Widespread Environmental Presence of Multidrug-Resistant Salmonella in an Equine Veterinary Hospital That Received Local and International Horses
- 1Escuela de Medicina Veterinaria, Facultad de Ciencias de la Vida, Universidad Andres Bello, Santiago, Chile
- 2Millennium Initiative for Collaborative Research on Bacterial Resistance (MICROB-R), Santiago, Chile
- 3Facultad de Medicina, Pontificia Universidad Católica de Chile, Santiago, Chile
- 4Unidad de Epidemiología Veterinaria, Departamento Medicina Preventiva Animal, Facultad de Ciencias Veterinarias y Pecuarias, Universidad de Chile, Santiago, Chile
- 5Laboratorio de Investigación de Agentes Antimicrobianos, Departamento de Microbiología, Facultad de Ciencias Biológicas, Universidad de Concepción, Concepción, Chile
- 6Escuela de Medicina Veterinaria, Pontificia Universidad Católica de Chile, Santiago, Chile
Salmonella enterica is a highly infectious microorganism responsible for many outbreaks reported in equine hospitals. Outbreaks are characterized by high morbidity and mortality rates, nosocomial transmission to other patients, zoonotic transmission to hospital personnel, and even closure of facilities. In this study, 545 samples (environmental and hospitalized patients) were collected monthly during a 1-year period from human and animal contact surfaces in an equine hospital that received local and international horses. A total of 22 Salmonella isolates were obtained from human contact surfaces (e.g., offices and pharmacy) and animal contact surfaces (e.g., stalls, surgery room, and waterers), and one isolate from a horse. Molecular serotyping revealed 18 isolates as Salmonella Typhimurium and three as Salmonella Infantis. Nineteen isolates were resistant to at least one antimicrobial class, and only two isolates were susceptible to all antimicrobials tested. In addition, we identified nine multidrug-resistant (MDR) isolates in S. Typhimurium, which displayed resistance to up to eight antimicrobials (i.e., amoxicillin/clavulanate, ampicillin, ciprofloxacin, chloramphenicol, streptomycin, gentamicin, trimethoprim/sulfamethoxazole, and tetracycline). Pulsed-field gel electrophoresis (PFGE) revealed the presence of three PFGE patterns permanently present in the environment of the hospital during our study. The persistent environmental presence of MDR Salmonella isolates, along with the fact that local and international horses are attended in this hospital, highlights the importance of improving biosecurity programs to prevent disease in horses and the hospital personnel and also for the global dissemination and acquisition of MDR Salmonella.
Introduction
Salmonella enterica, a Gram-negative bacteria of the family Enterobacteriaceae, is an important zoonotic pathogen that causes an estimated of 93.8 human cases and 150,000 deaths every year worldwide (1). Salmonella is usually transmitted to humans as foodborne and through contact with infected animals (2). This pathogen is a microorganism responsible for gastrointestinal disease affecting equines (among other animals) of all ages (3). Clinical symptoms include diarrhea, fever, and dehydration, with severity ranging from a subclinical colonization to a severe systemic illness (4). As a highly contagious disease, it can be reported as sporadic cases or as an outbreak (5, 6). Previous studies have reported significant mortality (38–44%) (7, 8) associated with salmonellosis outbreaks in equine veterinary hospitals (EVHs). Also, hospitalization and associated use of health-care resources increase the susceptibility of horses to strains of S. enterica disseminated by asymptomatic animals (4, 5).
It has been reported that one of the main reasons for the increasing rate of salmonellosis outbreaks are multidrug-resistant (MDR) strains of Salmonella (9–13). Last year, the New York State Veterinary Diagnostic Laboratory reported the isolation of Salmonella Group C2 from four different horse farms, which had shown the same MDR profile (14). This is rather concerning if we consider that back in the early 2000s, a strain of an MDR–Salmonella Newport (G2) was responsible of a serious outbreak in a Large Animal Teaching Hospital (9, 15). It is still unclear when or how MDR–Salmonella emerged, being one of the main suspects in the non-therapeutic use of antibiotics (14).
Salmonellosis outbreaks in animal health facilities are full of challenges beside the sole medical treatment and control the outbreak per se; they also involve communication with owners and referring veterinarians of infected horses (10). On the other hand, the consequences are serious including hospital-acquired infections of patients and hospital personnel, the establishment of expensive infection control programs, and decrease in clients' trust and hospitals' revenues and may even lead to litigation procedures (11, 12). Infection control programs should be an integral part of every animal health facility (16, 17). Several studies have reported outbreak control measurements (7, 12, 18) and assessment of protocols of contamination, which have been adopted by many facilities (16, 19). To date, there are no reports of salmonellosis in veterinary hospitals in Chile, and therefore, scarce biosecurity protocols have been established. Hence, this study was performed to determine the presence, antimicrobial resistance, and subtypes of Salmonella in the environment and patients from an EVH without reported history of outbreaks or hospital-acquired infections.
Materials and Methods
Description of the Setting and Location
The EVH is located at a thoroughbred horse racetrack at the center of the city of Santiago (Chile). It has an average flow of 100 incoming patients daily, providing equine health services to Thoroughbred, Arabian, Chilean rodeo, and Warmblood horses. This veterinary hospital has no records of outbreak or hospital-acquired infections due to Salmonella spp., and this information is remarkable in view of the lack of biosecurity measures or infection control programs (e.g., isolation of infected patients and protocols for cleaning and sanitation).
Sampling Procedure
A total of 545 samples were obtained in a longitudinal study conducted from July 2015 to June 2016. With the corresponding consent from the Chief Director, we collected both environmental (n = 61, for details see Table 1) samples and patient fecal samples, from one to nine, depending on hospitalized horses at a given time (20, 21). Samples were conducted during the afternoon on the last Friday of every month. The hospital was divided into four areas: surgical area (SA), proceeding area (PA), hospitalization area (HA), exterior area (EA), and a fifth category for equipment (EQ), similarly as described by Alinovi et al. (18) (Figure 1A). In addition, the surfaces sampled were classified into animal contact surfaces (direct contact of animals and humans) (n = 396) and human contact surfaces (direct contact of humans, but out of reach of animals) (n = 96), as previously described Alinovi et al. (20) (Figure 1A). The samples were obtained using a sterile gauze soaked in 90 ml of peptone water (Becton-Dickinson™, Franklin Lakes, NJ) and rubbed on the surface for 5 min. For patient samples, approximately 100 g of manure was collected and transferred into a sterile recipient. To avoid interference with the normal activities of the EVH, only one sample per hospitalized patient was collected on each sampling day. All the samples were maintained at 4°C during sampling and immediately transferred to the laboratory at Universidad Andres Bello (Santiago, Chile) for further analysis.
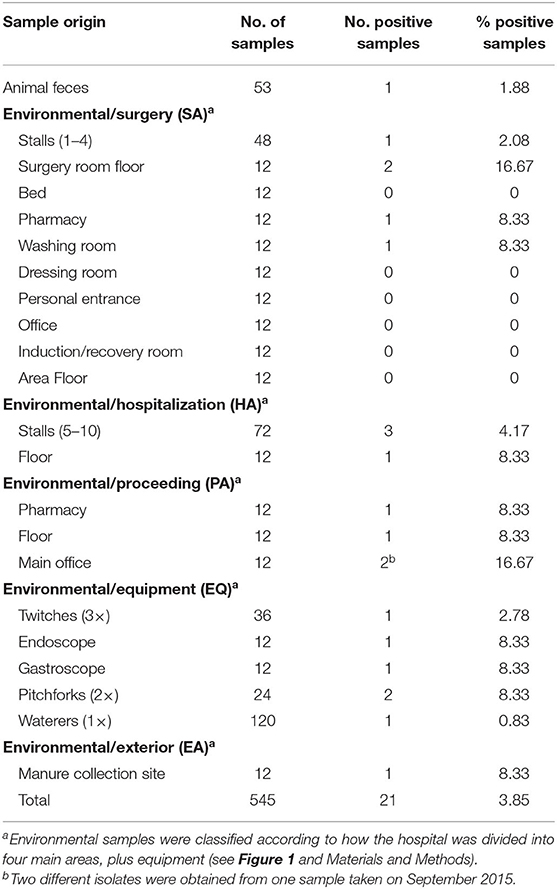
Table 1. Results of Salmonella spp. on samples collected in the equine veterinary hospital during the study.
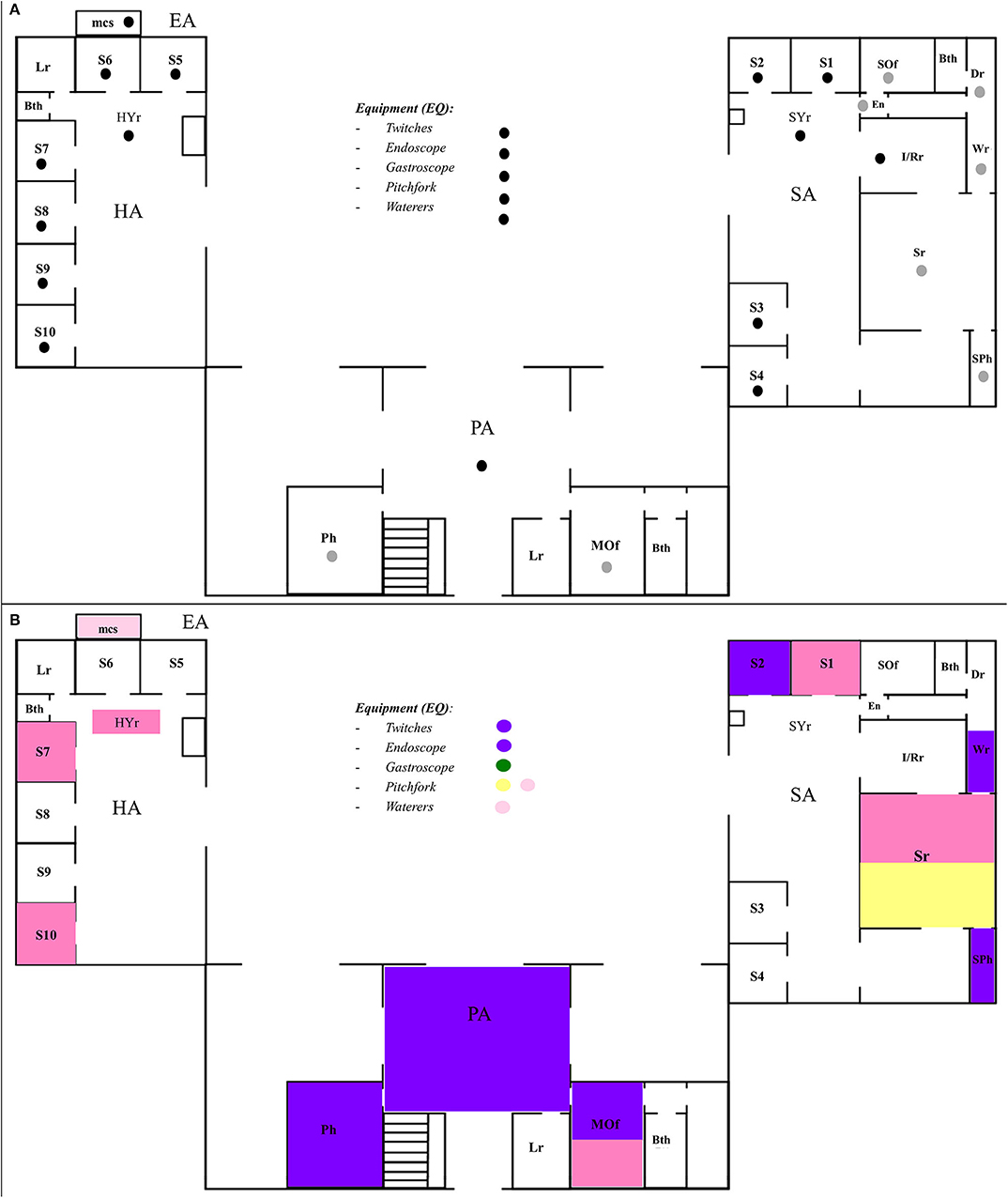
Figure 1. Schematic diagram of the equine veterinary hospital. (A) Black circles show the animal contact surfaces, whereas the white circles show the human contact surfaces. (B) Locations of the different pulse types of Salmonella enterica found in this study (colors matching Figure 2). SA, surgery area; PA, proceeding area; HA, hospitalization area; EA, exterior area; S1–10, stalls; Sr, surgery room; I/Rr, induction/recovery room, SPh, surgery pharmacy; Wr, washing room; SOf, surgery office; En, entrance; Bth, bathroom; Dr, dressing room; MOf, main office; SYr, surgery yard; HYr, hospitalization yard; mcs, manure collection site.
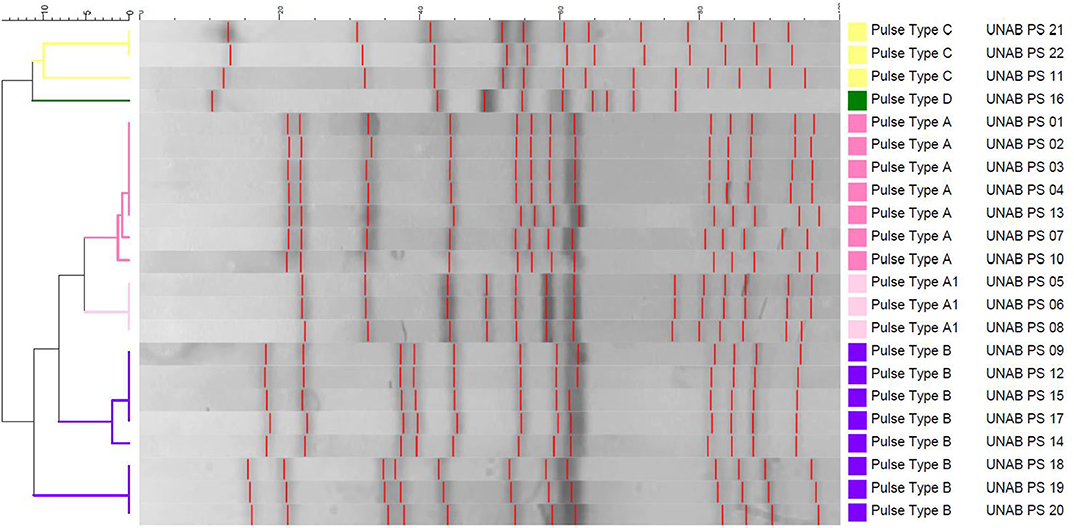
Figure 2. Dendrogram representation of Salmonella enterica isolates clustered using unweighted pair group method with arithmetic mean (UPGMA) method. Five pulse types of Salmonella were identified at the right; colors have been assigned for each pulse type (A–D), matching Figure 1B.
Bacterial Culture and Molecular Identification
Salmonella isolation was conducted as previously described (22). In brief, all samples were cultured in peptone water at 37°C overnight, and 100 μl and 1 ml were transferred into Rappaport–Vassiliadis (RV) (BD, Franklin Lakes, NJ) supplemented with novobiocin (20 mg/ml) and 100 μl of Tetrathionate (TT) (BD, Franklin Lakes, NJ) supplemented with iodine, respectively, and incubated at 42°C overnight. Finally, 100 μl of aliquot of each selective broth was streaked into an XLT-4 agar plate (BD, Franklin Lakes, NJ) and incubated at 37°C overnight. Four colonies of each agar plate were selected and transferred into Tryptic Soy Agar (TSA) (BD, Franklin Lakes, NJ). All presumed colonies of Salmonella spp. were confirmed by invA-PCR. Primers and PCR conditions used in this study have been previously described (23). Confirmed colonies were grown overnight in Trypticase Soy Broth (TSB) (BD, Franklin Lakes, NJ) and then immersed in a 20% solution of glycerol (Winkler, Santiago, Chile) and stored at −80°C.
Determination of Antimicrobial Susceptibility
The disk diffusion method of Kirby–Bauer was used to determine antimicrobial susceptibility (24). PCR-confirmed colonies were suspended in 5 ml of Mueller–Hinton (MH) broth (BD, Franklin Lakes, NJ) and incubated at 37°C overnight. Cultures were adjusted to MacFarland 0.5 (bioMérieux, France) (equivalent to 1.5 × 108 CFU/mL) and streaked on MH agar. An OXOID™ (Hampshire, UK) sensitivity disk dispenser was used, along with the antimicrobial disks, detailed as follows: amikacin (AMK; 30 μg), amoxicillin/clavulanate (AMC; 30 μg), ampicillin (AMP; 10 μg), cefoxitin (FOX; 30 μg), ceftriaxone (CTR; 30 μg), ciprofloxacin (CIP; 5 μg), chloramphenicol (CHL; 30 μg), streptomycin (STR; 300 μg), gentamicin (GEN; 10 μg), kanamycin (KAN; 30 μg), trimethoprim/sulfamethoxazole (SXT; 23.75 μg), and tetracycline (TET; 30 μg). The agar plates were incubated at 37°C overnight. Escherichia coli American Type Culture Collection (ATCC) 25922 was used as control. Interpretations were made based on the guidelines of Clinical Laboratory Standard Institute (25). The samples were classified according to Magiorakos's criteria as MDR when resistant to at least one agent in three or more antimicrobial classes (26).
Molecular Characterization of Salmonella Serotype
A previously described molecular method for serotype prediction was used (27, 28). Briefly, DNA extraction of the isolates was conducted using the DNeasy Blood and Tissue kit (QIAGEN, Hilden, Germany). The molecular scheme included an initial multiplex PCR, conducted to identify the serogroup of each isolate, followed by PCR-sequencing approaches to determine H1 and H2 antigens (27, 28). PCR products were sent to MACROGEN™ (Korea) for Sanger sequencing. Consensus sequences were obtained using CAP3 Sequence Assembly Program (http://doua.prabi.fr/software/cap3); the complementary reverse was obtained by using Bioinformatics.org. The results were analyzed using basic local alignment tool (BLAST) on the National Center for Biotechnology Information (NCBI).
Molecular Typing
Molecular typing of the isolates was conducted by pulsed-field gel electrophoresis (PFGE), using the CDC PulseNet standard protocol (29). For this, overnight cultures in brain hearth infusion broth (BHI, BD, Germany) were embedded in 1% of SeaKem® Gold Agarose (Lonza, Rockland, ME, USA). Upon lysis and washing, the plugs were digested with XbaI (Thermo Fisher Scientific Inc., Waltham, MA). The CHEF-DR® III System (Bio-Rad Laboratories, Hercules, CA) was used for the electrophoresis for 20 h. A standard, Salmonella Braenderup digested with XbaI was used. BioNumerics v 7.5 (Applied Maths, Sint-Martens-Latem, Belgium) (30) was used to analyze the PFGE images using unweighted pair group method with arithmetic mean (UPGMA) and the Dice correlation coefficient. PFGE was conducted at the Microbiology Unit of the Clinical Laboratory Services of Red Salud UC-CHRISTUS, Catholic University. The results were analyzed using Tenover guidelines as previously described (31).
Results
Salmonella spp. Were Obtained Mostly From Environmental Samples in Human Contact Surfaces
A total of 545 samples (environmental, n = 492; patient, n = 53) were analyzed. Among these, 21 samples (3.85%) yielded positive for Salmonella, which were confirmed by invA-PCR (Table 1 and Supplementary Figure 1). In 3/21 (14.2%) samples, Salmonella isolates were obtained from TT enrichments; in 10/21 (47.6%), Salmonella isolates were obtained from RV enrichments; and in the remaining 10/21 (47.6%) samples, Salmonella isolates were obtained from both enrichments conducted. On positive samples, one isolate was selected, except for one sample, in which two different colonies were obtained; therefore, a total of 22 Salmonella colonies were further characterized. From the 22 isolates, 1/22 (4.5%) was obtained from a sick Chilean rodeo patient, which died of peritonitis after colic surgery (no positive foreign patients were found), and the other 21 (21/23; 95.4%) were obtained from 20 environmental samples (i.e., stalls, surgery room floor, surgical pharmacy, washing room, hospitalization area floor, main office, pitchforks, endoscope, gastroscope, twitches, waterers, manure collection site, proceeding area floor, and pharmacy) (Table 1). Regarding the type of contact surface, 13/396 (3.28%) isolates were obtained from animal contact surfaces and 8/96 (9.38%) from human contact surfaces (Table 2). About the dates of isolation, two peaks were seen during the months of September 2015 and May 2016, where 9/22 and 8/22 isolates of Salmonella spp. were obtained, respectively (Supplementary Figure 1). A few isolates were also obtained during October 2015 (n = 1), December 2015 (n = 1), April 2016 (n = 1), and May 2016 (n = 2) (Table 2, Supplementary Figure 1).
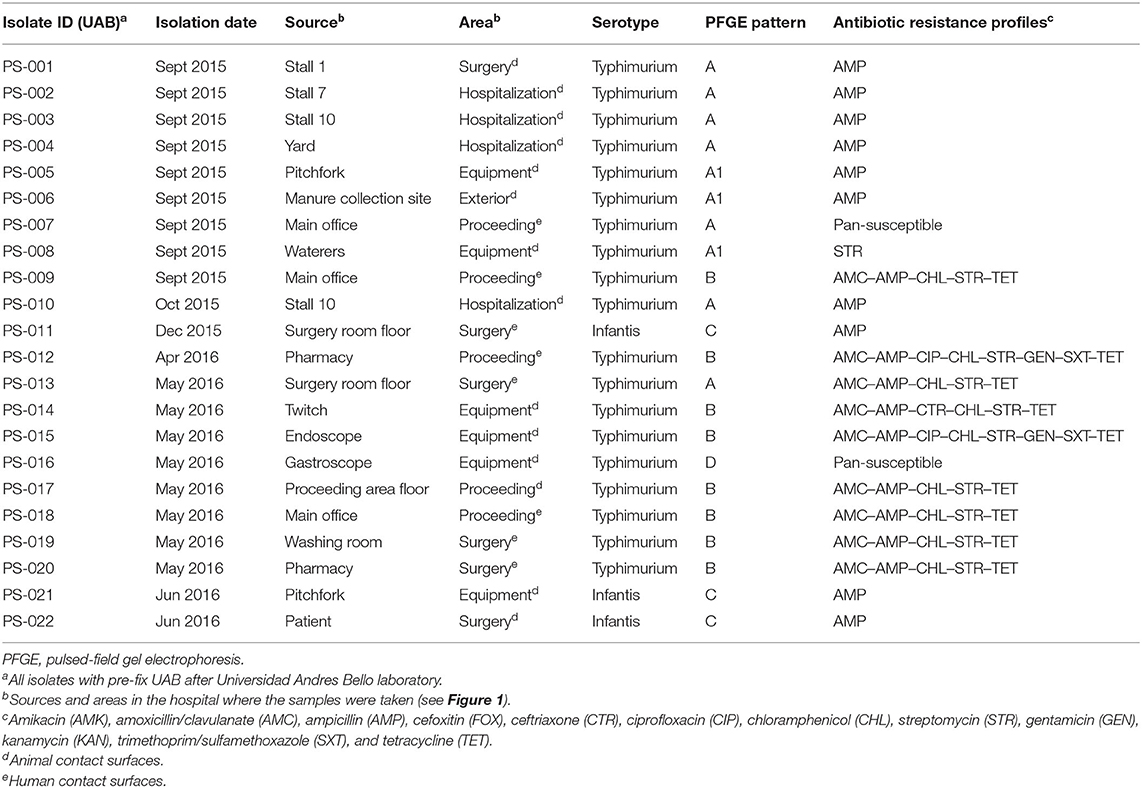
Table 2. Characteristics, serotypes, PFGE patterns, and antimicrobial resistance of Salmonella isolates.
Presence of Multidrug-Resistant Salmonella Isolates
Kirby–Bauer tests revealed six antimicrobial resistant profiles (Table 2). From the 22 Salmonella isolates, two were pan-susceptible, 10 isolates were resistant to AMP; one isolate was resistant to STR; six isolates were resistant to AMC, AMP, CHL, STR, and TET; one isolate was resistant to AMC, AMP, CTR, CHL, STR, and TET; and two isolates were resistant to AMC, AMP, CIP, CHL, STR, GEN, SXT, and TET. From these, 9/22 (40.1%) were classified as MDR, as these were resistant to one agent in three or more antimicrobial classes (26).
Predominance of Salmonella Serotype Typhimurium
All isolates were tested to predict the serogroup and serotype as described above. The molecular methods showed 19/22 (86.4%) of Salmonella isolates to O:4 (B) serogroup and three Salmonella isolates 3/22 (13.6%) to O:7 (C1) serogroup. Concerning flagellar antigens, DNA was amplified for both genes, fliC and fljB, in all Salmonella isolates. The BLAST algorithm of the FASTA consensus sequences of the PCR products allowed us to predict the serotype. All isolates belonging to O:4 (B) serogroup (20/22) yielded positive for serotype Typhimurium, whereas the isolates belonging to O:7 (C1) serogroup (2/22) were predicted as Infantis serotype (Table 2).
Five Different Pulsed-Field Gel Electrophoresis Types of Salmonella Were Identified
According to the PFGE, four PFGE patterns were identified in 19 Salmonella typhimurium isolates, and one PFGE type was found in three S. Infantis isolates. Among S. Typhimurium, seven isolates (1, 2, 3, 4, 7, 10, and 13) were indistinguishable from each other and classified as PFGE pattern A. In three isolates (5, 6, and 8), PFGE patterns were also indistinguishable from each other and related to PFGE pattern A, which was therefore classified as A1. All PFGE patterns A and A1 were detected only in the sampling of September 2015. Eight isolates (9, 12, 14, 15, 17, 18, 19, and 20) were indistinguishable from each other and different from all others, classified as PFGE pattern B; these isolates were obtained in samplings of September 2015 and in April and May 2016. One additional PFGE pattern D of isolate 16 was found in S. Typhimurium. Isolates 11, 21, and 22 were indistinguishable from each other and different from all others, classified as PFGE pattern C. Importantly, these isolates were classified as S. Infantis (Table 2).
Discussion
This study examined the environmental presence of Salmonella in an equine hospital with no history of outbreak or hospital-acquired infections. Here, we identified two serotypes that were widely distributed. The major findings of this study are the following: (i) wide spatial distribution of Salmonella in the hospital, mainly in spring and autumn; (ii) MDR Salmonella Typhimurium accounted for most of the isolates; and (iii) multiple Salmonella PFGE patterns present in human contact surfaces highlight the need of developing biosecurity standard protocols.
Wide Spatial Distribution of Salmonella in the Hospital, Mainly in Spring and Autumn
In this study, we found a considerable presence of Salmonella in the EVH environment, compared with the equine's samples. The prevalence of Salmonella in equine subclinical shedders (1–2%) tends to increase under stress conditions owing to hospitalization to 9–13% (5, 6, 10). In the environmental samples, positivity was widespread to all sampled areas (including equipment), reaching 4.5%. A previous study conducted at a large animal hospital has shown the presence of Salmonella in several areas, accounting for a positivity rate of 3.9% during a post-outbreak period (32). Importantly, in our study, no outbreak or hospital-acquired infections were reported, before and/or during the study.
It has been shown that the peak incidence of salmonellosis in horses occurs in summer and autumn (5, 33), although there are some outbreak reports during spring (7). Here, we obtained Salmonella isolates in every season of the year, although the highest number of isolates was obtained during September 2015 and June 2016, spring and winter for the southern hemisphere, respectively (Table 2, Supplementary Figure 2). Our first peak, on September 2015, was an incoming Chilean rodeo patient suffering from severe acute diarrhea, which died within 24 h after being admitted to the EVH. As Salmonella was isolated from the stall of that patient, Stall 10 (Figure 1), it may have been introduced to the EVH by this patient, but further investigation is needed, which is beyond the scope of this study. Importantly, these isolates represented a closely related PFGE pattern. Nevertheless, neither official information nor patient history could be collected to explain the second peak, in June 2016. Although it is uncertain about the origin of these isolates, shedding patients present during non-sampling periods could be a common source of dissemination (5). Other possible sources of contaminations, such as other animals (rodents), feed, or even environmental persistent strains (34), are also plausible and have to be considered.
Multidrug-Resistant Salmonella Typhimurium Accounted for Most Isolates
Reported outbreaks of Salmonella in EVHs have involved serotypes such as Typhimurium, Newport, Agona, Anatum (12, 35), Infantis (36), Heidelberg (37), and Oranienburg (38). Here, we found that 87% of the isolates were represented by S. Typhimurium. This serotype has been commonly isolated from horses, causing severe clinical signs, along with high morbidity and mortality rates (7, 8, 33). In Chile, only one outbreak of S. Typhimurium has been reported, which affected weanling foals with a morbidity rate of 87% and mortality rate of 13% (39). Regarding Salmonella Infantis, which is less commonly reported compared with S. Typhimurium, only three isolates were found. Nonetheless, there is a report of a serious outbreak in 1996, which caused important economic losses and even the closure of the facilities (36).
Antimicrobial resistance profiles, which include resistance to AMP (10 isolates), as the most common profile, followed by the profile AMC–AMP–CRO–CHL–STR–TE (six isolates), include antimicrobials in which resistance has already been described in other salmonellosis outbreaks (38), not only in equine hospitals but also in small animal shelters (13). Notably, we found that almost half of the isolates (n = 10) displayed an MDR phenotype, showing resistance to three or more antimicrobial classes (26), which is a major concern for the public health, the personnel at the hospital, and the treatment of hospitalized horses.
Multiple Salmonella Pulsed-Field Gel Electrophoresis Patterns Present in Human Contact Surfaces Highlight the Need of Developing Biosecurity Standards
We found five different PFGE patterns, which were present in all areas of the hospital, including human contact surfaces. Environmental presence of Salmonella indicates that personnel without animal contact at all (e.g., secretary) could also be at risk of infection. As pointed before, no information concerning hospital-acquired infections was reported during our study, neither from incoming patients nor from veterinary staff. In the environment, Salmonella could put into high risk the incoming susceptible patients, as young horses or immunocompromised individuals (33). This leads us to think that it may be a potential risk of an outbreak. There has been reports of $755,000 USD of estimated cost to control salmonellosis outbreaks in a large animal teaching hospital in Virginia (USA) (12), which lead us to the conclusion that biosecurity standard protocols must be implemented to prevent any undesirable event (17). There are many guidelines of biosecurity protocols (e.g., rubber boots, hand washing, and foot bath) (21, 40, 41) and also published articles in which salmonellosis outbreaks have been controlled (7, 12, 16, 18, 19). Although the implementation of biosecurity protocols is quite expensive, it is much less than controlling an outbreak itself, especially considering the fact that the EVH located at a thoroughbred racetrack, harbors nearly 1,500 horses together with hospital personnel (17).
Conclusions
This study has revealed the importance of implementing mitigation strategies and biosecurity protocols to control MDR Salmonella to ensure the safety of patients and hospital personnel. Also, this could set an example for other veterinary facilities to establish or recheck their functioning biosecurity protocols, especially in developing countries.
Data Availability Statement
The datasets generated for this study are available on request to the corresponding author.
Ethics Statement
The animal study was reviewed and approved by The University Andres Bello Bioethics Committee, Santiago, Chile. Written informed consent for participation was not obtained from the owners because fresh fecal samples were obtained from the floor.
Author Contributions
PS-O designed the study, conducted the experiments, and wrote the manuscript. AM-S wrote the manuscript, analyzed data, and designed the study. DR and RT conducted the experiments. RR-N critically reviewed the manuscript. AA, GG-R, and CH-W analyzed the data. PG conducted the experiments. All authors contributed to the article and approved the submitted version.
Funding
We thank the following funding sources: ANID Millennium Science Initiative/Millennium Initiative for Collaborative Research on Bacterial Resistance, MICROB-R, NCN17_081 FONDECYT 11140108, and FONDECYT 1181167.
Conflict of Interest
The authors declare that the research was conducted in the absence of any commercial or financial relationships that could be construed as a potential conflict of interest.
Acknowledgments
We also thank the EVH in Santiago (Chile) for facilitating samplings.
Supplementary Material
The Supplementary Material for this article can be found online at: https://www.frontiersin.org/articles/10.3389/fvets.2020.00346/full#supplementary-material
References
1. Majowicz SE, Musto J, Scallan E, Angulo FJ, Kirk M, O'Brien SJ, et al. The global burden of nontyphoidal salmonella gastroenteritis. Clin Infect Dis. (2010) 50:882–9. doi: 10.1086/650733
2. Hoelzer K, Switt AIM, Wiedmann M. Animal contact as a source of human non-typhoidal salmonellosis. Vet Res. (2011) 42:1–27. doi: 10.1186/1297-9716-42-34
3. FDA - USA Food and Drug Administration. Get the Facts About Salmonella! Anim Heal Lit. (2017). Available online at: https://www.fda.gov/animal-veterinary/animal-health-literacy/get-facts-about-salmonella (accessed February 15, 2020).
4. Timoney J. Salmonella in domestic animals. 2nd edition. In: Paul A, Barrow U, editors. Methner. Oxfordshire: CABI. (2013). 305–17 p.
5. Ernst NS, Hernandez JA, MacKay RJ, Brown MP, Gaskin JM, Nguyen AD, et al. Risk factors associated with fecal salmonella shedding among hospitalized horses with signs of gastrointestinal tract disease. J Am Vet Med Assoc. (2004) 225:275–81. doi: 10.2460/javma.2004.225.275
6. Kim L, Morley PS, Traub-Dargatz JL, Salman MD, Gentry-Weeks C. Factors associated with salmonella shedding among equine colic patients at a veterinary teaching hospital. J Am Vet Med Assoc. (2001) 218:740–8. doi: 10.2460/javma.2001.218.740
7. Schott HC, Ewart SL, Walker RD, Dwyer RM, Dietrich S, Eberhart SW, et al. An outbreak of salmonellosis among horses at a veterinary teaching hospital. J Am Vet Med Assoc. (2001) 218:1152–9. doi: 10.2460/javma.2001.218.1152
8. Ward MP, Brady TH, Couëtil LL, Liljebjelke K, Maurer JJ, Ching CW. Investigation and control of an outbreak of salmonellosis caused by multidrug-resistant salmonella typhimurium in a population of hospitalized horses. Vet Microbiol. (2005) 107:233–40. doi: 10.1016/j.vetmic.2005.01.019
9. Dallap Schaer BL, Aceto H, Rankin SC. Outbreak of salmonellosis caused by Salmonella enterica serovar newport MDR-AmpC in a large animal veterinary teaching hospital. J Vet Intern Med. (2010) 24:1138–46. doi: 10.1111/j.1939-1676.2010.0546.x
10. Dargatz D A, Traub-Dargatz JL. Multidrug-resistant Salmonella and nosocomial infections, Vet Clin North Am Equine Prac. (2004) 20:587–600. doi: 10.1016/j.cveq.2004.07.008
11. Johnson J A. Nosocomial infections. Vet Clin North Am Small Anim Pract. (2002) 32:1101–26. doi: 10.1016/S0195-5616(02)00038-4
12. McMillan NS. The Management of a Multidrug-Resistant Salmonella Agona Outbreak at Large Animal Teaching Hospital. (2004) Available online at: http://scholar.lib.vt.edu/theses/available/etd-05272004-144444/unrestricted/Chapter2NSM.pdf (accessed May 11, 2015).
13. Wright JG, Tengelsen LA, Smith KE, Bender JB, Frank RK, Grendon JH, et al. Multidrug-resistant salmonella typhimurium in four animal facilities. Emerg Infect Dis. (2005) 11:1235–41. doi: 10.3201/eid1108.050111
14. Animal Health Diagnostic Center. Multi-drug Resistant Salmonella in Horses. Cornell Univ - Coll Vet Med. (2019). Available online at: https://www.vet.cornell.edu/animal-health-diagnostic-center/news/multi-drug-resistant-salmonella-horses (accessed November 18, 2019).
15. Brown KS. New Bolton Salmonella Outbreak. The Horse. (2004). Available online at: http://www.thehorse.com/print?article/11547 (accessed May 23, 2015).
16. Benedict KM, Morley PS, Van Metre DC. Characteristics of biosecurity and infection control programs at veterinary teaching hospitals. J Am Vet Med Assoc. (2008) 233:767–73. doi: 10.2460/javma.233.5.767
17. Morley PS, Anderson MEC, Burgess BA, Aceto H, Bender JB, Clark C, et al. Report of the third havemeyer workshop on infection control in equine populations. Equine Vet J. (2013) 45:131–6. doi: 10.1111/evj.12000
18. Steneroden KK, Van Metre DC, Jackson C, Morley PS. Detection and control of a nosocomial outbreak caused by salmonella newport at a large animal hospital. J Vet Intern Med. (2010) 24:606–16. doi: 10.1111/j.1939-1676.2010.0484.x
19. Alinovi CA, Ward MP, Couëtil LL, Wu CC. Detection of salmonella organisms and assessment of a protocol for removal of contamination in horse stalls at a veterinary teaching hospital. J Am Vet Med Assoc. (2003) 223:1640–4. doi: 10.2460/javma.2003.223.1640
20. Hoet AE, Johnson A, Nava-Hoet RC, Bateman S, Hillier A, Dyce J, et al. Environmental methicillin-resistant staphylococcus aureus in a veterinary teaching hospital during a nonoutbreak period. Vector Borne Zoonotic Dis. (2011) 11:609–615. doi: 10.1089/vbz.2010.0181
21. Morley PS, Burguess B, Van Metre D, Ouyang B. Infection control and biosecurity Standard Operation Procedures (SOP) JLV-VTH. Infect Control Biosecurity. (2015). Available online at: http://csu-cvmbs.colostate.edu/Documents/biosecurity-sop.pdf (accessed May 23, 2015).
22. Dueñas F, Rivera D, Toledo V, Tardone R, Hervé-claude LP, Hamilton-west C, et al. Short communication : characterization of salmonella phages from dairy calves on farms with history of diarrhea. J Dairy Sci. (2017) 100:1–5. doi: 10.3168/jds.2016-11569
23. Kim J, Lee GG, Park JS, Jung YH, Kwak HS, Kim SB, et al. A novel multiplex pcr assay for rapid and simultaneous detection of five pathogenic bacteria: Escherichia Coli O157:H7, Salmonella, Staphylococcus aureus, listeria monocytogenes, and vibrio parahaemolyticus. J Food Prot. (2007) 70:1656–62. doi: 10.4315/0362-028X-70.7.1656
24. Hudzicki J (University of KMC. Kirby Bauer Disk Diffusion Susceptibility Test Protocol. (2016) Available online at: https://www.asmscience.org/content/education/protocol/protocol.3189 (accessed January 1, 2016).
25. Clinical and Laboratory Standards Institute (CLSI). Performance Standards for Antimicrobial Susceptibility Testing. M100, 27th edition. Wayne, PA: Clinical and Laboratory Standars Institute. (2017).
26. Magiorakos A, Srinivasan A, Carey RB, Carmeli Y, Falagas ME, Giske CG, et al. Multidrug-resistant, extensively drug-resistant and pandrug-resistant bacteria: an international expert proposal for interim standard definitions for acquired resistance. Clin Microbiol Infect. (2011) 18:268–81. doi: 10.1111/j.1469-0691.2011.03570.x
27. Herrera-Leon S, Ramiro R, Arroyo M, Diaz R, Usera MA, Echeita MA. Blind comparison of traditional serotyping with three multiplex PCRs for the identification of salmonella serotypes. Res Microbiol. (2007) 158:122–7. doi: 10.1016/j.resmic.2006.09.009
28. Ranieri ML, Shi C, Moreno-Switt AI, Den Bakker HC, Wiedmann M. Comparison of typing methods with a new procedure based on sequence characterization for salmonella serovar prediction. J Clin Microbiol. (2013) 51:1786–97. doi: 10.1128/JCM.03201-12
29. Graves LM, Swaminathan B. PulseNet standardized protocol for subtyping listeria monocytogenes by macrorestriction and pulsed-field gel electrophoresis. Int J Food Microbiol. (2001) 65:55–62. doi: 10.1016/S0168-1605(00)00501-8
30. Applied Maths NV. Bionumerics Version 7.6. (2019) Available online at: http://www.applied-maths.com (accessed November 06, 2019).
31. Tenover FC, Arbeit RD, Goering R V, Mickelsen PA, Murray BE, Persing DH, et al. Interpreting chromosomal DNA restriction patterns produced by pulsed- field gel electrophoresis: criteria for bacterial strain typing. J Clin Microbiol. (1995) 33:2233–9. doi: 10.1128/JCM.33.9.2233-2239.1995
32. Albers AL, Mollenkopf DF, Mathys DA, Daniels JB, Wittum TE. Salmonella Enterica Prevalence in the Ohio State University Veterinary Medical Center Environment. (2017) Available online at: https://kb.osu.edu/handle/1811/80678 (accessed February 03, 2018).
33. Jones SL. Inflammatory Diseases of the Gastrointestinal Tract Causing Diarrhea, In Reed S, editors. Equine Internal Medicine, (St. Louis: Saunders), p. 884–888.
34. Wales A, Davies RH. Environmental Aspects of Salmonella, In Barrows PA, Methner U, editors. Salmonella in Domestic Animals. Oxfordshire: CABI. p. 399–425. doi: 10.1079/9781845939021.0399
35. Hartmann FA, West SE. Antimicrobial susceptibility profiles of multidrug-resistant salmonella anatum isolated from horses. J Vet Diagn Invest. (1995) 7:159–61. doi: 10.1177/104063879500700128
36. Dunowska M, Morley PS, Traub-Dargatz JL, Davis MA, Patterson G, Frye JG, et al. comparison of salmonella enterica serotype infantis isolates from a veterinary teaching hospital. J Appl Microbiol. (2007) 102:1527–36. doi: 10.1111/j.1365-2672.2006.03198.x
37. Amavisit P, Markham PF, Lightfoot D, Whithear KG, Browning GF. Molecular epidemiology of Salmonella Heidelberg in an equine hospital. Vet Microbiol. (2001) 80:85–98. doi: 10.1016/S0378-1135(00)00373-4
38. Cummings KJ, Rodriguez-Rivera LD, Mitchell KJ, Hoelzer K, Wiedmann M, McDonough PL, et al. Salmonella enterica serovar oranienburg outbreak in a veterinary medical teaching hospital with evidence of nosocomial and on-farm transmission. Vector Borne Zoonotic Dis. (2014) 14:496–502. doi: 10.1089/vbz.2013.1467
39. Soza-Ossandón P, Toledo V, Moreno-Switt A. Brote de Salmonella spp. en potrillos al pie, en un criadero de caballos fina sangre de carrera (fsc) de la región metropolitana en chile. in XXIII Congreso Latino Americano de Microbiología (Rosario, Argentina), 1160. Available online at: http://www.alam-cam2016.aam.org.ar/descarga/LibroCAMALAM2016.pdf (accessed February 03, 2018).
40. Ekiri AB, Morton AJ, Long MT, MacKay RJ, Hernandez JA. Review of the epidemiology and infection control aspects of nosocomial Salmonella infections in hospitalised horses. Equine Vet Educ. (2010) 22:631–41. doi: 10.1111/j.2042-3292.2010.00144.x
41. Ducel G, Fabry J, Nicolle L. Prevention of Hospital Acquired Infection: A Practical Guide. World Heal Organ. (2002). p. 1–72. Available at: http://www.who.int/csr/resources/publications/whocdscsreph200212.pdf (accessed May 11, 2015).
Keywords: Salmonella enterica, multidrug-resistant, equine hospital, hospital-acquired infections, biosecurity
Citation: Soza-Ossandón P, Rivera D, Tardone R, Riquelme-Neira R, García P, Hamilton-West C, Adell AD, González-Rocha G and Moreno-Switt AI (2020) Widespread Environmental Presence of Multidrug-Resistant Salmonella in an Equine Veterinary Hospital That Received Local and International Horses. Front. Vet. Sci. 7:346. doi: 10.3389/fvets.2020.00346
Received: 24 February 2020; Accepted: 18 May 2020;
Published: 10 July 2020.
Edited by:
Marina Spinu, University of Agricultural Sciences and Veterinary Medicine of Cluj-Napoca, RomaniaReviewed by:
Min Yue, Zhejiang University, ChinaYves Millemann, INRA École Nationale Vétérinaire d'Alfort (ENVA), France
Copyright © 2020 Soza-Ossandón, Rivera, Tardone, Riquelme-Neira, García, Hamilton-West, Adell, González-Rocha and Moreno-Switt. This is an open-access article distributed under the terms of the Creative Commons Attribution License (CC BY). The use, distribution or reproduction in other forums is permitted, provided the original author(s) and the copyright owner(s) are credited and that the original publication in this journal is cited, in accordance with accepted academic practice. No use, distribution or reproduction is permitted which does not comply with these terms.
*Correspondence: Andrea I. Moreno-Switt, andrea.moreno@unab.cl; andrea.moreno@uc.cl