Electronic Science and Technology of China, Chengdu, China
- 2Center of Informational Biology, University of Electronic Science and Technology of China, Chengdu, China
As an important relative of wheat (Triticum aestivum L), Dasypyrum breviaristatum contains novel high molecular weight glutenin subunits (HMW-GSs) encoded by Glu-1Vb genes. We identified new wheat—D. breviaristatum chromosome introgression lines including chromosomes 1Vb and 1VbL.5VbL by fluorescence in situ hybridization (FISH) combined with molecular markers. We found that chromosome changes occurred in the wheat—D. breviaristatum introgression lines and particularly induced the deletion of 5BS terminal repeats and formation of a new type of 5B-7B reciprocal translocation. The results imply that the D. breviaristatum chromosome 1Vb may contain genes which induce chromosomal recombination in wheat background. Ten putative high molecular weight glutenin subunit (HMW-GS) genes from D. breviaristatum and wheat—D. breviaristatum introgression lines were isolated. The lengths of the HMW-GS genes in Dasypyrum were significantly shorter than typical HMW-GS of common wheat. A new y-type HMW-GS gene, named Glu-Vb1y, was characterized in wheat—D. breviaristatum 1Vb introgression lines. The new wheat—D. breviaristatum germplasm displayed reduced plant height, increased tillers and superior grain protein and gluten contents, improved gluten performance index. The results showed considerable potential for utilization of D. breviaristatum chromosome 1Vb segments in future wheat improvement.
Introduction
The high-molecular weight glutenin subunits (HMW-GS) are well-conserved endosperm proteins in the grain of wheat and related genus or species (Lawrence and Shepherd, 1981; Shewry et al., 2003). Much attentions have been paid over several decades to demonstrate that the HMW-GS can largely influence grain end-use quality (Shewry et al., 1992). Although the HMW-GS encoded by the loci Glu-1 on long arm of wheat homoeologous chromosome group 1 only represent about 10% of grain protein (Payne et al., 1980), the variation of some allelic compositions of Glu-1 loci were firmly comfirmed to be associated with bread-making quality (Anderson and Greene, 1989; Halford et al., 1992). A number of Glu-1 genes from Triticeae species have been sequenced (Shewry et al., 2003; Liang et al., 2015), and the efforts have also been made to transfer Glu-1 alleles from related species by wide hybridization and chromosomal engineering approaches (Mackie et al., 1996; Cao et al., 2007; Garg et al., 2009a; Li et al., 2013). Novel Glu-1 loci from wheat relatives were also demonstrated to have positive effects on wheat improvement of end-use quality (Garg et al., 2009b). Meanwhile, the well-studied HMW-GS genes provide a useful model for the study of genetic variation during the process of wheat-alien hybridization and the development of introgression lines (Liu et al., 2008; Yuan et al., 2014).
Dasypyrum species possess agronomically important genes controlling traits including disease resistance, high protein contents and drought adaptation, which represent valuable resources for wheat improvement over the world (Zhang et al., 2005; De Pace et al., 2011). The annual species Dasypyrum villosum carries effective resistance genes to several fungal pathogens of wheat and has genes which may have a positive contribution to quality improvement when transferred into a wheat background (Chen et al., 1995; Patrizia et al., 2010; Qi et al., 2011; Zhao et al., 2010, 2015). It is thus essential to localize the novel seed proteins from D. villosum and to characterize the HMW-GS genes from wheat—D. villosum derivatives (Zhong and Qualset, 1993; Vaccino et al., 2010; Zhang et al., 2014; Yang et al., 2014). As an allotetraploid species of the genus Dasypyrum, D. breviaristatum (genome VVVbVb), has been previously studied with the aim of transferring useful genes into wheat (Yang et al., 2005; Baum et al., 2014) and such research has included the identification and localization of resistance genes on specific D. breviaristatum chromosomes (Li et al., 2014, 2016a). Meanwhile, the characterization of wheat—D. breviaristatum chromosomes derivatives has also revealed the genomic divergence between D. breviaristatum and D. villosum.
The aim of this study is to characterize new wheat—D. breviaristatum derivative lines carrying novel HMW-GS by fluorescence in situ hybridization (FISH) and molecular marker analysis, and to isolate the Dasypyrum specific HMW-GS genes with related to Glu-1 evolution in Triticeae species and wheat quality improvement.
Materials and Methods
Plant Material
Dasypyrum breviaristatum accession PI 546317 was provided by the National Small Grains Collection at Aberdeen, ID, United States. The wheat—D. breviaristatum partial amphiploid TDH-2 (2n = 42, genome AABBVbVb) has been previously described by Yang et al. (2005). Lines D2176, D2186 and D2533 were obtained from a BC1F6 generation of the wheat variety Mianyang 11′ (MY11) crosses to wheat line ML19 and TDH-2 hybrids.
Fluorescence in Situ Hybridization (FISH)
Chromosome preparation of mitotic metaphase from seedling root tips were followed the procedure of Han et al. (2006). In order to identify the wheat and Dasypyrum chromosomes, the sequences of synthesized oligo-nucleotide probes Oligo-pSc119.2, Oligo-pTa535 and Oligo- (GAA)7 referred the description of Tang et al. (2014) and Li et al. (2016a). The non-denaturing FISH (ND-FISH) with oligo probes was performed using the techniques described by Fu et al. (2015). The sequential FISH was conducted with long terminal repeat (LTR) pDb12H sequence (Yang et al., 2006; Liu et al., 2009) which was labeled by Alexa Fluor 488-5dUTP (Invitrogen) according to Han et al. (2006). The detection of FISH signals used an Olympus BX-51 Fluorescence microscope. The photography of FISH images was acquired with a DP-70 CCD camera.
Glutenin Separation and PCR Cloning of HMW-GS Genes
The seed storage proteins including HMW-GS were extracted and examined by SDS-PAGE using the protocol described by Li et al. (2013). A pair of degenerate primers P1 (ATGGCTAAGCGGC/TTA/GGTCCTCTTTG) and P2 (CTATCACTGGCTG/AGCCGACAATGCG) for amplifying conserved HMW-GS genes were designed according to Xia et al. (2003). The target PCR products were cloned to pGEM-T vector (Promega) and sequenced by an automatic DNA sequencer (TaKaRa Biotech, Japan). The sequence alignment and phylogenetic tree construction were carried out by MEGA 4.0 software (Tamura et al., 2007).
Grain Quality Analysis
The plant harvest for agronomic traits observations were collected from two field replications at the Xindu Experimental Station, Chengdu, China during the 2014–2017 seasons. Each 10 plants were grown in 1 m rows with 30 cm spacing between adjacent rows. The seeds quality were evaluated for each entry and plot with three replications. The protein content, wet gluten content, Zeleny sedimentation value, test weight, water absorption and of whole grains were determined using the near-infrared spectroscopy DA7250 (Perten, Sweden), according to the approved methods at Northwest A&F University, China. For testing solvent retention capacity (SRC) values, grain samples were milled using Brabender Quadramat milling system. The SRC tests were performed as described by Bettge et al. (2002). For 0.2 g wheat meal SRC, the deionized water, lactic acid solution (5% w/w), sucrose (50% w/w) and Na2CO3 solution (5% w/w) were tested for SRC-WA, SRC-LA, SRC-SU, SRC-SC, respectively. The gluten performance index (GPI) was defined as lactic acid/(sodium carbonate + sucrose) SRC values (Kweon et al., 2011). Each grain samples were measured in triplicate. Results of statistic data were analyzed by using SPSS software (version 22.0, SPSS, Chicago, IL, United States).
Results
Identification of D. breviaristatum-Specific HMW-GSs
The SDS-PAGE analysis of seed storage protein extracts revealed that the wheat—D. breviaristatum partial amphiploid TDH-2 displayed wheat HMW-GS 7+8 of Glu-B1 (Figure 1). TDH-2 contained the entire Vb chromosome and did not possess any chromosomes of the D-genome. The TDH-2 partial amphiploid had a strong glutenin subunit band with a fast electrophoretic mobility between the HMW-GS and LMW-GS regions (Figure 1, indicated by arrow). This subunit was also present in wheat—D. breviaristatum introgressions D2176, D2186 and D2533, but absent in other addition and control wheat parents ML-19 and MY11 (Figure 1). The unusual subunit was presumed to have originated from D. breviaristatum and expressed in the wheat—D. breviaristatum introgressions. Thus, we designated the additional bands in Figure 1 as a D. breviaristatum specific Glu-1 glutenin subunit.
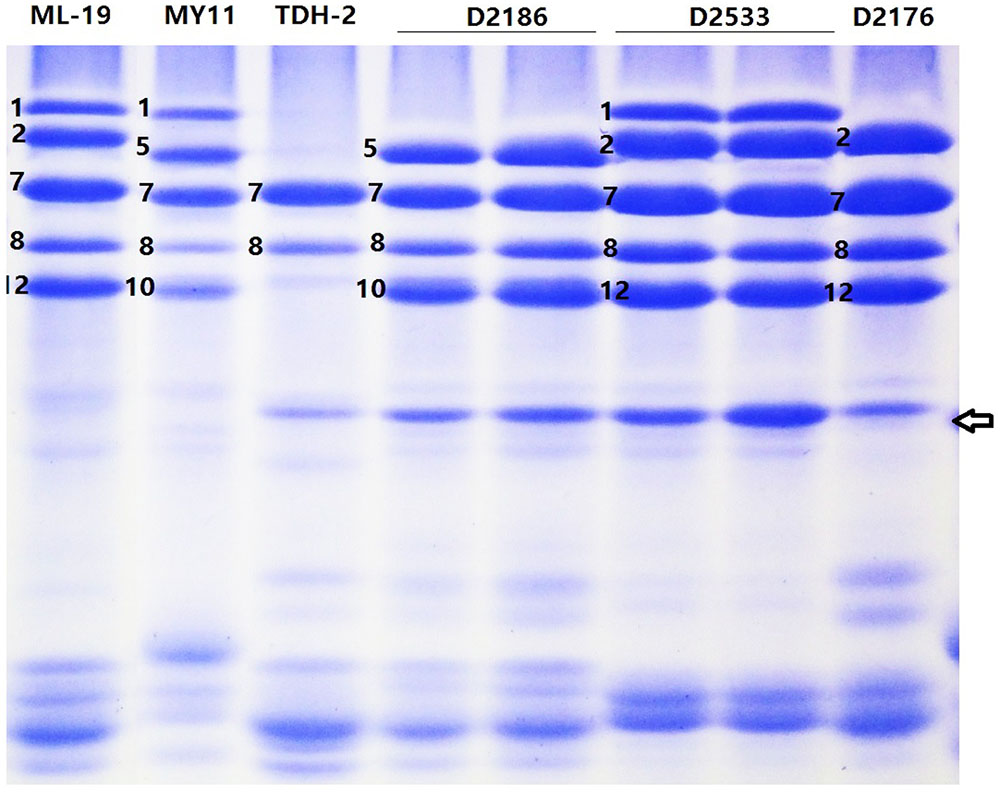
FIGURE 1. SDS-PAGE of seeds protein from wheat—D. breviaristatum lines and their parents. The arrow indicates the D. breviaristatum specific band. Electrophoretic profile indicated D. breviaristatum specific protein introduced to lines D2176, D2186 and D2533.
FISH of Wheat—D. breviaristatum Derivatives and Its Parents
Since the above identified lines carried D. breviaristatum specific glutenin bands, sequential multi-color ND-FISH by probes Oligo-pSc119.2, Oligo-pTa535 and Oligo-(GAA)7 was used to characterize the chromosome constitution of wheat—D. breviaristatum lines D2176, D2186 and D2533, in comparison with their parents TDH-2, ML-19 and MY11 (Figures 2, 3). The 28 wheat chromosomes and seven pairs of D. breviaristatum chromosomes can be identified using probes Oligo-pSc119.2 and Oligo-pTa535 in TDH-2 (Figure 2A). The wheat parents ML-19 and MY11 were also characterized by multi-color ND-FISH using probes Oligo-pSc119.2 and Oligo-pTa535. The results showed that lines ML-19 carried a pair of 1RS.1BL translocation chromosomes (Figure 2B), while MY11 did not (Figure 2C).
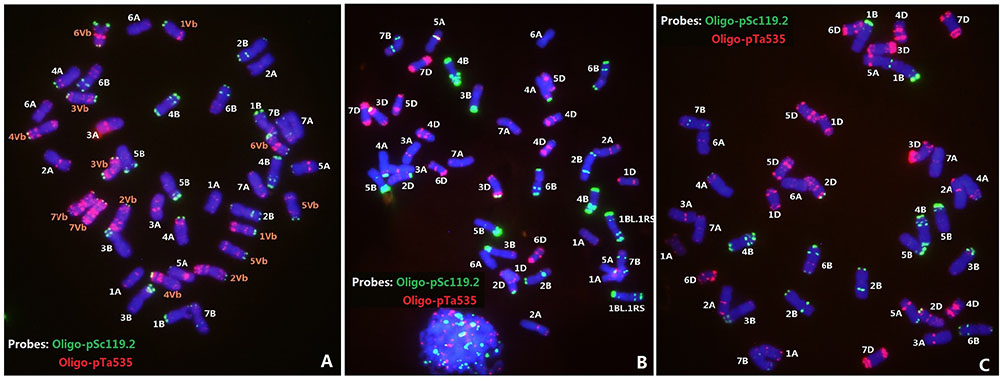
FIGURE 2. Fluorescence in situ hybridization (FISH) of wheat—D. breviaristatum partial amphiploid TDH-2 (A), and wheat line ML-19 (B) and MY11 (C). FISH with probes of Oligo-pSc119.2 (green) + Oligo-pTa535 (red) enables to precisely distinguish individual wheat and D. breviaristatum chromosomes.
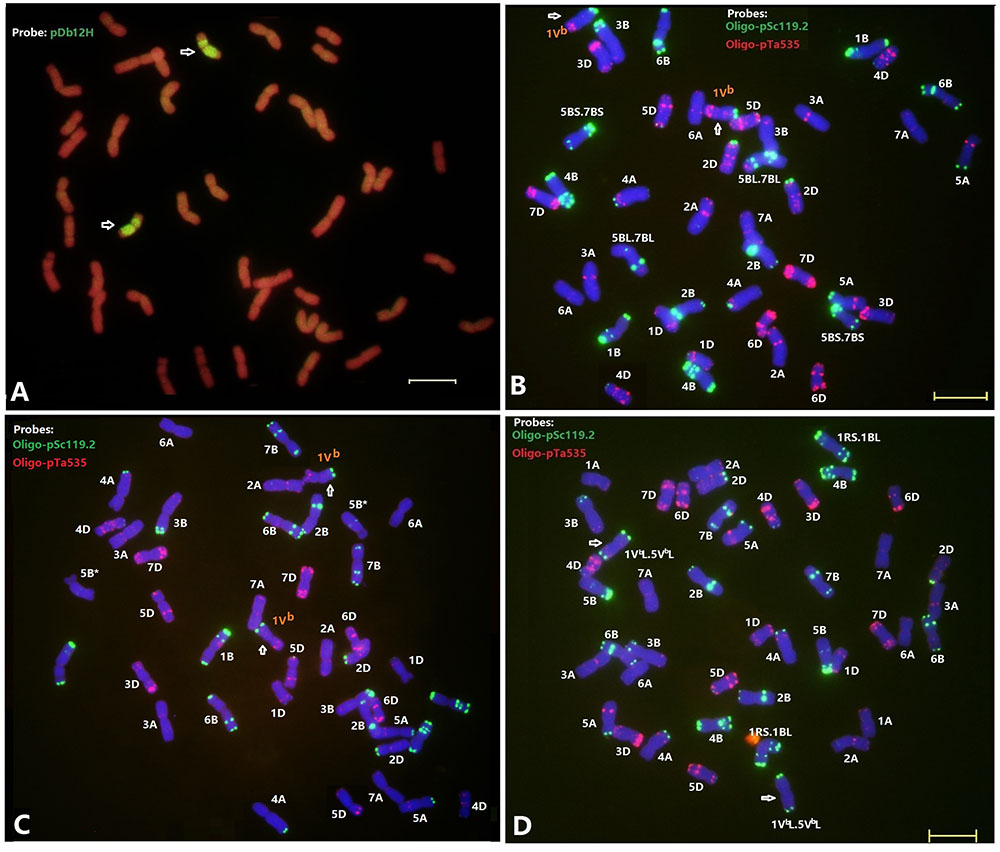
FIGURE 3. Fluorescence in situ hybridization of the wheat—D. breviaristatum introgression lines D2176 (A,B), D2186 (C), and D2533 (D). The probe for sequential FISH (A) was pDb12H (green), while probes for FISH (B–D) were Oligo-pSc119.2 (green) and Oligo-pTa535 (red). Arrows showed the D. breviaristatum chromosomes. Stars represent the modified FISH signals for chromosome 5B. Bars indicate 10 μm. FISH analysis indicates that D2176 (2n = 42) and D2186 (2n = 42) were D. breviaristatum chromosome 1Vb substitute wheat chromosome 1A, D2533 (2n = 44) was a addition with D. breviaristatum translocated chromosomes.
The FISH using the Dasypyrum specific LTR probe (Yang et al., 2006) indicated the presence of a pair of D. breviaristatum chromosomes in the wheat—D. breviaristatum lines D2176 (Figure 3A). Sequential FISH revealed that D. breviaristatum chromosomes possessed specific and distinctive bands using probes Oligo-pSc119.2 and Oligo-pTa535. The chromosome number of D2176 was 2n = 42, in which a pair of chromosomes 1A was absent, and a pair of D. breviaristatum chromosomes had been added into the wheat background. The FISH revealed that 2176 contained a pair of alien chromosomes, which displayed a faint Oligo-pSc119.2 hybridization signals at the telomeric region of the long arms, and strong hybridization signals of Oligo-pTa535 in its short arm (Figure 3B). The FISH hybridization pattern of the chromosomes was identical to D. breviaristatum chromosomes 1Vb (Figure 2). Therefore, we conclude that the line D2176 was a wheat—D. breviaristatum chromosome 1Vb substitution line. Comparing the FISH patterns of D2176 parents MY11 and TDH-2 (Figure 2), we found that the D2176 line contained a wheat chromosomal 5B-7B non-Roberstanian translocation. Similarly, FISH revealed that line D2186 with 2n = 42 contained a pair of 1Vb chromosome substituted for chromosome 1A of wheat. The wheat chromosome 5B was shown to lack any strong Oligo-pSc119.2 signals at the telomeric regions of short arms (Figure 3C). Since there are no known such translocated chromosomes in the partial amphiploid TDH-2 and the wheat parents, the changed karyotypes of D2176 and D2186 could have arisen from induced chromosome breakage associated with the introgression of D. breviaristatum chromosome in the wheat background. The FISH hybridization pattern of the chromosomes in D2533 with 2n = 44 chromosomes indicated that it contained a pair of 1RS.1BL translocation chromosomes which had been inherited from the ML-19 parent, and also a pair of D. breviaristatum chromosomes (Figure 3D). After comparing the FISH patterns of these D. breviaristatum chromosomes with TDH-2 karyotype, we found that the unknown alien chromosomes consisted of two long arm of translocated but unidentified chromosomes. Since the FISH patterns of 1VbL, 2VbL, 3VbL, and 5VbL (Li et al., 2016a) are quite similar, molecular markers need to determine the linkage group(s) of the D. breviaristatum chromosomes in D2533.
PCR Analysis of the Wheat—D. breviaristatum Introgression Lines
To identify the D. breviaristatum chromatin introduced in D2176, D2186, and D2533, PLUG markers were screened using PCR and the results compared with those of control wheat lines. A total of 18 and 32 markers previously located onto the short and long arms of wheat homoeologous group 1, respectively, were used to amplify DNA from the three introgression lines. We found that D. breviaristatum specific bands from both the short and long arms of group 1 could be visualized in D2176 (Figure 4A), however, only the long arm of group 1 specific bands could be amplified in D2533. The results showed that the D2176 and D2186 were wheat—D. breviaristatum 1Vb introgression lines, while only the 1VbL arm had been introduced to D2533. A total of 10 markers were located on the long arm of D. breviaristatum chromosome 5Vb and were mapped to the line D2533 (Figure 4B), which is similar to our previous study with chromosome 5VbL of D. breviaristatum (Zhang et al., 2015). The results showed that the 5VbL markers were present in D2533. In combination with the FISH analysis of D2533 (Figure 3C), we tentatively concluded that the D. breviaristatum chromosome in D2533 was a 1VbL.5VbL rearranged chromosome.
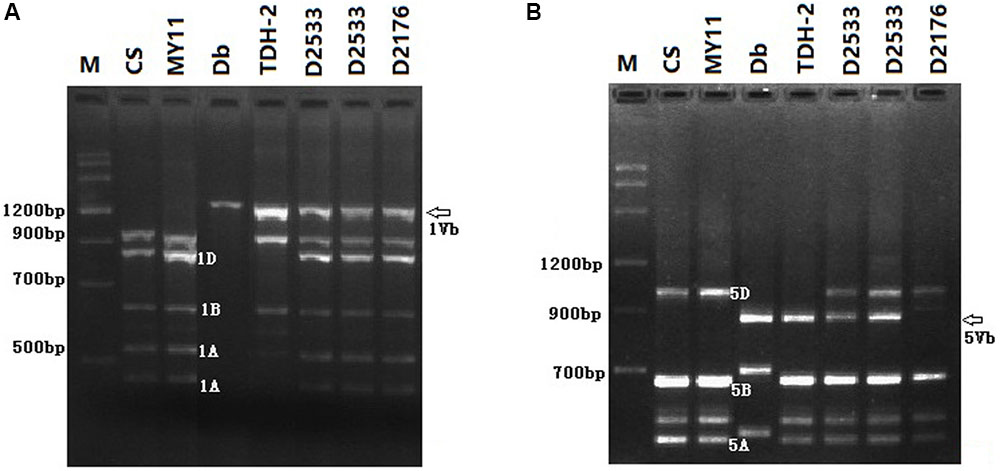
FIGURE 4. PCR analysis of markers TNAC 1021/TaqI (A) and TNAC1540/HaeIII (B) to the test lines. CS- wheat “Chinese Spring”; MY11- wheat parent; Db- D. breviaristatum; TDH-2- wheat—D. breviaristatum partial amphiploid; D2533- 1VbL.5VbL translocation; D2176- 1Vb substitution. The arrows indicate the D. breviaristatum specific bands.
Isolation of D. breviaristatum Glu-1
The AS-PCR primers P1 and P2 corresponding to the Glu-1 amplicon were used to amplify genomic DNA samples of D. breviaristatum and TDH-2. The amplicon was subjected to cloning and a total of 20 clones from each template were sequenced. A total of 13 different sequences were found to be highly similar to the Glu-1 consensus by Blast to NCBI database. Three sequences contained two in-frame stop codons and represented pseudogenes, and other ten sequences with intact ORFs encoding from 307 to 579 residue polypeptides, were deposited in Genbank under the accession numbers KU921609 to KU921618, respectively. Based on the prediction of the amino acid sequences, the Dasypyrum-specific subunit protein contained a signal peptide, an N-terminal domain, a central repetitive domain, and a C-terminal domain. The predicted cysteine number from five to nine was observed in the D. breviaristatum specific HMW-GS like sequences. In addition to the four D. villosum sequences (KF887414-KF887417) reported by Yang et al. (2014) and our previously cloned sequences EF524115 and EF524116, a total 16 Dasypyrum HMW-GS sequences were used to perform the phylogenetic analysis. As shown in Figure 5, the phylogenetic tree revealed that all D. breviaristatum and D. villosum HMW-GS sequences belonged to the y-type. The relative variations of the y-type of Dasypyrum HMW-GS sequences will provide the basis of interesting future studies test their effect on the quality improvement in wheat.
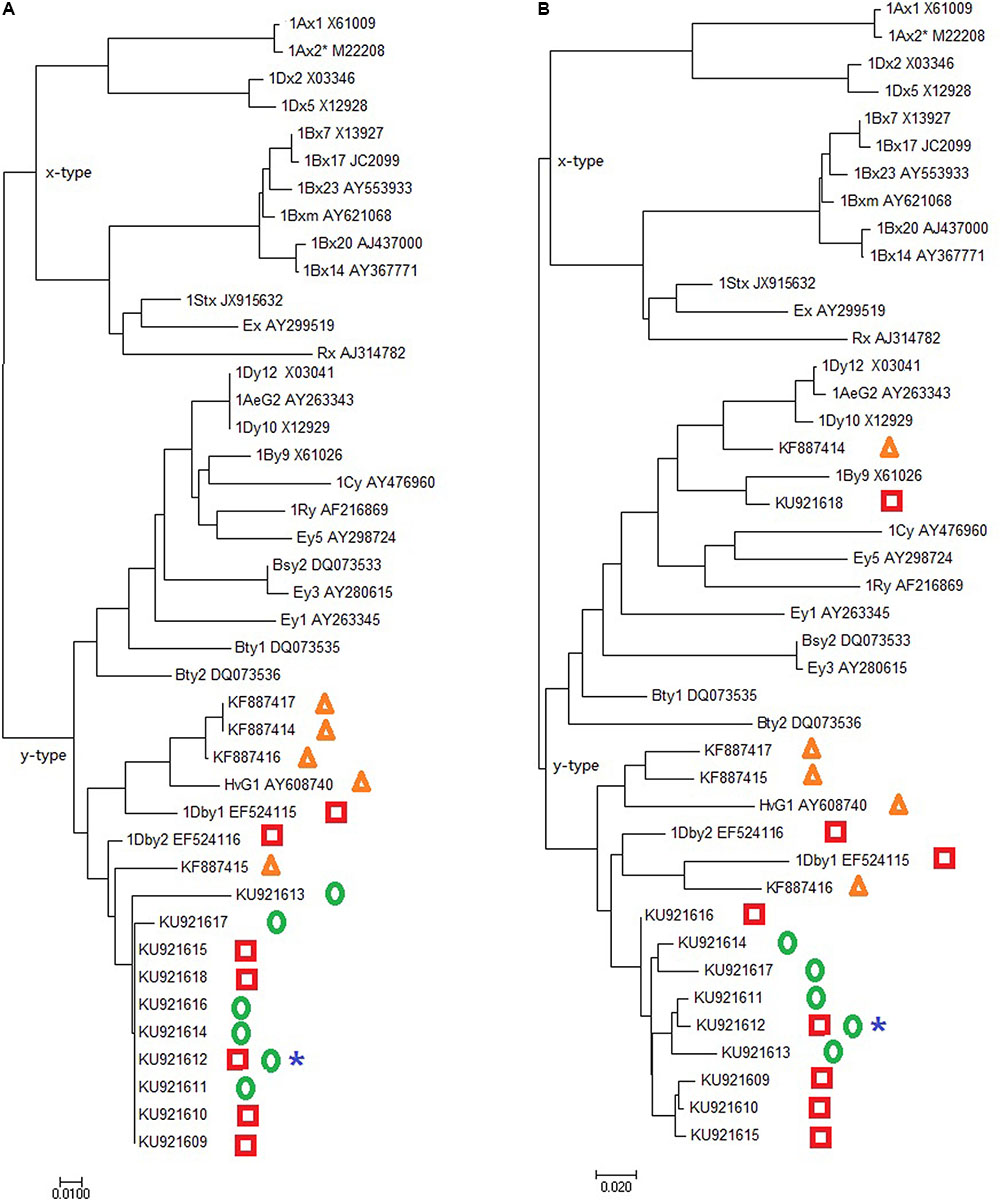
FIGURE 5. Phylogenetic tree of Glu-1 genes based on the N-terminal region (A) and C-terminal (B). The sequences from D. villosum, D. breviaristatum, TDH-2 and D2176 HMW-GS genes are marked as triangles, squares, circles and stars, respectively.
To determine whether the novel D. breviaristatum genes were transferred to the wheat—D. breviaristatum introgression lines, AS-PCR was also performed to amplify the DNA of D2176. The PCR products of 1.6-kb bands of D2176 were obtained and sent for sequencing. Besides the wheat Glu-1 fragments, we found that D2176 give rise to a sequence identical to D. breviaristatum specific to the intact 1,536-bp ORF (with Genbank number KU921612), which was identified in both the tetraploid D. breviaristatum and the wheat—D. breviaristatum partial amphiploid TDH-2. As shown in Supplementary Figure S1, the first 21 residues function as a signal peptide. Five cysteine residues were found in the 88 amino acids of N-terminal domain. The Glu-1 gene included the repetitive domains with two decapeptides, 13 nonapeptides, 32 hexapeptides, and two tripeptides. Both the number and positions of the cysteine residues in the N-terminal domain highly resembled those of typical y-type HMW subunits (Supplementary Figure S1). The D. breviaristatum Glu-1, named Glu-Vb1y, is consistent with the molecular weight indicated by SDS-PAGE results (Figure 1), suggesting that the target D. breviaristatum specific HMW-GS subunit was introduced to wheat.
Agronomic Trait Studies
Agronomic traits were measured on plants of the introgression lines D2176, D2533 and their parents grown in the field during 2015 and 2016 seasons. As shown in Figure 6 and Table 1, relative to the plant height of TDH-2 (102 cm), MY11 (80 cm) and ML-19 (95 cm), D2176 and D2533 lines had reduced plant height of 60 and 72 cm, respectively, suggesting that chromosome 1VbL may carry a dwarfing gene(s) expressed in the wheat background. The tiller number per plant was significantly increased in D2176 (averaged 14) than D2533 (averaged 7) implying that the gene(s) on chromosome 1VbS may enhance the tiller development although the partial amphiploid TDH-2 showed only a few tillers (Figure 6). The line D2176 displayed low grain weight which may be caused by the late maturity inherited from its wheat—D. breviaristatum partial amphiploid TDH-2.

TABLE 1. Agronomic and grain quality traits of wheat—Dasypyrum breviaristatum lines and the wheat parents.
Seeds Quality Test
The grain protein content (GPC), wet gluten content (WGC), Zeleny sedimentation value (ZEL), water absorption (ABS) as well as the solvent retention capacity (SRC) were tested among D2176, D2186, and D2533 and their parents MY11 and ML-19. Both D2176 and D2186 showed a increased GPC, WGC, ZEL values, indicating higher dough strength compared to the wheat lines (Table 2). The SRC provides a measure of solvent compatibility for the three functional polymeric components of flour (gluten, damaged starch, and pentosans), reflecting wheat protein quality, starch quality and dough rheology characteristics on microscale (Bettge et al., 2002). As indicated in Table 3, D2176 and D2186 showed higher SRC-LA and lower SRC-SC, SRC-SU compared to those SRC values of control wheat during the both years of 2015 and 2016 (Table 3). The gluten performance index (GPI) were calculated based on the SRC values of the flour (Kweon et al., 2011). The results indicate a clear increase in GPI of lines D2176, D2186 and D2533 compared to those of wheat parents in both years (Figure 7). It suggested that the wheat—D. breviaristatum lines may display an overall flour performance for good finished-product quality.

TABLE 2. Quality parameters of wheat—Dasypyrum breviaristatum lines in comparison to the wheat parents.
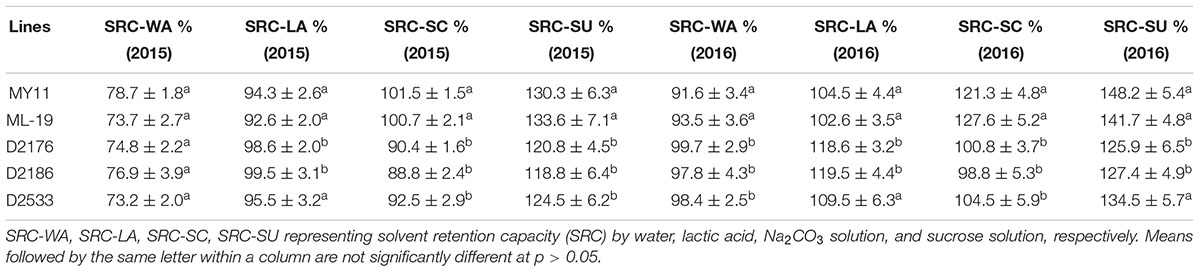
TABLE 3. Solvent retention capacity (SRC) comparison between wheat—Dasypyrum breviaristatum lines and their wheat parents by 2 years.
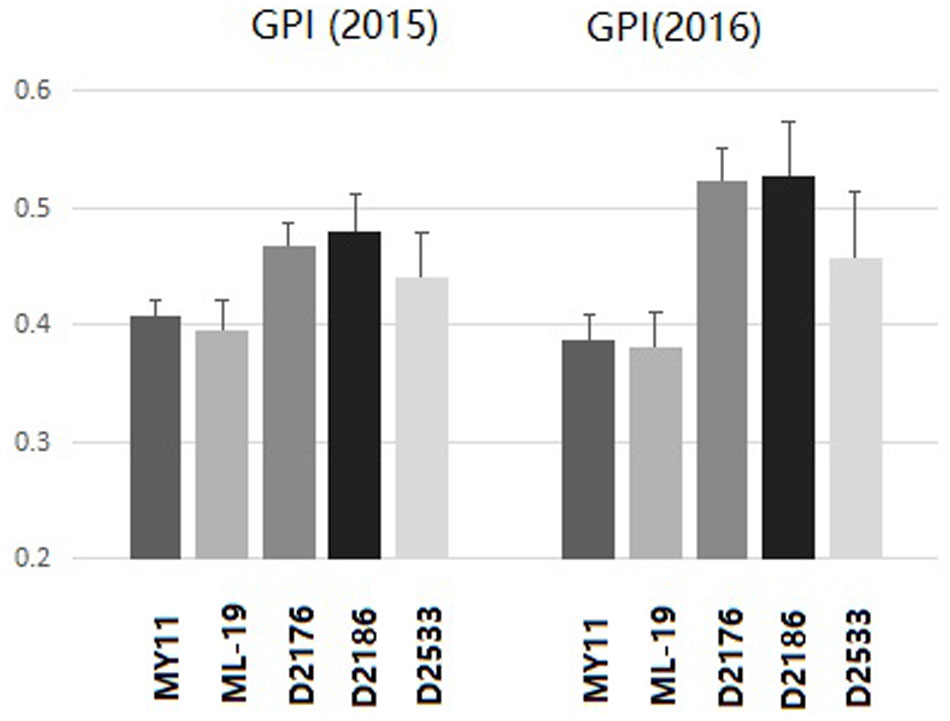
FIGURE 7. The gluten performance index (GPI) of flour for D2176, D2178, D2533 compared to wheat parents MY11 and ML-19 of 2015 and 2016. The GPI indicated the gluten quality improved in wheat—D. breviaristatum lines.
Discussion
Fluorescence in situ hybridization (FISH) has been one of the most useful techniques for identifying chromosome constitution of wheat-alien derivatives (Jiang and Gill, 1993). The development of non-denaturing FISH (ND-FISH) based on synthetic labeled oligonucleotides, such as Oligo-pSc119.2 and Oligo-pTa535, enables a fast, low-cost and effective method to identify the Secale, Dasypyrum, and Thinopyrum chromosomes in a wheat background (Tang et al., 2014; Li et al., 2016a,b). Screening by FISH and GISH, a D. breviaristatum chromosome 2Vb substitution line D11-5 (Li et al., 2014), a 7Vb addition D2139 (Li et al., 2016a), and a 5VbL arm translocated onto wheat chromosome 5AS in D2146 (Zhang et al., 2015), were recently identified in a wheat background. In the present study, we screened by SDS-PAGE the HMW-GS seed proteins, and identified a D. breviaristatum specific Glu-1 band introduced to wheat in lines D2176, D2186 and D2533 (Figure 1). The FISH and molecular markers confirmed that the D. breviaristatum derived HMW-GS was located on the 1VbL. The chromosome 1Vb of D2176 and D2186 contained a satellite region, which was detected by probe pTa71 in the short arm of 1Vb (data not shown); this result conforms to the structure of wheat chromosomes where NOR regions are commonly present in the short arm of homoeologous group chromosome 1. Since the agronomic traits observations revealed that the wheat—D. breviaristatum 1Vb lines displayed a higher tiller number than their wheat parents (Figure 6), it possibly implies that the short arm of D. breviaristatum chromosome 1Vb carries genes for enhancing the tiller development, which is similar to the reputed yield-enhancing effect of rye chromosome 1RS and hence possibly useful for yield improvement in a wheat background.
Interspecific hybridization combines divergent genomes into one nucleus, and is important for polyploidization and speciation by chromosome doubling of wide hybrids and introgression by subsequent backcrossing of the hybrids (Rey et al., 2015). Alterations of alien chromosomal structure and karyotypic variations of wheat chromosomes have previously been observed in the wheat-alien amphiploids, addition and substitution lines (Dou et al., 2006; Bento et al., 2010; Fu et al., 2013, 2015). Our recent studies also revealed the occurrence of apparent structural changes to chromosomes 1B, 2B, and 7A of the wheat—D. breviaristatum partial amphiploid TDH-2, and chromosomes 1D and 3D of the wheat—D. breviaristatum 7Vb addition lines by FISH (Li et al., 2016a). In the present study, the 5BS terminal Oligo-pSc119.2 signals were absent in D2186, and reciprocal translocations between the short and long arms of chromosomes 5B and 7B were observed in D2176 (Figure 3). The structure of the translocations were confirmed to be non-Roberstanian by FISH with probes of Oligo-pSc119.2 and Oligo-pTa535 (Figure 2), as well as Oligo-(GAA)7 (Figure 8). The breakpoints 5B-7B translocation in D2176 were located in the short arm of chromosome 5B distal to the sub-telomeric signals of Oligo-pSc119.2, and proximal the peri-centromeric signal Oligo-(GAA)7 in the long arm of chromosome 7B (Figure 8). The common wheat cultivar ‘Cappelle-Desprez’ with the 5B-7B reciprocal translocation was first reported by Riley et al. (1967) and was associated with durable rust resistance in European and Australian wheat lines (Law and Worland, 1997). The 5B-7B translocation is also present in a number of other wheat and durum wheats (Badaeva et al., 2007, 2014). The FISH of three Oligo probes also conducted to the French cultivar Vilmorin-27, with a 5B-7B translocation chromosome (Figure 8C). We indicated that the breakage point of 5B-7B in Vilmorin-27 was not identical to the 5B-7B chromosomes in D2176. We assumed that the new 5B.7B reciprocal translocation was induced by D. breviaristatum chromatin during the development of D2176, since no parent of D2176 contained such a translocation. Both chromosomes 5B and 7B contained rich heterochromatin in their centromeric and subtelomeric regions, the breakage and reunion easily happened in such repetitive sequence abundant regions (Molnár et al., 2011). Our results also supported that the 5B deletion in D2186 and 5B-7B translocation in D2176, which presumably induced by the introgression of D. breviaristatum chromatin. Recently, Gorafi et al. (2016) observed that insertions of miniature inverted-repeat transposable elements in the promoter region caused early flowering during the development of wheat—Leymus chromosome introgression lines. The results also support that the introgression of the chromosomes of wild species could promote genetic changes including both at the karyotypic level and sequence levels. The studies on the wheat-alien introgression lines provides opportunity to create the novel variations for future wheat breeding and the genetic studies to interpret the trait changes.
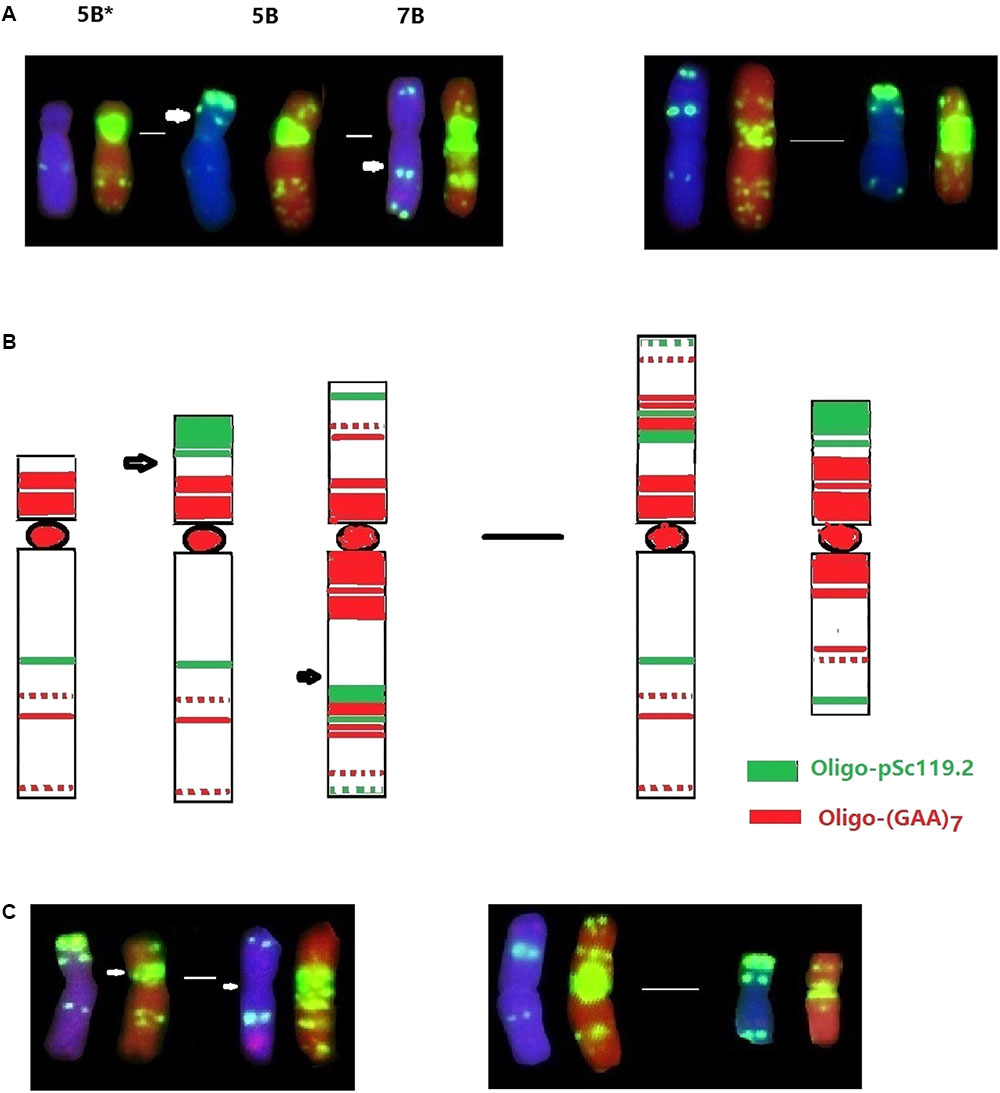
FIGURE 8. Comparison FISH karyotype of 5B deletion and 5B-7B translocations. The probes were Oligo-pSc119.2 (green) and Oligo-pTa535 (red). Arrows indicate the breakage points. The 5B deletion (5B∗) from D2176, the 5B-7B reciprocal translocation from D2186 indicated by FISH (A) and their karyotypes (B). The 5B-7B reciprocal translocation cultivar Vilmorin-27 was showed (C).
The wheat HMW glutenin locus and the orthologous loci are unique to Triticeae species suggests that these loci evolved relatively recently (Shewry et al., 1995; Gu et al., 2006). The alignments and phylogenetic trees of obtained Glu-1 sequences from wheat and its related Triticeae species have revealed a remarkable conservation of these protein genes (Gu et al., 2004; Wang et al., 2007). Novel variant of HMW glutenin subunits genes of Pseudoroegneria stipifolia (Li et al., 2008; Zhang et al., 2016), Thinopyrum elongatum (Liu et al., 2008), Th. intermedium (Cao et al., 2014), and Elymus glaucus (Jiang et al., 2010) showed smaller size than those of conventional HMW-GS subunits due to the short length of their repetitive domain. It is reasonable to assume that the parallel evolution of gene copy number and the gene length amplification in the orthologous HMW-GS genes has occurred during the divergence of wheat and its relative species. Southern hybridization seems to be a feasible method for testing the copy number variation of Glu-1 like loci in Dasypyrum genomes and their derivatives. The significant sequence changes occurred may due to the tetraploidization event of Dasypyrum speciation after the split of barley from Triticeae (Liu et al., 2010; Li et al., 2014). It is therefore noteworthy that the clear redundancy in both D. breviaristatum and D. villosum HMW-GS genes derived after a long period of cross-hybridization and polyploidization, may provide a clue to interpret the length of Glu-1 sequences from wheat’s ancestors to those in modern wheat species.
The Dasypyrum genus contains only two species, which represent unique evolutionary entities in Triticeae tribe (De Pace et al., 2011). Based on the SDS-PAGE analysis and cytological identification of the wheat—Dasypyrum materials, Zhong and Qualset (1993) showed that a large variability exists for Glu-V1 of D. villosum, and 14 alleles at Glu-V1 were found in their observed D. villosum accessions. Genes at locus Glu-V1 for HMW storage protein subunits are located on chromosome 1V, and prolamin genes are located on chromosomes 1V and 4V (Montebove et al., 1987; De Pace et al., 2001; Vaccino et al., 2010). The recently identified two wheat—D. villosum homozygous translocations confirmed that the HMW-GS gene of D. villosum was located on both 1VL and 1VS (Dong et al., 2013; Zhang et al., 2014; Zhao et al., 2015). In the present study, we also cloned ten sequences from Dasypyrum sequences and identified the different lengths of HMW-GS sequences in both D. breviaristatum and the wheat—D. breviaristatum partial amphiploid TDH-2. Only one sequence KU921012 named as Glu-Vb1y was found in the wheat—D. breviaristatum 1Vb line D2176. There also is the possibility that many of the D. breviaristatum Glu-1 gene sequences may be located on other chromosomes other than 1Vb. In particular, the D. breviaristatum derived Glu-1 genes showed great variation in the length and number of cysteines, such as the sequence KU921611 with 1457bp containing nine cysteines. The Glu-1Vby gene of KU921611 contained seven cysteines which were transferred to D2176 in wheat background, and the additional number of cysteines of Glu-1 may be potentially beneficial to bread-making quality. Yang et al. (2014) also found that the four HMW-GSs originated from D. villosum had positive effects on dough quality properties. In the present study, the effects of the new types of D. breviaristatum HMW-GS sequences to wheat quality were also confirmed that lines D2176 and D2186 relatively improved the flour quality of the wheat D. breviaristatum 1Vb lines by increasing the protein content, wet gluten contents, and gluten performance index from solvent retention capacity values. Previously, we identified a Thinopyrum intermedium ssp. trichophorum derived 1St#2L-specific Glu-1St#2x gene from a wheat—Th. intermedium 1St#2(1D) substitution line AS1677 which had a positive effect on wheat quality (Li et al., 2013). The Glu-1St#2x gene with ORF of 1,515 bp (Li et al., 2013), which was smaller than the present D. breviaristatum derived Glu-1Vby gene with ORF of 1,536 bp, although their protein located in the similar region by SDS-PAGE. It is thus to note that the small molecular weight HMW-GS genes from Dasypyrum and Thinopyrum chromosomes introgression can improve wheat end product quality.
Author Contributions
ZuY and GL designed the experiments. HW, HZ, BL, and ZhY performed the experiments. GL and JZ analysis the data. ZuY and GL wrote the paper.
Funding
We are thankful to the National Key Research and Development Program of China (2016YFD0102000), Applied and Basic Project (2016JY0075) from Science and Technology Department of Sichuan, China, National Natural Science Foundation of China (No. 31171542) for their financial support.
Conflict of Interest Statement
The authors declare that the research was conducted in the absence of any commercial or financial relationships that could be construed as a potential conflict of interest.
Supplementary Material
The Supplementary Material for this article can be found online at: https://www.frontiersin.org/articles/10.3389/fpls.2018.00365/full#supplementary-material
FIGURE S1 | The nucleotide and amino acid sequence of Glu-1Vby. The cysteine residues are boxed and the unique repeat motifs of decapeptides, nonapeptides, hexapeptides, tripeptides in the central repetitive region were underlined by double, dash, single, triple lines, respectively.
References
Anderson, O. D., and Greene, F. C. (1989). The characterization and comparative analysis of HMW glutenin genes from genomes A and B of hexaploid wheat. Theor. Appl. Genet. 77, 689–700. doi: 10.1007/BF00261246
Badaeva, E. D., Dedkova, O. S., Gay, G., Pukhalskyi, V. A., Zelenin, A. V., Bernard, S., et al. (2007). Chromosomal rearrangements in wheat: their types and distribution. Genome 50, 907–926. doi: 10.1139/g07-072
Badaeva, E. D., Keilwagen, J., Knüpffer, H., Waßermann, L., Dedkova, O. S., Mitrofanova, O. P., et al. (2014). Chromosomal passports provide new insights into diffusion of emmer wheat. PLoS One 10:e0128556. doi: 10.1371/journal.pone.0128556
Baum, B. R., Edwards, T., and Johnson, D. A. (2014). What does the nr5S DNA multigene family tell us about the genomic relationship between Dasypyrum breviaristatum and D. villosum (Triticeae: Poaceae)? Mol. Genet. Genomics 289, 553–565. doi: 10.1007/s00438-014-0825-5
Bento, M., Gustafson, P., Viegas, W., and Silva, M. (2010). Genome merger: from sequence rearrangements in triticale to their elimination in wheat-rye addition lines. Theor. Appl. Genet. 121, 489–497. doi: 10.1007/s00122-010-1325-6
Bettge, A. D., Morris, C. F., DeMacon, V. L., and Kidwell, K. K. (2002). Adaptation of AACC Method 56-11, solvent retention capacity, for use as an early generation selection tool for cultivar development. Cereal Chem. 79, 670–674. doi: 10.1094/CCHEM.2002.79.5.670
Cao, S., Li, Z., Gong, C., Xu, H., Yang, R., Hao, S., et al. (2014). Identification and characterization of high-molecular-weight glutenin subunits from Agropyron intermedium. PLoS One 9:e87477. doi: 10.1371/journal.pone.0087477
Cao, S., Xu, H., Li, Z., Wang, X., Wang, D., Zhang, A., et al. (2007). Identification and characterization of a novel Ag. intermedium HMW-GS gene from T. aestivum-Ag. intermedium addition lines TAI-I series. J. Cereal Sci. 45, 293–301. doi: 10.1016/j.jcs.2006.10.006
Chen, P. D., Qi, L. L., Zhou, B., Zhang, S. Z., and Liu, D. J. (1995). Development and molecular cytogenetic analysis of wheat-Haynaldia villosa 6VS/6AL translocation lines specifying resistance to powdery mildew. Theor. Appl. Genet. 91, 1125–1128. doi: 10.1007/BF00223930
De Pace, C., Snidaro, D., Ciaffi, M., Vittori, D., Ciofo, A., Cenci, A., et al. (2001). Introgression of Dasypyrum villosum chromatin into common wheat improves grain protein quality. Euphytica 117, 67–75. doi: 10.1023/A:1004095705460
De Pace, C., Vaccino, P., Cionini, P. G., Pasquini, M., Bizzarri, M., and Qualset, C. O. (2011). “Dasypyrum,” in Wild Crop Relatives, Genomic and Breeding Resources, Cereals, Vol. 4, ed. C. Kole (Heidelberg: Springer-Verlag), 185–292. doi: 10.1007/978-3-642-14228-4_4
Dong, J., Yang, H., Zhao, W. C., Xiao-Yan, L. I., and Chen, Q. J. (2013). Agronomic traits and grain quality of Chinese Spring–Dasypyrum villosum translocation lines T1DL.1VS and T1DS.1VL. Acta Agron. Sin. 39, 1386–1390. doi: 10.3724/SP.J.1006.2013.01386
Dou, Q. W., Tanaka, H., Nakata, N., and Tsujimoto, H. (2006). Molecular cytogenetic analyses of hexaploid lines spontaneously appearing in octoploid Triticale. Theor. Appl. Genet. 114, 41–47. doi: 10.1007/s00122-006-0408-x
Fu, S., Chen, L., Wang, Y., Li, M., Yang, Z., Qiu, L., et al. (2015). Oligonucleotide probes for ND-FISH analysis to identify rye and wheat chromosomes. Sci. Rep. 5:10552. doi: 10.1038/srep10552
Fu, S., Yang, M., Fei, Y., Tan, F., Ren, Z., Yan, B., et al. (2013). Alterations and abnormal mitosis of wheat chromosomes induced by wheat-rye monosomic addition lines. PLoS One 8:e70483. doi: 10.1371/journal.pone.0070483
Garg, M., Tanaka, H., Ishikawa, N., Takata, K., Yanaka, M., and Tsujimoto, H. (2009a). Agropyron elongatum HMW-glutenins have a potential to improve wheat end-product quality through targeted chromosome introgression. J. Cereal Sci. 50, 358–363. doi: 10.1016/j.jcs.2009.06.012
Garg, M., Tanaka, H., Tsujimoto, H., and Komatsuda, T. (2009b). Exploration of Triticeae seed storage proteins for improvement of wheat end-product quality. Breed. Sci. 59, 519–528. doi: 10.1270/jsbbs.59.519
Gorafi, Y. S. A., Eltayeb, A. E., and Tsujimoto, H. (2016). Alteration of wheat vernalization requirement by alien chromosome-mediated transposition of MITE. Breed. Sci. 66, 181–190. doi: 10.1270/jsbbs.66.181
Gu, Y. Q., Coleman-Derr, D., Kong, X., and Anderson, O. D. (2004). Rapid genome evolution revealed by comparative sequence analysis of orthologous regions from four Triticeae genomes. Plant Physiol. 135, 459–470. doi: 10.1104/pp.103.038083
Gu, Y. Q., Salse, J., Coleman-Derr, D., Dupin, A., Crossman, C., Lazo, G. R., et al. (2006). Types and rates of sequence evolution at the high-molecular-weight glutenin locus in hexaploid wheat and its ancestral genomes. Genetics 174, 1493–1504. doi: 10.1534/genetics.106.060756
Halford, N. G., Field, J. M., Blair, H., Urwin, P., Moore, K., Robert, L., et al. (1992). Analysis of HMW glutenin subunits encoded by chromosome 1A of bread wheat (Triticum aestivum L.) indicates quantitative effects on grain quality. Theor. Appl. Genet. 83, 373–378. doi: 10.1007/BF00224285
Han, F., Lamb, J. C., and Birchler, J. A. (2006). High frequency of centromere inactivation resulting in stable dicentric chromosomes of maize. Proc. Natl. Acad. Sci. U.S.A. 103, 3238–3243. doi: 10.1073/pnas.0509650103
Jiang, J., and Gill, B. S. (1993). Sequential chromosome banding and in situ hybridization analysis. Genome 36, 792–795. doi: 10.1139/g93-104
Jiang, Q. T., Wei, Y. M., Lu, Z. X., Pu, Z. E., Lan, X. J., and Zheng, Y. L. (2010). Structural variation and evolutionary relationship of novel HMW glutenin subunits from Elymus glaucus. Hereditas 147, 136–141. doi: 10.1111/j.1601-5223.2010.02182.x
Kweon, M., Slade, L., and Levine, H. (2011). Solvent retention capacity (SRC) testing of wheat flour: principles and value in predicting flour functionality in different wheat-based food processes and in wheat breeding—A review. Cereal Chem. 88, 537–552. doi: 10.1094/CCHEM-07-11-0092
Law, C. N., and Worland, A. J. (1997). The control of adult-plant resistance to yellow rust by the translocated chromosome 5BS-7BS of bread wheat. Plant Breed. 116, 59–63. doi: 10.1111/j.1439-0523.1997.tb00975.x
Lawrence, G. J., and Shepherd, K. W. (1981). Chromosomal location of genes controlling seed proteins in species related to wheat. Theor. Appl. Genet. 59, 25–31. doi: 10.1007/BF00275771
Li, G., Gao, D., Zhang, H., Li, J., Wang, H., La, S., et al. (2016a). Molecular cytogenetic characterization of Dasypyrum breviaristatum chromosomes in wheat background revealing the genomic divergence between Dasypyrum species. Mol. Cytogenet. 9:6. doi: 10.1186/s13039-016-0217-0
Li, G., Wang, H., Lang, T., Li, J., La, S., Yang, E., et al. (2016b). New molecular markers and cytogenetic probes enable chromosome identification of wheat-Thinopyrum intermedium introgression lines for improving protein and gluten contents. Planta 244, 1–12. doi: 10.1007/s00425-016-2554-y
Li, G. R., Liu, C., Li, C. H., Zhao, J. M., Zhou, L., Dai, G., et al. (2013). Introgression of a novel Thinopyrum intermedium St-chromosome-specific HMW-GS gene into wheat. Mol. Breed. 31, 843–853. doi: 10.1007/s11032-013-9838-8
Li, G. R., Zhao, J. M., Li, D. H., Yang, E. N., Huang, Y. F., Liu, C., et al. (2014). A novel wheat—Dasypyrum breviaristatum substitution line with stripe rust resistance. Cytogenet. Genome. Res. 143, 280–287. doi: 10.1159/000366051
Li, Z. X., Zhang, X. Q., Zhang, H. G., Cao, S. H., Wang, D. W., Hao, S. T., et al. (2008). Isolation and characterization of a novel variant of HMW glutenin subunit gene from the St genome of Pseudoroegneria stipifolia. J. Cereal Sci. 47, 429–437. doi: 10.1016/j.jcs.2007.05.010
Liang, X., Zhen, S., Han, C., Wang, C., Li, X., Ma, W., et al. (2015). Molecular characterization and marker development for hexaploid wheat-specific HMW glutenin subunit 1By18 gene. Mol. Breed. 35:221. doi: 10.1007/s11032-015-0406-2
Liu, C., Li, G. R., Sehgal, S. K., Jia, J. Q., Yang, Z. J., Friebe, B., et al. (2010). Genome relationships in the genus Dasypyrum: evidence from molecular phylogenetic analysis and in situ hybridization. Plant Syst. Evol. 288, 149–156. doi: 10.1007/s00606-010-0319-9
Liu, C., Yang, Z. J., Jia, J. Q., Li, G. R., Zhou, J. P., and Ren, Z. L. (2009). Genomic distribution of a long terminal repeat (LTR) Sabrina-like retrotransposon in Triticeae species. Cereal Res. Commun. 37, 363–372. doi: 10.1556/CRC.37.2009.3.5
Liu, S., Gao, X., and Xia, G. (2008). Characterizing HMW-GS alleles of decaploid Agropyron elongatum in relation to evolution and wheat breeding. Theor. Appl. Genet. 116, 325–334. doi: 10.1007/s00122-007-0669-z
Mackie, A. M., Lagudah, E. S., Sharp, P. J., and Lafiandra, D. (1996). Molecular and biochemical characterisation of HMW glutenin subunits from T. tauschii and the D genome of hexaploid wheat. J. Cereal Sci. 23, 213–225. doi: 10.1006/jcrs.1996.0039
Molnár, I., Cifuentes, M., Schneider, A., Benavente, E., and Molnár-Láng, M. (2011). Association between simple sequence repeat-rich chromosome regions and intergenomic translocation breakpoints in natural populations of allopolyploid wild wheats. Ann. Bot. 107, 65–76. doi: 10.1093/aob/mcq215
Montebove, L., Pace, C. D., Jan, C. C., Mugnozza, G. T. S., and Qualset, C. O. (1987). Chromosomal location of isozyme and seed storage protein genes in Dasypyrum villosum (L.) Candargy. Theor. Appl. Genet. 73, 836–845. doi: 10.1007/BF00289388
Patrizia, V., Riccardo, B., Maria, C., and Ciro, D. P. (2010). Improving the wheat genetic diversity for end-use grain quality by chromatin introgression from the wheat wild relative Dasypyrum villosum. Crop Sci. 50, 397–412. doi: 10.2135/cropsci2009.04.0179
Payne, P. I., Law, C. N., and Mudd, E. E. (1980). Control by homoeologous group 1 chromosomes of the high-molecular-weight subunits of glutenin, a major protein of wheat endosperm. Theor. Appl. Genet. 58, 113–120. doi: 10.1007/BF00263101
Qi, L. L., Pumphrey, M. O., Friebe, B., Zhang, P., Qian, C., Bowden, R. L., et al. (2011). A novel Robertsonian translocation event leads to transfer of a stem rust resistance gene (Sr52) effective against race Ug99 from Dasypyrum villosum into bread wheat. Theor. Appl. Genet. 123, 159–167. doi: 10.1007/s00122-011-1574-z
Rey, E., Molnár, I., and Doležel, J. (2015). “Genomics of wild relatives and alien introgressions,” in Alien Introgression in Wheat, eds M. Molnár-Láng, C. Ceoloni, and J. Doležel (Cham: Springer), 347–381.
Riley, R., Coucoli, H., and Chapman, V. (1967). Chromosomal interchanges and the phylogeny of wheat. Heredity 22, 233–247. doi: 10.1038/hdy.1967.29
Shewry, P. R., Halford, N. G., and Tatham, A. S. (1992). High molecular weight subunits of wheat glutenin. J. Cereal Sci. 15, 105–120. doi: 10.1016/S0733-5210(09)80062-3
Shewry, P. R., Halford, N. G., Tatham, A. S., Popineau, Y., Lafiandra, D., and Belton, P. S. (2003). The high molecular weight subunits of wheat glutenin and their role in determining wheat processing properties. Adv. Food Res. 45, 219–302. doi: 10.1016/S1043-4526(03)45006-7
Shewry, P. R., Napier, J. A., and Tatham, A. S. (1995). Seed storage proteins: structures and biosynthesis. Plant Cell 7, 945–956. doi: 10.1105/tpc.7.7.945
Tamura, K., Dudley, J., Nei, M., and Kumar, S. (2007). MEGA4: molecular evolutionary genetics analysis (MEGA) software version 4.0. Mol. Biol. Evol. 24, 1596–1599. doi: 10.1093/molbev/msm092
Tang, Z. X., Yang, Z. J., and Fu, S. L. (2014). Oligonucleotides replacing the roles of repetitive sequences pAs1, pSc119.2, pTa-535, pTa71, CCS1, and pAWRC.1 for FISH analysis. J. Appl. Genet. 55, 313–318. doi: 10.1007/s13353-014-0215-z
Vaccino, P., Banfi, R., Corbellini, M., and De Pace, C. (2010). Broadening and improving the wheat genetic diversity for end-use grain quality by introgression of chromatin from the wheat wild relative Dasypyrum villosum. Crop Sci. 50, 528–540. doi: 10.2135/cropsci2009.04.0179
Wang, J. R., Yan, Z. H., Jiang, Q. T., Wei, Y. M., Baum, B. R., and Zheng, Y. L. (2007). Sequence variations and molecular phylogenetic analyses of the HMW-GS genes from different genomes in Triticeae. Biochem. Syst. Ecol. 35, 421–433. doi: 10.1016/j.bse.2006.12.002
Xia, G., Xiang, F., Zhou, A., Wang, H., and Chen, H. (2003). Asymmetric somatic hybridization between wheat (Triticum aestivum L.) and Agropyron elongatum (Host) Nevishi. Theor. Appl. Genet. 107, 299–305. doi: 10.1007/s00122-003-1247-7
Yang, H., Gao, X., Chen, Q. J., Zhao, W. C., Dong, J., Xiao-Yan, L. I., et al. (2014). Isolation, characterization and Farinograph analysis of novel HMW-GSs from Dasypyrum villosum. Acta Agron. Sin. 40, 600–610. doi: 10.3724/SP.J.1006.2014.00600
Yang, Z. J., Li, G. R., Feng, J., Jiang, H. R., and Ren, Z. L. (2005). Molecular cytogenetic characterization and disease resistance observation of wheat—Dasypyrum breviaristatum partial amphiploid and its derivatives. Hereditas 142, 80–85. doi: 10.1111/j.1601-5223.2005.01918.x
Yang, Z. J., Liu, C., Feng, J., Guang-Rong, L. I., Zhou, J. P., Deng, K. J., et al. (2006). Studies on genome relationship and species-specific PCR marker for Dasypyrum breviaristatum in Triticeae. Hereditas 143, 47–54. doi: 10.1111/j.2006.0018-0661.01930.x
Yuan, Z., Liu, M., Ouyang, Y., Zeng, X., Hao, M., Zhang, L., et al. (2014). The detection of a de novo allele of the Glu-1Dx gene in wheat–rye hybrid offspring. Theor. Appl. Genet. 127, 2173–2182. doi: 10.1007/s00122-014-2370-3
Zhang, H., Li, G., Li, D., Dan, G., Jie, Z., Yang, E., et al. (2015). Molecular and cytogenetic characterization of new wheat—Dasypyrum breviaristatum derivatives with post-harvest re-growth habit. Genes 6, 1242–1255. doi: 10.3390/genes6041242
Zhang, L., Li, Z., Fan, R., Bo, W., and Zhang, X. (2016). Structural characterization and evolutionary relationship of high-molecular-weight glutenin subunit genes in Roegneria nakaii and Roegneria alashanica. Int. J. Mol. Sci. 17:1115. doi: 10.3390/ijms17071115
Zhang, Q., Li, Q., Wang, X., Wang, H., Lang, S., Wang, Y., et al. (2005). Development and characterization of a Triticum aestivum-Haynaldia villosa translocation line T4VS.4DL conferring resistance to wheat spindle streak mosaic virus. Euphytica 145, 317–320. doi: 10.1007/s10681-005-1743-8
Zhang, R., Zhang, M., Wang, X., and Chen, P. (2014). Introduction of chromosome segment carrying the seed storage protein genes from chromosome 1V of Dasypyrum villosum showed positive effect on bread-making quality of common wheat. Theor. Appl. Genet. 127, 523–533. doi: 10.1007/s00122-013-2244-0
Zhao, W., Qi, L., Gao, X., Zhang, G., Dong, J., Chen, Q., et al. (2010). Development and characterization of two new Triticum aestivum-Dasypyrum villosum Robertsonian translocation lines T1DS⋅1V#3L and T1DL⋅1V#3S and their effect on grain quality. Euphytica 175, 343–350. doi: 10.1007/s10681-010-0177-0
Zhao, W. C., Gao, X., Dong, J., Zhao, Z. J., Chen, Q. G., Chen, L. G., et al. (2015). Stripe rust resistance and dough quality of new wheat - Dasypyrum villosum translocation lines T1DL⋅1V#3S and T1DS⋅1V#3L and the location of HMW-GS genes. Genet. Mol. Res. 14, 8077–8083. doi: 10.4238/2015.July.17.16
Keywords: Dasypyrum, glutenin, FISH, grain quality, wheat
Citation: Wang H, Zhang H, Li B, Yu Z, Li G, Zhang J and Yang Z (2018) Molecular Cytogenetic Characterization of New Wheat—Dasypyrum breviaristatum Introgression Lines for Improving Grain Quality of Wheat. Front. Plant Sci. 9:365. doi: 10.3389/fpls.2018.00365
Received: 26 October 2017; Accepted: 05 March 2018;
Published: 19 March 2018.
Edited by:
Chengdao Li, Murdoch University, AustraliaReviewed by:
Hamid Khazaei, University of Saskatchewan, CanadaGalina Suvorova, All-Russia Research Institute of Legumes and Groat Crops, Russia
Copyright © 2018 Wang, Zhang, Li, Yu, Li, Zhang and Yang. This is an open-access article distributed under the terms of the Creative Commons Attribution License (CC BY). The use, distribution or reproduction in other forums is permitted, provided the original author(s) and the copyright owner are credited and that the original publication in this journal is cited, in accordance with accepted academic practice. No use, distribution or reproduction is permitted which does not comply with these terms.
*Correspondence: Zujun Yang, yangzujun@uestc.edu.cn
†These authors have contributed equally to this work.